Abstract
Cardiospermum halicacabum. Linn. (Sapindaceae) is a climber, commonly found as a weed throughout India, used as vegetable as well as traditional medicine for rheumatism, lumbago, and nervous diseases and as demulcent in orchitis and in dropsy. In the current study, we investigated the antioxidant potency of methanol extract of C. halicacabum. (CHE) employing various established in vitro. systems, such as the β.-carotene-linoleate model system, 1,1-diphenyl-2-picrylhydrazyl (DPPH)/superoxide/nitric oxide radical scavenging, reducing power, and iron ion chelating activity. CHE showed noticeable inhibitory activity toward β.-carotene–linoleate model system. Furthermore, CHE exhibited a moderate concentration-dependent inhibition of DPPH radical. The multiple antioxidant activity of CHE was evident as it also possessed reducing power, superoxide scavenging ability, nitric oxide scavenging activity, and also ferrous ion chelating potency. The data obtained in the in vitro. models clearly establish the antioxidant potency of methanol extract of C. halicacabum..
Introduction
Reactive oxygen species (ROS) have been implicated in the development of chronic diseases, such as cancer, arteriosclerosis, rheumatism, and other age-related problems (Halliwell, Citation1996). An increasing amount of experimental and clinical evidence shows that a balanced intake of antioxidant with scavenging action of ROS is important for the prevention of diseases (Bland, Citation1995; Gerber et al., Citation2002; Kris-Etherton et al., Citation2002). Plants have played an important role throughout human history in treating diseases and now serve as a lead in the development of new drugs. Several plant extracts and different classes of phytochemicals have been shown to have antioxidant activity (Oomah & Mazza, Citation1994; Cao et al., Citation1996; Wang et al., Citation1996; Bergman et al., Citation2001). Thus, there has been an increasing demand to evaluate the antioxidant properties of direct plant extracts or isolated products from plant origin rather than looking for synthetic ones (McClements & Decker, Citation2000). Epidemiological studies show that many phytonutrients of fruits and vegetables may be beneficial in protecting the human body against damage by reactive oxygen and nitrogen species (Diplock et al., Citation1998; Halliwell, Citation1997). In recent years, one of the areas that have attracted a great deal of attention is antioxidants in the control of degenerative diseases in which oxidative damage has been implicated.
Cardiospermum halicacabum. Linn. (Sapindaceae) is an annual or sometimes perennial climber, commonly found as a weed throughout India. The tender, young shoots are used as a vegetable, fodder, diuretic, stomachic, and rubefacient. It is used in rheumatism, lumbago, nervous diseases, and as a demulcent in orchitis and in dropsy. In Sri Lanka, it is used for the treatment of skeletal fractures. The juice of the herb is used to cure ear ache and to reduce hardened tumors. It exhibits significant analgesic, anti-inflammatory activity and vasodepressant activity, which is transient in nature. In vitro. studies have revealed its antispasmodic and curate-like actions confirming the use of the herb in Ayurvedic medicine (Anonymous, Citation1992). The leaves of this plant mixed with castor oil are administered internally to treat rheumatism and to check lumbago (Kirthikar et al., Citation1969). Two glasses of a 12 h maceration of aerial parts of the plant are consumed or used for bathing in the treatment of hyperthermia, and in some areas water extracts of the seed are used (Neuwinger, Citation2000). Most traditional uses of this plant have not been investigated scientifically, and thus there is no scientific proof of efficacy.
As far as our literature survey could ascertain, antioxidant activities of this plant have not previously been published. Hence, the current work investigates the possible antioxidative effects of the methanol extract of C. halicacabum. (CHE). In this study, we examined the antioxidant activity of CHE, employing various in vitro. assay systems, such as the β.-carotene–linoleate model system, DPPH/superoxide/nitric oxide radical scavenging, reducing power, and iron ion chelation, in order to understand the usefulness of this plant as a foodstuff as well as a medicine.
Materials and Methods
Chemicals
Linoleic acid, β.-carotene, ferrozine, and 1,1-diphenyl-2-picrylhydrazyl (DPPH) were purchased from Sigma-Aldrich (Steinheim, Germany). Butylated hydroxytoluene (BHT), ascorbic acid (AA), sulfanilamide, N.-(1-naphthyl) ethylenediamine dihydrochloride, and ethylenediaminetetraacetic acid (EDTA) were purchased from Merck (Mumbai, India). Potassium ferricyanide, nitro blue tetrazolium (NBT), trichloroacetic acid (TCA), and ferric chloride were purchased from SD Fine Chemicals (Mumbai, India). All other reagents were of analytical grade.
Plant material and extract preparation
Fresh leaves were collected from Chennai, India, during the month of October 2003. Botanical identification was performed by Dr. D. Narashiman, Department of Botany, Madras Christian College, where the voucher specimen was deposited. The collected leaves were cleaned, dried under shade at room temperature, and powdered. The powder (25 g) was defatted with n.-hexane. The defatted material was then extracted repeatedly with methanol at room temperature until the extraction solvent became colorless. The obtained extracts were filtered over Whatman no. 1 filter paper and the filtrate was collected, then methanol was removed by a rotary evaporator at 50°C to yield 2.91 g of dried methanol extract.
Antioxidant assay using a β.-carotene–linoleate model system
The antioxidant activity of extract was evaluated by the β.-carotene–linoleate model system (Miller, Citation1971). A solution of β-carotene was prepared by dissolving 2 mg of β-carotene in 10 ml of chloroform. This solution (2 ml) was pipetted into a 100 ml round-bottom flask. After chloroform was removed under vacuum, 40 mg of purified linoleic acid, 400 mg of Tween 40 emulsifier, and 100 ml of aerated distilled water were added to the flask with vigorous shaking. Aliquots (4.8 ml) of this emulsion were transferred into test tubes containing different concentrations of the extract (0.2 ml). As soon as the emulsion was added to each tube, the zero time absorbance was measured at 470 nm using a spectrophotometer. The tubes were placed at 50°C in a water bath, and measurement of absorbance was recorded after 2 h; a blank, devoid of β-carotene, was prepared for background subtraction. The same procedure was repeated with the synthetic antioxidant, butylated hydroxytoluene (BHT), as a positive control. Antioxidant activity was calculated using the following equation:
DPPH radical scavenging activity
The antioxidant activity of the extracts, based on the scavenging activity of the stable 1,1-diphenyl-2- picrylhydrazyl (DPPH) free radical, was determined by the method described by Braca et al. (Citation2001). Plant extract (0.1 ml) was added to 3 ml of a 0.004% MeOH solution of DPPH. Methanol (0.1 ml), in the place of plant extract, was used as control. Absorbance at 517 nm was determined after 30 min, and the percent inhibition activity was calculated as [(A0 − A1)/A0] × 100, where A0 is the absorbance of the control, and A1 is the absorbance of the extract/standard.
Assay of superoxide radical (O2⋅−) scavenging activity
The assay was based on the capacity of the methanol extract to inhibit formazan formation by scavenging the superoxide radicals generated in riboflavin–light–NBT system (Beauchamp & Fridovich, Citation1971). The method used by Martinez et al. (Citation2001) for determination of superoxide dismutase was followed after modification. Each 3 ml reaction mixture contained 50 mM sodium phosphate buffer, pH 7.8, 13 mM methionine, 2 µM riboflavin, 100 µM EDTA, NBT (75 µM), and 1 ml sample solution. The production of blue formazan was followed by monitoring the increase in absorbance at 560 nm after a 10-min illumination from a fluorescent lamp. The entire reaction assembly was enclosed in a box lined with aluminum foil. Identical tubes with reaction mixture were kept in the dark and served as blanks. The percentage inhibition of superoxide anion generation was calculated using the following formula:
where A0 is the absorbance of the control, and A1 is the absorbance of the methanol extract/standard.
Assay of nitric oxide scavenging activity
The assay procedure is based on the principle that sodium nitroprusside, in aqueous solution at physiological pH, spontaneously generates nitric oxide, which interacts with oxygen to produce nitrite ions that can be estimated using Griess reagent. Scavengers of nitric oxide compete with oxygen leading to reduced production of nitrite ions. For the experiment, sodium nitroprusside (10 mM) in phosphate-buffered saline was mixed with different concentrations of CHE dissolved in methanol and incubated at room temperature for 150 min. The same reaction mixture without CHE but an equivalent amount of methanol served as control. After the incubation period, 0.5 ml of Griess reagent [1% sulfanilamide, 2% H3PO4, and 0.1% N.-(1-naphthyl) ethylenediamine dihydrochloride] was added. The absorbance of the chromophore formed was read at 546 nm. Curcumin was used as a positive control (Sreejayan & Rao, Citation1997).
Reducing power determination
The reducing power of CHE was determined according to the method of Oyaizu (Citation1986). Different concentrations of extract (25–200 µg/ml in methanol) were mixed with phosphate buffer (2.5 ml, 0.2 mol/L, pH 6.6) and potassium ferricyanide [K3Fe(CN)6] (2.5 ml, 1%). The mixture was incubated at 50°C for 20 min. A portion (2.5 ml) of trichloroacetic acid (10%) was added to the mixture, which was then centrifuged for 10 min. The supernatant (2.5 ml) was mixed with distilled water (2.5 ml) and FeCl3 (0.5 ml, 0.1%), and the absorbance was measured at 700 nm. Increased absorbance of the reaction mixture indicated increased reducing power.
Metal chelating activity
The chelating of ferrous ions by CHE was estimated by the method of Dinis et al. (Citation1994). Briefly, the extract (50–500 µg/ml) was added to a solution of 2 mM FeCl2 (0.05 ml). The reaction was initiated by the addition of 5 mM ferrozine (0.2 ml); the mixture was shaken vigorously and allowed to stand at room temperature for 10 min. Absorbance of the solution was then measured spectrophotometrically at 562 nm. The percentage of inhibition of ferrozine–Fe2+ complex formation was calculated as [(A0 − A1)/A0] × 100, where A0 is the absorbance of the control, and A1 is the absorbance of the extract/standard.
Statistical analysis
Experimental results were reported as mean ± SD of three parallel measurements. The data were analyzed by an analysis of variance (p < 0.05) and the means separated by Duncan's multiple range test. The EC50 values were calculated from regression analysis. Results were processed by computer programs: Excel and Statistica software.
Results and Discussion
shows the antioxidant activity of the extract as measured by the bleaching of β.-carotene. The addition of CHE and BHT at various concentrations prevented the bleaching of β.-carotene to different degrees. β.-Carotene in this model system undergoes rapid discoloration in the absence of an antioxidant. This is because of the coupled oxidation of β-carotene and linoleic acid, which generates free radicals. The linoleic acid free radical formed upon the abstraction of a hydrogen atom from one of its diallylic methylene groups attacks the highly unsaturated β.-carotene molecules. As a result, β-carotene will be oxidized and broken down in part; subsequently, the system loses its chromophore and characteristic orange color, which can be monitored spectrophotometrically. The presence of different antioxidants can hinder the extent of β.-carotene bleaching by neutralizing the linoleate-free radical and other free radicals formed in the system (Jayaprakasha et al., Citation2001). In our current study, CHE was found to hinder the extent of β.-carotene bleaching by neutralizing the linoleate free radical and other free radicals formed in the system. In comparison, CHE showed an appreciable antioxidant activity of 70.62% at 200 µg/ml, with BHT having 89.64% antioxidant activity at 100 µg/ml.
Table 1 Antioxidant activity of methanol extract of C. halicacabum. in β.-carotene–linoleate system
CHE showed a concentration-dependent antiradical activity by inhibiting DPPH radical with an EC50 value of 67.55 µg/ml (). EC50 value is the effective concentration at which the antioxidant activity was 50%. DPPH is usually used as a substrate to evaluate antioxidative activity of antioxidants (Oyaizu, Citation1986). The method is based on the reduction of methanolic DPPH solution in the presence of a hydrogen donating antioxidant due to the formation of the nonradical form DPPH-H by the reaction. The extract was able to reduce the stable radical DPPH to the yellow-colored diphenylpicrylhydrazine. It has been found that cysteine, glutathione, ascorbic acid, tocopherol, polyhydroxy aromatic compounds (hydroquinone, pyrogallol, gallic acid, etc.), and aromatic amines (p.-phenylene diamine, p.-aminophenol, etc.) reduce and decolorize 1,1-diphenyl-2-picrylhydrazyl by their hydrogen-donating ability (Blois, Citation1958). It appears that CHE possesses hydrogen-donating abilities to act as an antioxidant. The scavenging effect increased with increasing concentration of the extract. However, scavenging activity of gallic acid, a known antioxidant used as a positive control, was relatively more pronounced than that of CHE.
Table 2 Antiradical activity of methanol extract of C. halicacabum. observed with DPPH
shows the superoxide radical (O2.−) scavenging activity of the extract as measured by the in vitro. riboflavin–NBT–light system. Superoxide radical is known to be very harmful to cellular components as a precursor of more reactive oxygen species (Halliwell & Gutteridge, Citation1989). Photochemical reduction of flavins generates O2.−, which reduces NBT, resulting in the formation of blue formazan (Beauchamp & Fridovich, Citation1971). The extract was found to be a moderate scavenger of superoxide radical generated in the in vitro. riboflavin–NBT–light system. The extract inhibited the formation of the blue formazan, and the percent inhibition was proportional to the concentration with an EC50 value of 145.51 µg/ml. These results indicated that the tested extract had a noticeable effect on scavenging superoxide when compared with ascorbic acid, which was used as a positive control.
Table 3 Superoxide anion scavenging activity of methanol extract of C. halicacabum. observed with riboflavin–light–NBT system
The extract also showed a moderate nitric oxide scavenging activity between 50 and 250 µg/ml in a dose-dependent manner (EC50 = 227.33 µg/ml) (). In addition to reactive oxygen species, nitric oxide is also implicated in inflammation, cancer, and other pathological conditions (Moncada et al., Citation1991). The plant/plant products may have the property to counteract the effect of NO formation and in turn may be of considerable interest in preventing the ill effects of excessive NO generation in the human body. Further, the scavenging activity may also help to arrest the chain of reactions initiated by excess generation of NO that is detrimental to human health. The extract showed a moderate nitric oxide scavenging activity. The percent inhibition was increased with an increasing concentration of the extract. Curcumin, the natural antioxidant, was used as a positive control for comparison (Sreejayan & Rao, Citation1997).
Table 4 In vitro. NO scavenging activity of methanol extract of C. halicacabum.
illustrates the reductive capabilities of sample extract compared with BHT. For the measurements of the reductive ability, we investigated the Fe3+–Fe2+ transformation in the presence of CHE using the method of Oyaizu (Citation1986). Earlier authors (Tanaka et al., Citation1988; Duh, Citation1998; Duh et al., Citation1999a) have observed a direct correlation between antioxidant activity and reducing power of certain plant extracts. The reducing properties are generally associated with the presence of reductones (Duh, Citation1998), which have been shown to exert antioxidant action by breaking the free-radical chain through the donation of a hydrogen atom (Gordon, Citation1990). Reductones have also been reported to react with certain precursors of peroxide, thus preventing peroxide formation. Our data on the reducing power of CHE suggests that it is likely to contribute significantly toward the observed antioxidant effect. However, the antioxidant activity of antioxidants has been attributed to various mechanisms, among which are prevention of chain initiation, binding of transition metal ion catalysts, decomposition of peroxides, prevention of continued hydrogen abstraction, reductive capacity, and radical scavenging (Diplock, Citation1997). Like the antioxidant activity, the reducing power of CHE increased with increasing amount of sample. However, the reducing power of AA was relatively more pronounced than that of CHE.
Figure 1 Reducing power of CHE and AA (25–200 µg/ml); CHE, methanol extract of C. halicacabum.; AA, ascorbic acid.
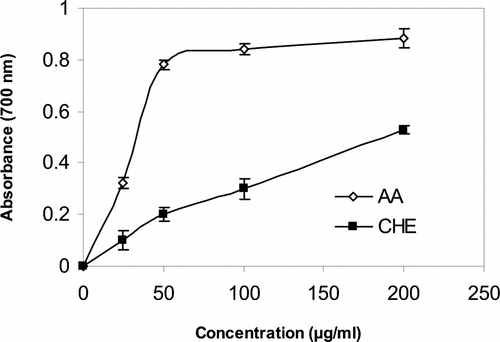
The chelating of ferrous ions by CHE was estimated by the method of Dinis et al. (Citation1994). Ferrozine can quantitatively form complexes with Fe2+. In the presence of other chelating agents, the complex formation is disrupted with the result being that the red color of the complexes decreases. Measurement of the rate of color reduction therefore allows estimation of the chelating activity of the coexisting chelator (Yamaguchi et al., Citation2000). In this assay, both CHE and EDTA interfered with the formation of the ferrous and ferrozine complex, suggesting chelating activity and capture of ferrous ion before ferrozine. The absorbance of Fe2+–ferrozine complex was decreased dose-dependently, otherwise the activity increased with increasing concentration from 50 to 500 µg/ml. Metal chelating capacity was significant because they reduced the concentration of the catalyzing transition metal in lipid peroxidation (Duh et al., Citation1999b). It was reported that chelating agents, which form σ-bonds with a metal, are effective as secondary antioxidants because they reduce the redox potential thereby stabilizing the oxidized form of the metal ion (Gordon, Citation1990). The data shown in reveal that CHE also possesses the capacity for iron binding, suggesting that its action as antioxidant may be related to its iron-binding capacity.
Figure 2 Chelating effect of CHE and EDTA (50–500 µg/ml) on Fe2+ ion. CHE, methanol extract of C. halicacabum.; EDTA, ethylenediaminetetraacetic acid.
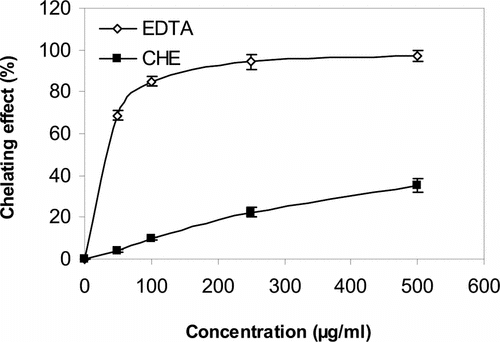
In conclusion, our studies reveal that the methanol extract of C. halicacabum. leaves exhibited different levels of antioxidant activity in all the models studied. The antioxidant activity may correlate with the ethnopharmacological use of this plant for the treatment of inflammatory conditions such as rheumatism, lumbago, and orchitis that involves oxidative stress and may be one of the mechanisms by which this drug is useful as foodstuff as well as traditional medicine. However, further investigation of individual compounds, their in vivo. antioxidant activity, and different antioxidant mechanisms is warranted.
Acknowledgment
The authors are thankful to the CSIR, New Delhi, for financial assistance.
References
- Anonymous (1992): The Wealth of India—A Dictionary of Indian Raw Materials & Industrial Products, Raw Materials Vol. 3. New Delhi, CSIR, pp. 269–271.
- Beauchamp C, Fridovich I (1971): Superoxide dismutase: Improved assays and an assay applicable to acrylamide gels. Anal Biochem 44: 276–277, [PUBMED], [INFOTRIEVE], [CSA]
- Bergman M, Varshavsky L, Gottlieb HE, Grossman S (2001): The antioxidant activity of aqueous spinach extract: Chemical identification of active fractions. Phytochemistry 58: 143–152, [PUBMED], [INFOTRIEVE], [CROSSREF], [CSA]
- Bland JS (1995): Oxidants and antioxidants in clinical medicine: Past, present, and future. J Nutr Environ Med 5: 255–280, [CSA]
- Blois MS (1958): Antioxidants determination by the use of a stable free radical. Nature 4617: 1199–1200, [CSA]
- Braca A, Tommasi ND, Bari LD, Pizza C, Politi M, Morelli I (2001): Antioxidant principles from Bauhinia terapotensis.. J Nat Prod 64: 892–895, [PUBMED], [INFOTRIEVE], [CROSSREF], [CSA]
- Cao GH, Sofic E, Prior RL (1996): Antioxidant capacity of tea and vegetables. J Agric Food Chem 44: 3426–3431, [CROSSREF], [CSA]
- Dinis TCP, Madeira VMC, Almeida LM (1994): Action of phenolic derivates (acetoaminophen, salycilate and 5-aminosalycilate) as inhibitors of membrane lipid peroxidation and as peroxyl radical scavengers. Arch Biochem Biophys 315: 161–169, [PUBMED], [INFOTRIEVE], [CROSSREF], [CSA]
- Diplock AT (1997): Will the good fairies please prove to us that vitamin E lessens human degenerative disease? Free Radical Res 27: 511–532, [CSA]
- Diplock A, Charleux J, Grozier-Willi G, Kok K, Rice-Evans C, Roberfroid M, Stahl W, Vina-Ribes J (1998): Functional food sciences and defence against reactive oxidative species. Br J Nutr 80: 77–82, [CROSSREF], [CSA]
- Duh, PD (1998): Antioxidant activity of Burdock (Arctium lappa. Linne): Its scavenging effect on free-radical and active oxygen. J Am Oil Chem Soc 75: 455–461, [CSA]
- Duh PD, Du PC, Yen GC (1999a): Action of methanolic extract of mung hulls as inhibitors of lipid peroxidation and non-lipid oxidative damage. Food Chem Toxicol 37: 1055–1061, [PUBMED], [INFOTRIEVE], [CROSSREF], [CSA]
- Duh PD, Tu YY, Yen GC (1999b): Antioxidant activity of water extract of harng Jyur (Chrysanthemum morifolium. Ramat). Lebensm Wiss Technol 32: 269–277, [CROSSREF], [CSA]
- Gerber M, Boutron-Ruault MC, Hercberg S, Riboli E, Scalbert A, Siess MH (2002): Food and cancer: State of the art about the protective effect of fruits and vegetables. Bull Cancer 89: 293–312, [PUBMED], [INFOTRIEVE], [CSA]
- Gordon MH (1990): The mechanism of antioxidant action in vitro.. In: Hudson BJF, ed., Food Antioxidants. London, Elsevier Applied Science, pp. 1–18.
- Halliwell B (1996): Oxidative stress, nutrition and health. Experimental strategies for optimization of nutritional antioxidant intake in humans. Free Radical Res 25: 57–74, [CSA]
- Halliwell B (1997): Antioxidants and human disease: A general introduction. Nutr Rev 55: 44–52, [CSA]
- Halliwell B, Gutteridge JMC (1989): Free Radicals in Biology and Medicine, 2nd ed. Oxford, Clarendon Press, pp. 30–55.
- Jayaprakasha GK, Singh RP, Sakariah KK (2001): Antioxidant activity of grape seed (Vitis vinifera.) extracts on peroxidation models in vitro. Food Chem 73: 285–290, [CROSSREF], [CSA]
- Kirthikar KR, Basu BD, An ICS (1969): Indian Medicinal Plants, Vol. 1, 2nd ed. New Delhi, Periodical Experts, p. 623.
- Kris-Etherton PM, Hecker KD, Bonanome A, Coval SM, Binkoski AE, Hilpert KF, Griel AE, Etherton TD (2002): Bioactive compounds in foods: Their role in the prevention of cardiovascular disease and cancer. Am J Med 113 (Suppl 9B): 71S–88S, [PUBMED], [INFOTRIEVE], [CROSSREF], [CSA]
- Martinez AC, Marcelo EL, Marco AO, Moacyr M (2001): Differential responses of superoxide dismutase in freezing resistant Solanum curtibolum. and freezing sensitive Solanum tuberosum. subjected to oxidative and water stress. Plant Sci 160: 505–515, [PUBMED], [INFOTRIEVE], [CROSSREF], [CSA]
- McClements J, Decker EA (2000): Lipid oxidation in oil–water emulsions: Impact of molecular environment on chemical reactions in heterogeneous food system. J Food Sci 65: 1270–1282, [CSA]
- Miller HE (1971): A simplified method for the evaluation of antioxidant. J Am Oil Chem Soc 18: 439–452, [CSA]
- Moncada A, Palmer RMJ, Higgs EA (1991): Nitric oxide: Physiology, pathophysiology and pharmacology. Pharmacol Rev 43: 109–142, [PUBMED], [INFOTRIEVE], [CSA]
- Neuwinger HD (2000): African Traditional Medicine: A Dictionary of Plant Use and Applications. Stuttgart, Germany, Medpharm GmbH Scientific Publishers, pp. 1–300.
- Oomah BD, Mazza G (1994): Flavanoids and antioxidant activities in buckwheat. J Agric Food Chem 7: 1746–1750, [CSA]
- Oyaizu M (1986): Studies on product of browning reaction prepared from glucose amine. Jpn J Nutr 44: 307–315, [CSA]
- Sreejayan N, Rao MNA (1997): Nitric oxide scavenging by curcuminoids. J Pharm Pharmacol 49: 105–107, [PUBMED], [INFOTRIEVE], [CSA]
- Tanaka M, Kuie CW, Nagashima Y, Taguchi T (1988): Applications of antioxidative Maillard reaction products from histidine and glucose to sardine products. Nippon Suisan Gakkaishi 54: 1409–1414, [CSA]
- Wang H, Cao GH, Prior RL (1996): Total antioxidant capacity of fruits. J Agric Food Chem 44: 701–705, [CROSSREF], [CSA]
- Yamaguchi F, Ariga T. Yoshimira Y, Nakazawa H (2000): Antioxidant and antiglycation of carcinol from Garcinia indica. fruit rind. J Agric Food Chem 48: 180–185, [PUBMED], [INFOTRIEVE], [CROSSREF], [CSA]