Abstract
The roots of three commonly used taxa of Echinacea.—E. purpurea., E. pallida. var. pallida., and E. pallida. var. angustifolia.—were extracted and fractionated by means of accelerated solvent extraction to reflect the most commonly used methods for commercial preparations. These fractions were analyzed by HPLC for their content of caffeic acid derivatives and alkamides and for antiviral activities against three viruses often implicated in colds and influenza. Aqueous extracts of E. purpurea. root contained a relatively potent activity against herpes simplex virus (HSV) and influenza virus (FV) but not against rhinovirus (RV). These fractions had low amounts of caffeic acids and alkamides. The ethyl acetate fraction contained significant but weak activity against both HSV and FV and contained significant levels of cichoric acid. In contrast, E. pallida. var. angustifolia. gave no water-soluble antiviral activity, but the ethanolic and ethyl acetate fractions contained significant activity against all three viruses, and this activity correlated with the presence of alkamides. E. pallida. var. pallida., however, gave no antiviral activity in any of the fractions, and this observation accorded well with the near absence of the marker compounds. Thus, we have detected a relatively potent water-soluble antiviral activity in E. purpurea. root, together with the weaker antiviral cichoric acid; an antiviral alkamide fraction in E. pallida. var. angustifolia.; but no antiviral activity in E. pallida. var. pallida.. Therefore, different types of Echinacea. root preparations, such as tinctures, tablets, and teas, based on different species and extraction methods, would be expected to offer quite different antiviral profiles.
Introduction
The genus Echinacea. is endemic in the U.S. Great Plains and Canadian prairies of North America, and the nine species originally described by McGregor have now been assigned to four species and eight varieties (Binns et al., Citation2002a). Healers of the indigenous First Nations have traditionally used a number of different species for ethnomedical applications, particularly for coughs and chest conditions, sore throat, and thrush (Shemluck, Citation1982). Modern uses supported in the literature (Bauer, Citation1998; Barrett, Citation2003) are treatment of colds and influenza, lung conditions, wound healing and candidiasis, and various other topical and systemic conditions. There are hundreds of Echinacea. products on the market. Several common types of root preparation are made commercially as tinctures, teas, or whole powders in capsule. These may be made from E. purpurea., E. pallida. var. angustifolia. (syn. E. angustifolia.), or E. pallida. var. pallida. (syn. E. pallida.). The chemical composition and bioactivities of Echinacea. have been reviewed comprehensively by Bauer (Citation1998), and a survey of authentic wild collected North American taxa has recently been reported (Binns et al., Citation2002a). The best characterized constituents are the caffeic acid derivatives and bioactive lipophilic alkamides and polyacetylenes (Bauer, Citation1998). The cell wall–derived extractable polysaccharides and glycoproteins are complex and less well characterized, but they have been shown to possess immune modulatory activities in various test systems in vivo. and in vitro. (Bauer, Citation1998).
In view of the current popularity of Echinacea. preparations in the prevention and treatment of upper respiratory symptoms, it is tempting to speculate that antiviral activities are present, and indeed there have been a few reports of anti-HSV activity (Wacker & Hilbig, Citation1978; Binns et al., Citation2002b). However, there has been no comprehensive analysis of such activities, and furthermore it is not clear whether these are due to direct or indirect effects on specific virus infections; whether the effects are due to specific classes of phytochemicals; or which types of formulation might best present antiviral activity. We therefore decided to study antiviral effects of phytochemically characterized extracts and fractions of different Echinacea. species. This report focuses on root extracts derived from E. purpurea., E. pallida. var. angustifolia., and E. pallida. var. pallida. and their effects on three different human pathogenic viruses associated with epithelial cell infections. In addition, we have found previously that some other members of the Asteraceae family contain potent antiviral photosensitizers (Towers et al., Citation1997; Hudson & Towers, Citation1999). This observation, together with the more recent demonstration of photoactive antifungal components in Echinacea. (Merali et al., Citation2003), prompted us to carry out antiviral assays both in light and dark conditions.
Materials and Methods
Echinacea. sources
Echinacea purpurea., E. pallida.var. angustifolia., and E. pallida. var. pallida. (Heliantheae: Asteraceae) were commercially grown materials sourced from North American growers. They were identified according to a recent taxonomic revision (Binns et al., Citation2002a), and voucher specimens were deposited in the herbarium at the University of Ottawa, Canada. Voucher numbers and codes for extracts are provided in .
Table 1.. Echinacea. samples voucher numbers and codes for extracts.
Extraction methodology
Ethanol extracts (55% and 70%).: Ground dried plant material (10 g) (1 mm Ø screen; Thomas-Wiley mill, Philadelphia, PA, USA) was extracted with an ASE 100 (Accelerated Solvent Extraction, DIONEX) using the following parameters: temperature, 40°C; static time, 5 min; flush volume, 70%; purge time, 100 s, static cycles, 3. These were fractionated according to Binns et al. (Citation2002b) three times with equal parts n.-hexane and distilled water, giving three n.-hexane fractions (pooled into one) and three hydroalcoholic portions (pooled). The latter were fractionated twice with an equal volume of ethyl acetate (pooled), the second of which was adjusted to pH < 2.5 to maximize dissolution of cichoric acid.
Water extracts (40% and 80°C).: The ASE 100 was not used to produce the water extracts because foam blocked the machine. The following method was used: Ground plant material (10 g) was extracted twice with 100 ml of dH2O (40°C or 80°C bath during 1 h) and the extracts were filtered.
All fractions were concentrated at 30°C in a rotary-evaporator (not to dryness) and adjusted to 50 ml in the appropriate solvent prior to HPLC separation. The alkamide dodeca-2E.,4E.,8Z.,10E./Z.-tetraenoic acid isobutylamide with peak identification numbers 8 and 9 as assigned by Bauer et al. (Citation1988) (“Tetraene 8/9”) and caffeic acid derivative were analyzed as described previously (Binns et al., Citation2002b).
Cells and viruses
The cell lines.: Vero, H-1 (a subclone of HeLa cells that is particularly sensitive to rhinovirus replication), and BEAS-2B human bronchial epithelial cell line were obtained from American Type Culture Collection (ATCC). They were propagated in Dulbecco MEM with 5–10% fetal bovine serum, without antibiotics or antifungal agents. In the case of BEAS-2B cells, the medium was a 1:1 mix of DMEM and F-12. Herpes simplex virus (HSV) type 1 and influenza virus (FV) were propagated and assayed in Vero cells and H-1, respectively, according to our published methods (Marles et al., Citation1992; Hudson et al., Citation1998). Rhinovirus (RV) type 14 was obtained from ATCC. It was propagated and assayed in H-1 cells at 34°C.
Antiviral assays
Complete details of our assay procedures have been given in several recent publications (Marles et al., Citation1992; Hudson et al., Citation1998). The cpe (cytopathic effect) end-point method was used, unless indicated otherwise, in 96-well culture trays. In essence, serial twofold dilutions of extracts were made, in duplicate or triplicate in DMEM, across the trays. Infectious virus particles (in the form of 100 plaque-forming units or 100 tissue culture infectious doses) were added to each well, and the trays were exposed to combinations of UV-A and fluorescent lamps for 30 min. These reaction mixtures were then transferred by means of multipipettors onto aspirated cell monolayers in another set of trays. The presence or absence of viral cpe in all wells was recorded when untreated virus-containing wells showed complete cpe, 3–5 days depending on the cell-virus combination. In every experiment, a variety of controls were incorporated in separate wells or trays, including untreated virus-infected wells, wells with no virus (medium or solvent only), and one or more unrelated extracts known to have antiviral activity or no activity, as positive and negative controls. The endpoint was the highest dilution of extract giving complete elimination of viral cpe (i.e., 100% killing of the virus inoculum). In some experiments, virus plaque assays were used, as indicated in the text, to confirm the results of the end-point methods.
In some cases, when tests were conducted to examine the presence of photosensitizers, the light exposure was omitted (dark antiviral activity) in half the test trays by wrapping them in aluminum foil.
Results
Fractionated E. purpurea. roots
Portions of the dried roots were individually fractionated as indicated in the scheme shown in . These were designed to reflect a few of the different modes of traditional consumption of E. purpurea.. Thus, the 55% ethanol fractions (SG 1) represent root tinctures, water extraction at 40°C (SG 2) represents oral consumption (i.e., as tablets or capsules), and the 80°C water fractions (SG 3) represent teas. The 70% ethanol fraction (SGEPR) was subfractionated to give different phytochemical fractions of varying lipophilicity: hexane (SG 4), ethyl acetate (SG 5), and aqueous (SG 6), as shown.
Figure 1 Fractionation scheme for Echinacea purpurea. root. Numbers in boxes represent cpe end-point dilutions in antiviral assays. HSV, herpes simplex virus; FV, influenza virus. There were no anti-rhinovirus activities in these fractions.
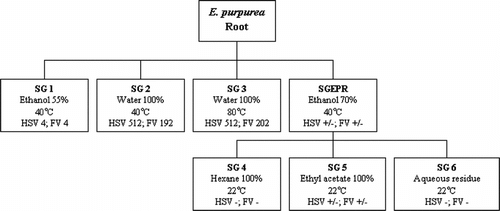
Phytochemical profiling by HPLC () of the fractions revealed the standard profile (Binns et al., Citation2002c) of previously determined E. purpurea. compounds in the alcohol extracts (SG 1 and SGEPR). These are large amounts of cichoric acid and absence or traces of echinacoside or cynarin. There were small amounts of caftaric and chlorogenic acids. The tetraene 8/9 content was low in these samples. The water extract at 40°C had only trace amount of one compound, caffeic acid, and at 80°C small amounts of CADs (caffeic acid derivatives) were extracted, but no alkamides. The solvent series (SG 4, 5, and 6) gave significant amounts of CAD only in the ethyl acetate extract. We hypothesized that it would be possible to correlate these phytochemical markers with antiviral activity.
Table 2.. Phytochemical profiling by HPLC of Echinacea. root extracts.
Three important human viruses were used to evaluate antiviral activities: HSV commonly causes skin and mucous membrane lesions, including “cold sores”; RV and FV are the most frequent causes of “colds” and “flu.” shows the end-point dilutions of each fraction giving complete inactivation of the test virus; the higher the number the greater the dilution and hence the more potent the fraction. Results are shown for one representative experiment.
In general, the antiviral profiles were similar for HSV and FV, both of which contain membranes, potential targets of the antiviral compounds. The numbers for the two viruses were not necessarily identical, however, and, in fact, were not expected to be, because for practical reasons the viruses cannot be assayed simultaneously and cannot be assayed in the same cell type.
The data shown in clearly indicate that the antiviral activity was located predominantly in the aqueous fractions (SG 2 and 3). Because each fraction was equivalent in terms of dry weight of starting plant material, then these values are relative to each other and therefore reflect relative potency in the original plant.
These findings were reinforced by the data in , in which the antiviral activities are expressed as MIC100 (minimum concentration required to inactivate 100% virus), based on the actual concentrations (µg/ml) of each fraction. Again, the most potent fractions (i.e. those with the lowest MICs) were SG 2 and 3.
Table 3.. Antiviral activities of Echinacea purpurea. root fractions. The concentrations of cichoric acid are presented along with the MIC100(µg/ml) against herpes simplex virus (HSV) and influenza virus (FV).
The water-soluble activity, however, was not associated with the standard Echinacea. markers in , nor the concentration of cichoric acid, which we have previously reported to possess moderate anti-HSV activity (Binns et al., Citation2002b). Possibly, the cell wall extractable polysaccharides or glycoproteins contributed to the potent antiviral activity.
The active fractions were also effective against HSV in two other human cell lines, the BEAS-2B respiratory line, and the H-1 subclone of HeLa cells. In addition, these results were confirmed in selected cases by plaque assays for HSV (data not shown). None of the fractions, however, were active against rhinovirus.
Fractionated E. pallida. var. angustifolia. and E. pallida. var. pallida. roots
E. pallida. var. angustifolia. and E. pallida. var. pallida. roots were fractionated in the same way as E. purpurea. and subjected to the same phytochemical evaluation and antiviral tests. E. pallida. var. angustifolia. (SG 7a and SGEARa) had the expected marker phytochemicals in the alcohol extracts (). There were large amounts of echinacoside and tetraene 8/9 as well as significant amounts of the cynarin. Again, the aqueous extracts were low in all CAD and alkamides. In the solvent series, the hexane extract and ethyl acetate had large amounts of alkamides, and the ethyl acetate extract had most of the CADs.
In the antiviral testing, however, the results were remarkably different from E. purpurea.. None of the fractions were active against HSV or FV, but E. pallida. var. angustifolia. displayed significant anti-rhinovirus activity. The latter is expressed as MIC100 in . This activity was confined to the ethanol extracts, and particularly in the hexane/ethyl acetate subfractions. In contrast to the situation with E. purpurea., these antiviral activities correlated well with the presence of alkamides in the extracts.
Table 4.. Antiviral activities of Echinacea pallida. var. angustifolia. and var. pallida. root fractions. SG 7b and SG 9b were not obtained in sufficient quantities to analyze. The concentrations of tetraene 8/9 are presented along with the MIC100(µg/ml) against rhinovirus (RV), herpes simplex virus (HSV) and influenza virus (FV).
Another E. pallida. var. angustifolia. accession from a different location (root b) was fractionated in the same way, and although in this case the ethyl acetate fraction contained relatively little alkamide, this correlated with the correspondingly lower level of anti-RV activity, in contrast with the alkamide-rich hexane fraction, which had potent anti-RV activity. Thus, the anti-RV activities were probably due to the alkamide constituents.
The E. pallida. var. pallida. alcohol extracts showed characteristic phytochemistry (): low alkamides, presence of several CADs including echinacoside. Ketoalkene/ynes were also observed in the chromatogram but not quantified. In this root, no antiviral activities at all were detected; thus no correlation with phytochemistry was possible.
Tests for antiviral photosensitizers
Because we have often found that herbal antivirals are photosensitizers (Towers et al., Citation1997; Hudson & Towers, Citation1999), we decided to carry out assays on the E. purpurea. fractions in the presence and absence of light (both visible and UV-A) for HSV and FV. It was not practical to do this for the RV activities, which were close to the upper limit of concentrations that could be used. As positive and negative controls, we routinely included antiviral extracts with and without known photodependency. The results are shown in as toxicity ratios; that is, toxicity in the presence of light (1/MIC100(Light)) divided by toxicity in the absence of light (1/MIC100(Dark)). Green tea is an example of an extract that has no dependency on light; therefore, the MICs in presence or absence of light were essentially the same (ratio approximately 1.0), whereas red clover extract has no antiviral activity against HSV or FV in the dark but is active in the presence of light (ratio >> 1). The known antiviral photosensitizer hypericin had a ratio >100. In contrast, the antiviral E. purpurea. fractions had ratios between 1 and 4, suggestive of possible slight photoenhancement but not photodependency.
Table 5.. Antiviral photosensitizer analysis. The toxicity ratios of MIC100(light)/MIC100(dark) (µg/ml) against herpes simplex virus (HSV) and influenza virus (FV) are presented.
Cytotoxic activities
Among all the Echinacea. fractions tested, only SG 1–4 and SGEPR showed any cytotoxic effects during the assays (observed microscopically), and then only at the highest concentrations tested. These did not interfere with the antiviral assays.
Discussion
We have shown in this study that E. purpurea. roots contain at least one potent water-souble antiviral compound, which is active against the two membrane-containing viruses herpes simplex type 1 and influenza virus. In contrast, this compound was not active against the common cold-producing rhinovirus. Because RV does not contain a membrane, this suggests that a membrane component of HSV and FV might be the target of the antiviral compound.
This antiviral activity was not associated with any of the known caffeic acid constituents or lipophilic compounds of E. purpurea., including those often advocated as chemical markers, such as cichoric acid and echinacoside (Bauer, Citation1998; Binns et al., Citation2002c). In fact, the most active fractions, SG 2 and SG 3, were largely devoid of such compounds. However, because the water-soluble fractions were expected to contain several types of bioactive polysaccharides and glycoproteins, these could be the source of the antiviral activity, although many other types of compound are also present in these fractions. Nevertheless, the fact that the other species of Echinacea., which presumably also contained substantial glycoproteins and polysaccharides but had no such antiviral activity, suggests that the active compounds in E. purpurea. were not in these classes.
The ethanol-soluble fraction of E. purpurea., and the ethyl acetate subfraction derived from it, did however possess marginal antiviral activity against HSV and FV, and this activity corresponded well with high concentrations of cichoric acid, which we have previously reported to have moderate antiviral activity (Binns et al., Citation2002b).
We tested active fractions for the presence of antiviral photosensitizers, by comparing their MICs in the presence and absence of light. We normally conduct antiviral assays with defined exposure to both UV-A and fluorescent light, in accordance with our previous observations that demonstrated the frequent presence of UV-A–dependent and visible light–dependent compounds in herbal extracts (Hudson & Towers, Citation1999). However, none of them showed more than a slight enhancement of activity in light, suggesting the absence of antiviral photosensitizers.
The results of the analogous studies on fractions of E. pallida. were completely different from E. purpurea.. The water-soluble fractions did not show antiviral activity, but the ethanol and ethyl acetate fractions of E. pallida. var. angustifolia. were active to some degree against all three viruses. These activities correlated very well with the presence of alkamides, but not with echinacoside, the principal phenolic constituent.
In the case of E. pallida. var. pallida., we were unable to detect antiviral activity in any of the fractions. None of these contained alkamides, although various phenolic compounds were present in small amounts.
In summary, we conclude that E. purpurea. root contains a potent water-soluble antiviral ingredient that can inactivate HSV and FV but not RV and that is not one of the major phenolic constituents. In contrast, E. pallida. var. angustifolia. root contains a significant anti-rhinovirus activity, probably due to one or more alkamides, which would be anticipated in ethanol soluble preparations. E. pallida. var. pallida., however, appears to be devoid of all these compounds and consequently devoid of antiviral activity.
Based on these results, it is clear that different commercial preparations of Echinacea. roots can be expected to differ widely in their profiles of antiviral activities.
References
- Barrett B (2003): Medicinal properties of Echinacea.: A critical review. Phytomedicine 2 10: 66–86.
- Bauer R (1998): Echinacea.: Biological effects and active principals. Phytomedicines of Europe: Chemistry and biological activity. In: Lawson LD, Bauer R, eds., ACS Symposium Series 691. Washington, DC, American Chemical Society, pp. 140–157.
- Bauer R, Khan IA, Wagner H (1988): TLC and HPLC analysis of Echinacea pallida. and E. angustifolia. roots. Planta Med 54: 426. [CSA]
- Binns S, Baum BR, Arnason JT (2002a): A taxonomic revision of the genus Echinacea. (Heliantheae: Asteraceae). Syst Bot 27: 610–632. [CSA]
- Binns SE, Hudson J, Merali S, Arnason JT (2002b): Antiviral activity of characterized extracts from Echinacea. spp. (Heliantheae: Asteraceae) against Herpes simplex virus (HSV-1). Planta Med 68: 780–783. [PUBMED], [INFOTRIEVE], [CSA], [CROSSREF]
- Binns SE, Livesey JF, Arnason JT, Baum BR (2002c): Phytochemical variation in Echinacea. from roots and flowerheads of wild and cultivated populations. J Agric Food Chem 50: 3673–3687. [PUBMED], [INFOTRIEVE], [CSA], [CROSSREF]
- Hudson JB, Kim JH, Lee MK, DeWreede RE, Hong YK (1998): Anti-viral compounds in extracts of Korean seaweeds: Evidence for multiple activities. J Appl Phycol 10: 427–434. [CSA], [CROSSREF]
- Hudson JB, Towers GHN (1999): Phytomedicines as antivirals. Drugs of the Future 24: 295–320. [CSA], [CROSSREF]
- Marles RJ, Hudson JB, Graham EA, Soucy-Breau C, Morand P, Compadre RL, et al. (1992): Structure-activity studies of photoactivated anti-viral and cytotoxic tricyclic thiophenes. Photochem Photobiol 56: 479–487. [PUBMED], [INFOTRIEVE], [CSA]
- Merali S, Binns S, Paulin-Levasseur M, Ficker C, Smith M, Baum BR, et al. (2003): Antifungal and anti-inflammatory activity of the genus Echinacea.. Pharm Biol 41: 412–420. [CSA]
- Shemluck M (1982): Medicinal and other uses of the Compositae by Indians in the United States and Canada. J Ethnopharmacol 5: 303–358. [PUBMED], [INFOTRIEVE], [CSA], [CROSSREF]
- Towers GHN, Page JE, Hudson JB (1997): Light-mediated biological activities of natural products from plants and fungi. Curr Org Chem 1: 395–414. [CSA]
- Wacker A, Hilbig A (1978): Virus inhibition by Echinacea purpurea.. Planta Med 33: 89–102. [PUBMED], [INFOTRIEVE], [CSA]