Abstract
Different culture types (shoot culture, callus culture, and cell suspension culture) of Rosmarinus officinalis. L. were established and their ability to biosynthesize the phytochemicals carnosic acid, carnosol, and rosmarinic acid were assessed and compared with subcultures of the same stem plant over time. Furthermore, we examined the antioxidative effect of extracts from different culture types by measuring their scavenging activity on 1,1-diphenyl-2-picrylhydrazyl (DPPH) radicals as well as their anti-inflammatory potential on neonatal microglial rat cells activated by means of the inflammogen lipopolysaccharide (LPS) taking nitric oxide (NO) as parameter. Our results show that during an investigation period of 17 months, the shoot culture accumulated varying amounts of carnosic acid and carnosol, which were also present in callus culture but about 20- to 80-fold lower than in the shoot culture. In suspension culture, only carnosic acid and no carnosol could be detected. The level of carnosic acid in suspension culture was threefold less than detected for the callus culture on average. The amount of rosmarinic acid produced in shoot culture and callus culture were comparable, whereas in suspension culture higher concentrations of rosmarinic acid could be measured than in shoot and callus culture. Thus, the content of carnosic acid, carnosol, and rosmarinic acid in the extracts depended on the differentiation grade of the cell culture type. The DPPH radical-scavenging activity of the extracts depended on the amount of all three phytochemicals, in particular of rosmarinic acid. The anti-inflammatory character of the extracts was mainly based on their carnosic acid content.
Introduction
Rosemary (Rosmarinus officinalis. L.) belongs to the family Labiatae (Lamiaceae) and has been an important medicinal plant since earliest times. It is also a commonly used spice and flavoring agent. Its essential oil is used therapeutically and balneologically. Rosemary contains a large number of compounds responsible for its antioxidant, anti-inflammatory, antimutagenic, anticarcinogenic, chemopreventive, antimicrobial, and antiviral activities (Minnunni et al., Citation1992; Paris et al., Citation1993; Ho et al., Citation1994; Aruoma et al., Citation1996; del Campo et al., Citation2000; Lo et al., Citation2002; Oluwatuyi et al., Citation2004). Also, an induction of NGF (nerve growth factor) in human glioblastoma cells using rosemary extract has been described (Kosaka & Yokoi, Citation2003).
The main antioxidant compounds found in rosemary extract are rosmarinic acid, carnosic acid, and carnosol (Schwarz & Ternes, Citation1992b; Cuvelier et al., Citation1996; Shetty, Citation1997). Carnosic acid and carnosol are phenolic diterpenes of abietane type, and rosmarinic acid is an ester of caffeic acid and 3,4-dihydroxyphenyllactate (phenolic depside) (see for structures). Carnosic acid represents the main constituent of the phenolic diterpenes in rosemary.
Due to the antioxidative effect, rosemary extracts have been widely used in the food industry as a natural antioxidant as a stabilizer of fat and fat-containing foods. The potential of the antioxidative compounds of rosemary has also been researched with increasing interest for its application in pharmaceuticals.
Chan et al. (Citation1995) have demonstrated the anti-inflammatory activity of carnosol showing its inhibition of lipopolysaccharide (LPS) and interferon-γ (IFN-γ) induced nitrite production by mouse peritoneal cells. Later, it was shown that to a much lesser extent, carnosic acid and rosmarinic acid also reduce LPS-induced nitrite formation in RAW 264.7 cells (murine macrophage cell line). The mechanism for the anti-inflammatory activity of carnosol is based on the inhibition of nuclear factor-κB (NF-κB), p38, and p44/42 mitogen-activated protein kinase (MAPK) activation leading to a reduced inducible nitric oxide synthase (iNOS) expression and in consequence to reduced nitric oxide (NO) formation. Furthermore, it was suggested that this might be the mechanism of how special dietary phytochemicals prevent carcinogenesis associated with chronic inflammation (Lo et al., Citation2002).
For rosmarinic acid, the inhibition of several complement-dependent inflammatory processes has been widely studied (Rampart et al., Citation1986; Engleberger et al., Citation1988;). Whereas rosmarinic acid is found in substantial quantities in several species in the family Lamiaceae, it is also widespread in other families such as Borangiaceae (De-Eknamkul & Ellis, Citation1984; Shetty, Citation1997). The phenolic diterpenes carnosic acid and carnosol are found predominantly in Rosmarinus officinalis. and Salvia officinalis. subspecies (sage), which are members of the Lamiaceae (Cuvelier et al., Citation1996; Kintzios, Citation2000).
Previous studies have examined factors that increase the yield of phenolic antioxidant compounds in rosemary and sage plants under field and greenhouse conditions (Munné-Bosch et al., Citation1999Citation2001; Areias et al., Citation2000; Müller, Citation2004). These studies revealed that phenolic diterpenes are highly unstable depending on factors such as water stress, temperature, light, and oxygen contact. Therefore, sage and rosemary extracts show great differences in composition due to the variability of environmental factors. With in vitro. cultures, environmental and nutritional conditions are easily controlled, but alternative production mechanisms for phenolic diterpenes in vitro. have not been sufficiently studied.
Therefore, we have established in vitro. rosemary (Rosmarinus officinalis.) cultures of decreasing differentiation degree (shoot culture, callus culture, and suspension culture) in order to investigate their ability to biosynthesize antioxidant compounds, especially carnosic acid and carnosol. During a period of 17 months, we examined sample material in regular intervals. We examined whether all different in vitro. cultures would produce the desired products and, if they did, determined whether the products would accumulate over several subcultures and in quantities that were comparable with those of plants. Additionally, we examined the antioxidative and anti-inflammatory effects of the extracts based on the differentiation grade of the in vitro. culture they derive from. To determine the antioxidative effect, their scavenging activity on 1,1-diphenyl-2-picrylhydrazyl (DPPH) radicals was measured. Their anti-inflammatory potential was examined by means of neonatal microglial rat cells activated by the inflammogen LPS. The nitrite concentration of cell supernatants was taken as a parameter for NO formation of microglial cells due to inflammatory processes.
Materials and Methods
Plant material and growth medium
Seeds of the Rosmarinus officinalis. cv. variety “Hanging” were obtained from the nursery Rühlemann's Kräuter & Duftpflanzen (Horstedt, Germany). For establishment and subcultivation of rosemary in vitro. cultures, Murashige & Skoog medium (MS medium) including Nitsch and Nitsch vitamins (Duchefa no. M 0256, The Netherlands) with 3% (w/v) sucrose was used as basal medium. Solid MS medium contained 0.8% (w/v) agar and was supplemented with 50 mg/l ascorbic acid and 20 mg/l glutathione. Fluid medium consisted of MS basal medium with 50 mg/l ascorbic acid. The pH value of the medium was adjusted to 5.7.
Seed sterilization and germination
Seeds were rinsed in 70% ethanol for 1 min, surface sterilized with 1:10 diluted 12% sodium hypochlorite solution (Roth no. 9062.3) for 4 min, and washed three times with sterile distilled water. Ethanol and sodium hypochlorite solutions contained 0.02% Tween 80 (Serva). Surface-sterilized seeds were germinated on solid MS medium containing 0.5 mg/l kinetin. For germination, the seeds were cultivated in the dark at 25°C.
Establishment of rosemary in vitro. cultures
Seven days after germination, derived seedlings were excised and used as primary explants in the establishment of shoot cultures on solid MS medium supplemented with 1 mg/l 6-benzylaminopurine (BAP). Proliferated shoots were subcultured every 20–30 days on the same medium.
Leaf sections, approximately 5 mm long, of a 3-month-old in vitro. shoot culture were used for callus culture induction on solid MS medium with 4.7 mg/l BAP and 3.9 mg/l 1-naphthalene acetic acid (NAA). The tissue was maintained by regular subculturing at intervals of 20–30 days. Some of the callus tissues differentiated spontaneously and were subcultured separately on the same medium.
A cell suspension culture was established by transferring fresh undifferentiated callus of a 3-month-old culture to 50 ml of liquid MS medium with 4.7 mg/l BAP and 3.9 mg/l NAA in a 300-ml Erlenmeyer flask. Subcultures were performed in 10-dayto 11-day cycles by threefold dilution of the cells into fresh medium. Cultures were grown on a gyrotory shaker at 105 rpm.
In vitro. shoot culture, callus culture, and cell suspension culture were exposed to flourescent tubes (OSRAM, 1 × L 36 W/77 Fluora; 2 × L 36 W/31-830; 4 × l 36 W/21-840) and incubated at 25°C with a 11-h light/13-h dark cycle.
Preparation of crude methanol extracts from rosemary in vitro. cultures
Between 5 and 10 g of biomass fresh weight was taken as sample material at the beginning of the subculture. Approximately 160 mg of freeze-dried samples were extracted after grinding in centrifuge tubes with 4 ml of methanol containing 50 mg/l citric acid and 50 mg/l ascorbic acid. After 1 min of ultrasonication (Sonicator Bandelin Sonoplus HD 2200, Berlin, Germany), the extract was centrifuged for 3 min at 3°C and 1500 × g.. The supernatant was transferred into a 10-ml flask. The extraction procedure was repeated 3-times with 2 ml of solvent. The combined supernatants of the extracted sample were filled up to 10 ml and filtered through a folded filter (Macherey-Nagel, MN 615 1/4). The resulting extract was stored at −18°C prior to analysis.
Determination of carnosic acid and carnosol (HPLC analysis)
Carnosic acid (CA) and carnosol (CAR) content in the crude extract was quantified by HPLC on a reversed-phase Nucleosil column (120 Å pore size; 5 µm particle size; 250 × 4 mm) following the modified method of Schwarz and Ternes (Citation1992a) which was subsequently optimized by Müller (Citation2004).
The solvent system used was a gradient of eluent A methanol/water (50:50 v/v) with 0.5% phosphoric acid and eluent B methanol/water (97:3 v/v) with 0.25 phosphoric acid. The gradient was as follows: 0–8 min 85% A; 8–20 min 20% A; 20–25 min, 0% A, 25–30 min 20% A; 30–40 min 85% A.
The injection volumn was 10 µl, and the solvent flow rate was set at 0.6 ml/min. Peaks were detected at a wavelength of 230 nm with a variable wavelength detector (Agilent 1100, Waldbronn, Germany). Duplicates were run for each sample. Quantification was accomplished using a calibration curve of carnosic acid and carnosol obtained from Qbiogene-Alexis (Grünberg, Germany).
Determination of rosmarinic acid
The concentration of rosmarinic acid (RA) in the crude extract was determined directly by measuring the absorbance at 333 nm with an UV-visible spectrophotometer (DU 530 Spectrophotometer; Beckman, Sullerton, CA, USA). The calibration curve was established using rosmarinic acid in methanol. Duplicate measurements were determined for each sample.
DPPH radical-scavenging activity
A 1 ml ethanolic solution of 1,1-diphenyl-2-picrylhydrazyl (DPPH, 0.33 mM) was mixed with 1950 µl ethanol. The absorbance of the mixture was measured at 516 nm, and then 50 µl of crude extract was added. Fifteen minutes after adding the extract, the absorbance of the mixture was measured again at 516 nm. Triplicate measurements were made with a UV-visible spectrophotometer (DU 530 Spectrophotometer; Beckman). The antioxidant activity was calculated by the amount of DPPH that was scavenged using a calibration curve of DPPH in ethanol. It was expressed as number of reduced DPPH molecules per gram biomass dry weight (DW).
Microglial cultures
Microglial cells were prepared from rostral mesencephali and cerebral hemispheres of 2-day-old Wistar rats as described previously (Röhl & Sievers, Citation2005). Meninges, hippocampi, and choroid plexus were removed, and cortices and mesencephali were dissociated mechanically (by means of Pasteur pipettes) and enzymatically (with trypsin and DNAse I). Suspended cells of two hemispheres or one mesencephalon were plated per culture flask (75 cm2) in 10 ml growth medium [Dulbecco's modified Eagle's Medium (DMEM) supplemented with 10% (v/v) fetal calf serum (FCS; 53°C heat-inactivated), 1% (v/v) penicillin/streptomycin] [DMEM (no. 41965) and FCS (no. 10270), Gibco Invitrogen]. After approximately 1 week, floating microglial cells were harvested daily up to 3–4 weeks. Supernatants from these cultures were collected and centrifuged (700 × g., 5 min), and microglial cells were replated into 96-well microtiter plates (NO-, protein measurement, and viability assay) with a seeding density of 100,000 cells/cm2 (320,000 cells/ml). All cells were kept in astrocyte-conditioned medium (ACM) at 37°C and 7.8% CO2 until experiments were performed 3 to 4 days after seeding.
Treatment of microglia with LPS, extracts, and pure phytochemicals
A defined volume of a crude methanolic extract of either a shoot, callus, or suspension culture was evaporated before experiments in a Speedvac. Then they were dissolved like the pure phytochemicals carnosic acid and rosmarinic acid in DMSO and diluted with culture medium (final DMSO concentration 0.2%). LPS concentrations (no. 8274, Sigma) were made up in growth medium. For experiments, cells were treated with 310 µl/cm2. The concentration-effect relationship was examined for LPS (0.00625–0.05 µg/ml) and carnosic acid (2–100 µM). For examination of the anti-inflammatory potential of rosemary cell culture extracts, microglia were treated simultanously with the inflammogen LPS (0.02 µg/ml) and the extract. The extract with the highest carnosic acid content (from shoot culture) was made up with medium to the subcytotoxic concentration of 20 µM carnosic acid. The extracts from callus and suspension culture were diluted like that from shoot culture. A concentration of 40 µM for the pure rosmarinic acid control was chosen because it corresponded with the rosmarinic acid concentration of diluted extract from suspension culture, which contained the highest level of this compound. Measurement of cell protein, NO, or assessment of viability (MTT assay) were carried out after 24 h of incubation.
NO assay
Nitric oxide (NO) production as a parameter for inflammation was measured by a colorimetric method based on Griess reagent. By this method, nitrite as a derivative of the short-lived NO was determined in culture supernatants after the incubation period. NaNO2 was used as standard. The optical density (OD) was determined at 550 nm (Photometer 340 ATTC; SLT Labinstruments, Germany).
Viability assessment
Viability of the treated microglia was determined by means of the MTT [3-(4,5-dimethylthiazol-2-yl)-2,5-diphenyl-tetrazolium bromide] assay. Therefore, 25 µl MTT solution (1 mg/ml medium) was added after the incubation period to the microglia-containing wells and as background control to parallel wells without cells (37°C, 7.8% CO2). Solubilization solution [20% (w/v) SDS, 2.5% (v/v) 1 N HCl, and 2.5% (v/v) acetic acid (80%) in 50% (v/v) DMF, pH 2] (100 µl) was added 2 hours later. Production of formazan by viable cells was assessed after another 2 hours (37°C, 7.8% CO2) by measuring the absorbance at 550 nm (Photometer 340 ATTC; SLT Labinstruments Germany).
Protein measurement
To determine total cell protein concentrations of the samples and cytotoxic concentration of carnosic acid, cells were washed 3-times with PBS without Ca2+ and Mg2+ and incubated for 45 min with 0.5 N NaOH (60 µl/well). The cell protein contents were measured by colorimetric determination at 620 nm (Photometer 340 ATTC; SLT Labinstruments Germany) according to the method described by Lowry et al. (Citation1951). Bovine serum albumin was used as standard.
Phase-contrast microscopy
For morphological analysis, phase-contrast microscopy was performed with the inverted Axiovert 35 M microscope (OPTON, Germany), and cells were photographed using an Agfa Pan APX 100 professional black-and-white negative film (Agfa, Germany).
Results
Formation of phenolic antioxidant compounds in in vitro. cultures of rosemary and DPPH radical-scavenging activity
An in vitro. shoot culture was established from seeds of Rosmarinus officinalis. variety “Hanging.” This variety was chosen because investigations of 13 varieties of Rosmarinus officinalis. plants for their content of carnosic acid showed that this variety was the one with the highest content of approximately 2.8% of dry weight (Müller, Citation2004).
The content of carnosic acid, carnosol, and rosmarinic acid as well as the antioxidant activity expressed as number of reduced DPPH molecules was monitored over a 17-month period (). As shown in , the total amount of carnosic acid and carnosol varied during this time from approximately 8 to 65 mg/g biomass dry weight, and the amount of rosmarinic acid from 13 to 60 mg/g biomass dry weight. Usually, the concentration of rosmarinic acid was higher than those of carnosic acid and carnosol.
Table 1. Statistical analysis of the content of carnosic acid (CA), carnosol (CAR), and rosmarinic acid (RA) and DPPH radical-scavenging activity of crude methanolic extracts of in vitro. shoot culture, differentiated callus, and cell suspension of Rosmarinus officinalis. “hanging” measured during study period as shown in , , and .
Figure 2 Changes in the content of phenolic antioxidant compounds and in DPPH radical-scavenging activity in in vitro. shoot cultures of Rosmarinus officinalis. “hanging” over a period of 17 months [each value is the mean of two (CA, CAR, RA) or three (DPPH) measurements].
![Figure 2 Changes in the content of phenolic antioxidant compounds and in DPPH radical-scavenging activity in in vitro. shoot cultures of Rosmarinus officinalis. “hanging” over a period of 17 months [each value is the mean of two (CA, CAR, RA) or three (DPPH) measurements].](/cms/asset/6888f6da-fc0f-4a77-9dd2-0450428698ba/iphb_a_179353_f0002_b.gif)
The in vitro. shoot culture contained significantly higher amounts of carnosic acid and carnosol compared with the differentiated callus and cell suspension culture (p < 0.001).
The callus culture was initiated from leaf sections of the in vitro. shoot culture. Callus tissues differentiated spontaneously and could be maintained through subculturing. In these differentiated callus tissues, the total content of carnosic acid and carnosol was around 20- to 80-fold lower than in shoot culture. The content varied from 0.33 to 0.82 mg/g biomass dry weight. Rosmarinic acid was found in similar concentrations as in shoot culture (; ).
Figure 3 Changes in the content of phenolic antioxidant compounds and in DPPH radical-scavenging activity in differentiated callus cultures of Rosmarinus officinalis. “hanging” over a period of 10 months [each value is the mean of two (CA, CAR, RA) or three (DPPH) measurements].
![Figure 3 Changes in the content of phenolic antioxidant compounds and in DPPH radical-scavenging activity in differentiated callus cultures of Rosmarinus officinalis. “hanging” over a period of 10 months [each value is the mean of two (CA, CAR, RA) or three (DPPH) measurements].](/cms/asset/da083098-5b87-46d5-af13-ad8099d10dee/iphb_a_179353_f0003_b.gif)
In cell suspension culture, only carnosic acid and no carnosol could be detected (). The level of carnosic acid in suspension culture was three-fold less than detected for the differentiated callus culture on the average (). Rosmarinic acid could be measured in significantly higher concentrations than in shoot culture and differentiated callus culture (p < 0.001). The content ranged from 46 to 106 mg/g biomass dry weight ().
Figure 4 Changes in the content of phenolic antioxidant compounds and in DPPH radical-scavenging activity in suspension culture of Rosmarinus officinalis. “hanging” over a period of 14 months [each value is the mean of two (CA, CAR, RA) or three (DPPH) measurements].
![Figure 4 Changes in the content of phenolic antioxidant compounds and in DPPH radical-scavenging activity in suspension culture of Rosmarinus officinalis. “hanging” over a period of 14 months [each value is the mean of two (CA, CAR, RA) or three (DPPH) measurements].](/cms/asset/c48e0bac-d53e-4950-b4c9-84d8543ee041/iphb_a_179353_f0004_b.gif)
DPPH radical-scavenging activity of extracts from the in vitro. cultures (, 3B, 4B; see also ) depended primarily on the amount of rosmarinic acid, carnosic acid, and carnosol, which was represented by the coefficient of determination of r2 (). Rising concentrations of these substances in the extracts corresponded with higher numbers of reduced DPPH molecules per gram dry weight. On average, the suspension culture showed significantly higher DPPH radical-scavenging activity due to the high content of rosmarinic acid followed by shoot culture and differentiated callus culture (p < 0.001 cell suspension vs. shoot or differentiated callus, p < 0.05 shoot vs. callus). Although pure carnosic acid and rosmarinic acid showed similar scavenging activity toward DPPH radicals (see ), for the shoot culture with comparable contents of these compounds, the contribution of carnosic acid and carnosol to DPPH radical-scavenging activity was lower (r2 = 0.27;) than that of rosmarinic acid (r2 = 0.77; ). For the callus culture, the greatest part of the radical-scavenging activity was contributed by rosmarinic acid due to its low carnosic acid and carnosol content.
Figure 7 Anti-inflammatory (black bars) and antioxidative (white diamonds) effect of the pure phytochemicals carnosic acid (CA) and rosmarinic acid (RA) and of rosemary cell culture extracts deriving from cell cultures with different differentiation degrees that decreases in the order shoot, differentiated callus, and suspension culture, on LPS-stimulated microglial cells. Each bar/diamond represents the mean ± SE of three independent experiments. ***p < 0.001, **p < 0.01, *p < 0.05 versus control + LPS (ANOVA followed by Bonferroni test).
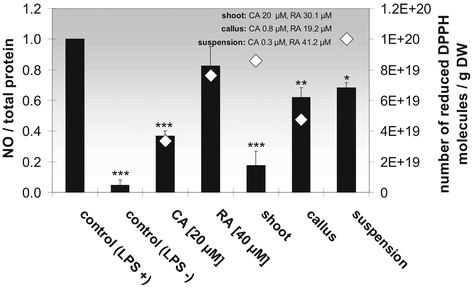
Anti-inflammatory potential of extracts from rosemary in vitro. cultures
In order to measure the anti-inflammatory potential of extracts from the rosemary in vitro. cultures on neonatal microglial rat cells, the inflammatory stimulus for microglial cells by examination of the concentration-effect relationship for LPS was optimized. shows, at very low LPS concentrations, opposite runs of the curve for NO formation (as parameter for inflammation) and formazan formation (MTT-assay). With increasing concentrations of LPS, NO increases, whereas formazan decreases. At about 0.0125 µg/ml, maximal inflammation and constant formazan formation are reached. Following these results, we chose 0.02 µg/ml LPS for the following stimulation experiments.
Figure 5 Effect of different concentrations of the inflammogen LPS on formazan formation (MTT assay) and NO production by microglial cells. A maximal microglia activation is reached at 0.0125 µg/ml LPS (24 h of incubation). MTT assay (black squares), NO measurement (white squares). Each point represents the mean ± SE of three independent experiments. ***p < 0.001 versus 0 µM carnosic acid (ANOVA followed by Bonferroni test).
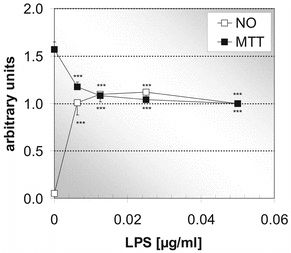
In further experiments (), we investigated the anti-inflammatory effect of pure carnosic acid on activated microglial cells. shows that at carnosic acid concentrations of 2–10 µM, NO/protein-ratios already decrease 20–30%. A further significant decrease was found at higher concentrations at 20–100 µM. These results only can be interpreted by analyzing both components (NO and cell protein) separately. At the lowest examined carnosic acid concentration, both total cell protein and NO formation are increased compared with controls. Whereas NO decreases continously with increasing carnosic acid concentrations (), cell protein contents remain approximately constant up to 50 µM and decrease rapidly thereafter (). Phase contrast micrographs show in parallel to cell protein determinations the toxic effect of carnosic acid at 100 µM compared with 20 µM. Different-shaped microglial cells, broad or compact with short processes, can be found in activated microglial cell cultures up to 20 µM, but at higher concentrations the toxic effect of carnosic acid leads to retraction of cell processes and rounding of the affected cells (). According to these results, a carnosic acid concentration of 20 µM was chosen as guiding value for the dilution of the rosemary cell culture extracts (). Contrarywise, the highest rosmarinic acid concentration of the three extracts (about 40 µM in the extract of suspension culture) was taken for the pure compound control. Concerning the pure antioxidants, it can be seen in that carnosic acid has a strong anti-inflammatory potential, reducing NO/protein ratios about 60% compared with controls, whereas rosmarinic acid only exhibits a slight anti-inflammatory effect. These properties of the pure phytochemicals are reflected by the anti-inflammatory potential of the three different extracts. The sample from shoot culture extract, which contains the highest carnosic acid concentration (20 µM), reduces the NO/protein ratio about 80%, but the sample from callus culture extract and the sample from suspension culture extract, containing very low carnosic acid concentrations (0.3 and 0.8 µM), decrease the NO/cell protein ratio only about 30%.
Figure 6 Effect of carnosic acid (different concentrations) and LPS (0.02 µg/ml) on NO formation (A), cell protein (B), morphology (C), and NO/cell protein-ratio (D). (A, B) Concentration-effect relationship. The curve with black squares represents the mean of three independent experiments (white squares). (C) Phase-contrast micrographs of treated microglia in culture. Scale bar: 90 µm. (D) Histogramm for the concentration-effect relationship. Each bar represents the mean ± SE of three independent experiments. ***p < 0.001, **p < 0.01 versus 0 µM carnosic acid (ANOVA followed by Bonferroni test).
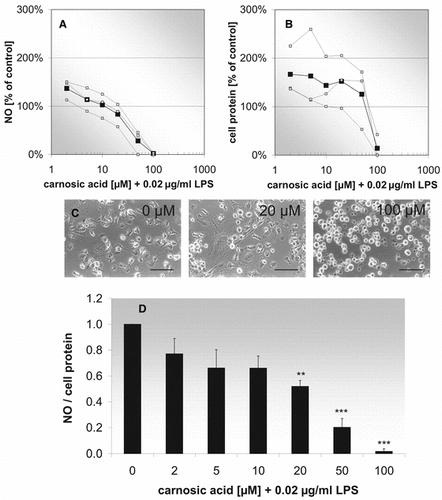
In parallel to the anti-inflammatory effect of the phytochemicals carnosic acid and rosmarinic acid, their antioxidative potential was determined by means of the DPPH assay. The antioxidative effect of 40 µM rosmarinic acid is comparable with the extract from shoot culture (CA 20 µM, RA 30.1 µM) and suspension culture (CA 0.3 µM, RA 41.2 µM), whereas 20 µM carnosic acid and extract from callus culture (CA 0.8 µM, RA 19.2 µM) only show half of its antioxidative effect ().
Discussion
The levels of carnosic acid and carnosol we found in in vitro. shoot cultures of rosemary are comparable with those reported for in vivo. grown rosemary plants. As described in the literature, the concentration varies in the range of 0.1% to 6% of dry weight according to climate conditions, agricultural factors, and individual origin (Hidalgo et al., Citation1998; Munné-Bosch et al., Citation2000; Müller, Citation2004). In all examined in vitro. cultures, the concentration of rosmarinic acid was either comparable with or higher than those reported for in vivo. grown plants. Normally, in vivo. grown plants contain up to 2.8% rosmarinic acid (Müller, Citation2004).
Rosmarinic acid has been widely investigated for its in vitro. production. It was produced by in vitro. cultures from different plant origin up to a concentration of 36% of biomass dry weight [6% in Anuchsa officinalis. (De-Eknamkul & Ellis, Citation1984)]; 21% in Coleus blumei. (Gertlowski & Petersen, Citation1993); 36% in Salvia officinalis. (Hippolyte, Citation2000). The highest concentration we could determine in our own experiments was about 10% in the cell suspension culture.
So far, there are few studies published regarding the in vitro. production of phenolic diterpenes (Caruso et al., Citation2000; Santos-Gomes et al., Citation2002; Santos-Gomes et al., Citation2003). Caruso et al. (Citation2000) have found carnosic acid in regenerated shoots obtained from leaf and nodal segments of Rosmarinus officinalis. and in 5-week-old nodular green callus, but not in non-green, undifferentiated callus. Quantitative data concerning the concentration of carnosic acid was not given, however.
With in vitro. shoots of sage obtained from 6-week-old seedlings, Santos-Gomes et al. (Citation2002) determined carnosic acid and carnosol at a value of 1.53 mg/g of biomass dry weight on average depending on the cytokinin supplementation. These data were obtained at the end of the seventh subculture period with intervals of about 5 weeks. Our investigations revealed that the in vitro. rosemary shoots showed levels of carnosic acid and carnosol about 5- to 42-times over that of the in vitro. sage shoots. Contrary to our results, the concentration of carnosic acid in sage shoots was about 10-fold lower than the concentration of carnosol.
Usually we found with in vitro. shoots much more carnosic acid than carnosol. One reason could be that carnosol is an artifact of carnosic acid due to its instability as described by many authors (Schwarz & Ternes, Citation1992b; Schwarz et al., Citation1992; Richheimer et al., Citation1996).
The same authors (Santos-Gomes et al., Citation2003) observed after nearly 6 months of subculturing in callus cultures of sage an average accumulation of carnosic acid and carnosol that was about 50- to 120-fold higher than the levels we have found in callus cultures of rosemary. Furthermore, they determined similar levels of carnosic acid and carnosol in suspension cultures of sage. However, these suspensions differentiated a high number of small root-shaped structures. In a single cell suspension culture, carnosic acid and carnosol were found only at trace amounts.
To interpret the anti-inflammatory effect of carnosic acid expressed as reduction of NO/cell protein ratio correctly (), the single components have to be analyzed separately ( and 6B). In lower concentrations, the decrease of NO/cell protein is predominantly due to enhanced protein levels. This means carnosic acid has a strong proliferating effect, which can be seen below 100 µM carnosic acid. This finding is in accordance with the tendency of macrophages either to proliferate or to become activated (Valledor et al., Citation2000). A similar effect can be seen in , where the MTT signal decreases until a maximum of microglial activation is accomplished. In contrast, NO formation decreases continously from 2 to 100 µM carnosic acid. Thus, despite nearly constant protein levels up to 50 µM of carnosic acid, NO declines. This means the significant decrease of NO/cell protein levels at 20 and 50 µM carnosic acid indicates anti-inflammatory potential. Lo et al. (Citation2002) examined the anti-inflammatory effect of carnosic acid on LPS-stimulated RAW 264.7 cells, a murine macrophage cell line. At 20 µM, they found a reduced NO generation of about 15%, which is in agreement with our results (). The decrease at the highest carnosic acid concentration in our experiments is ascribed to a toxic effect. The toxic effect can be seen on the phase-contrast photographs (, 100 µM). At 100 µM, microglial cells have retracted their processes completely. Detachment from the surface of the culture flasks leads to the loss of damaged cells during rinsing of the cultures for protein determination. Furthermore, Chan et al. (Citation1995) have shown that carnosol itself does not effect nitrite detection and, hence, is unlikely responsible itself for the NO decrease.
From a comparison of the anti-inflammatory potential of the in vitro. plant culture extracts with each other and with purified phytochemicals, it was found that carnosic acid is about 4-fold as effective as rosmarinic acid. Interestingly, Lo et al. (Citation2002) could not show a difference of the anti-inflammatory potential of these two phytochemicals in their LPS stimulated RAW 264.7 cells. They measured approximately the same anti-inflammatory potential of both substances. This might indicate different mechanisms of action that could lead to different anti-inflammatory effects in primary microglial cell cultures compared with the RAW 264.7 macrophage cell line. The antioxidant potential of carnosic acid and rosmarinic acid is very similar, in contrast with their anti-inflammatory potential. These results agree with those published by Lo et al. (Citation2002).
In conclusion, the biosynthesis of carnosic acid and carnosol is obviously dependent on the differentiation level of the in vitro. culture. Whereas the production of rosmarinic acid is not negatively influenced by the differentiation level, the biosynthesis of carnosic acid and carnosol is negatively influenced by morphological undifferentiation. Furthermore, we have shown that whereas the antioxidative effect is more a cumulative effect of the attendant phytochemicals, the anti-inflammatory character is mainly based on their carnosic acid content. These findings are also reflected by the anti-inflammatory and antioxidative properties of the rosemary cell culture extracts of varying diffentiation levels.
References
- Areias F, Valentao P, Andrate PB, Ferreres F, Seabra RM (2000): Flavonoids and phenolic acids of sage: Influence of some agriculture factors. J Agric Food Chem 48: 6081–6084. [INFOTRIEVE], [CSA]
- Aruoma OI, Spencer JPE, Rossi R, Aeschbach R, Khan A, Mahmood N, Munoz A, Murcia A, Butler J, Halliwell B (1996): An evaluation of the antioxidant and antiviral action of extracts of rosemary and provencal herbs. Food Chem Toxicol 34: 449–456. [INFOTRIEVE], [CSA], [CROSSREF]
- Caruso JL, Callahan J, DeChant C, Jayasimhulu K, Winget GD (2000): Carnosic acid in green callus and regenerated shoots of Rosmarinus officinalis.. Plant Cell Rep 19: 500–503. [CSA], [CROSSREF]
- Chan MM-Y, Ho C-T, Huang H-I (1995): Effects of three dietary phytochemicals from tea, rosemary and turmeric on inflammation-induced nitrite production. Cancer Lett 96: 23–29. [INFOTRIEVE], [CSA], [CROSSREF]
- Cuvelier ME, Richard H, Berset C (1996): Antioxidative activity and phenolic composition of pilot-plant and commercial extracts of sage and rosemary. J Am Oil Chem Soc 73: 645–652. [CSA]
- De-Eknamkul W, Ellis BE (1984): Rosmarinic acid production and growth characteristics of Anchusa officinalis. cell suspension cultures. Planta Med 51: 346–350. [CSA]
- del Campo J, Amiot MJ, Nguyen-The C (2000): Antimicrobial effect of rosemary extracts. J Food Protect 63: 1359–1368. [CSA]
- Engleberger W, Hadding V, Etschenberg E, Graf E, Leyck S, Winkelmann J, Parnham MJ (1988): Rosmarinic acid. A new inhibitor of coplement C3-convertase with anti-inflammatory activity. Int J Immunopharmacol 10: 729–737. [CSA], [CROSSREF]
- Gertlowski K, Petersen M (1993): Influence of the carbon source on growth and rosmarinic acid production in suspension cultures of Coleus blumei.. Plant Cell Tiss Org 34: 183–190. [CSA], [CROSSREF]
- Hidalgo PJ, Ubera JL, Tena MT, Valcarcel M (1998): Determination of the carnosic acid content in the wild and cultivated Rosmarinus officinalis.. J Agric Food Chem 46: 2624–2627. [CSA], [CROSSREF]
- Hippolyte I (2000): In vitro. rosmarinic acid production. In: Kintzios SE, ed., Sage: The Genus Salvia. Amsterdam, Harwood Academic Publishers, pp. 233–242.
- Ho C-T, Ferraro T, Chen Q, Rosen RT, Huang M-T (1994): Phytochemicals in teas and rosemary and their cancer-preventive properties. In: Ho C-T, Toshihiko O, Huang M-T, Rosen RT, eds., Food Phytochemicals for Cancer Prevention II. Washington, DC, American Chemical Society, pp. 2–19.
- Kintzios SE (2000): Sage: The Genus Salvia. Amsterdam, Harwood Academic Publishers, pp. 225–263.
- Kosaka K, Yokoi T (2003): Carnosic acid, a component of rosemary (Rosmarinus officinalis. L.) promotes synthesis of nerve growth factor in T98G human glioblastoma cells. Biol Pharm Bull 26: 1620–1622. [INFOTRIEVE], [CSA], [CROSSREF]
- Lo A-H, Liang Y-C, Lin-Shiau S-Y, Ho C-T, Lin J-K (2002): Carnosol, an antioxidant in rosemary, supresses inducible nitric oxide synthase through down-regulating nuclear factor- κB in mouse macrophages. Carcinogenesis 23: 983–991. [INFOTRIEVE], [CSA], [CROSSREF]
- Lowry OH, Rosebrough NJ, Farr AL, Randall RJ (1951): Protein measurement with the Folin phenol reagent. J Biol Chem 193: 265–275. [INFOTRIEVE], [CSA]
- Minnunni M, Wolleb U, Mueller O, Pfeifer A, Aeschbacher HU (1992): Natural antioxidants as inhibitors of oxygen species induced mutagenicity. Mutat Res 269: 193–200. [INFOTRIEVE], [CSA]
- Müller M (2004): Formation of antioxidants and essential oil in sage and rosemary grown under Mediterranean and Central European climate. Thesis, Kiel: Christian-Albrechts-Universität, Agrar- und Ernährungswissenschaftliche Fakultät.
- Munné-Bosch S, Alegre L, Schwarz K (2000): The formation of phenolic diterpenes in Rosmarinus officinalis. L. under Mediterranean climate. Eur Food Res Technol 210: 263–267. [CSA], [CROSSREF]
- Munné-Bosch S, Schwarz K, Alegre L (1999): Response of abietane diterpenes to stress in Rosmarinus officinalis. L.: New insights into the function of diterpenes in plants. Free Radical Res 31: 107–112. [CSA]
- Munné-Bosch S, Müller M, Schwarz K, Alegre L (2001): Diterpenes and antioxidative protection in drought-stressed Salvia officinalis. plants. J Plant Physiol 158: 1431–1437. [CSA], [CROSSREF]
- Oluwatuyi M, Kaatz GW, Gibbons S (2004): Antibacterial and resistance modifying activity of Rosmarinus officinalis.. Phytochemistry 65: 3249–3254. [INFOTRIEVE], [CSA], [CROSSREF]
- Paris A, Strukelj B, Renko M, Turk V (1993): Inhibitory effect of carnosolic acid on HIV-1 protease in cell-free assay. J Nat Prod 56: 1426–1430. [INFOTRIEVE], [CSA], [CROSSREF]
- Rampart M, Beetens JR, Bult H, Herman AG, Parnham MJ, Winkelmann J (1986): Complement-dependent stimulation of prostacyclin biosynthesis: Inhibition by rosmarinic acid. Biochem Pharmacol 35: 1397–1400. [INFOTRIEVE], [CSA], [CROSSREF]
- Richheimer SL, Bernart MW, King GA, Kent MC, Bailey DT (1996): Antioxidant activity of lipid-soluble phenolic diterpenes from rosemary. J Am Oil Chem Soc 73: 507–514. [CSA]
- Röhl C, Sievers J (2005): Microglia is activated by astrocytes in trimethyltin intoxication. Toxicol Appl Pharmacol 204: 36–45. [CSA], [CROSSREF]
- Santos-Gomes PC, Seabra RM, Andrade PB, Fernandes-Ferreira M (2002): Phenolic antioxidant compounds produced by in vitro. shoots of sage (Salvia officinalis. L.). Plant Sci 162: 981–987. [CSA], [CROSSREF]
- Santos-Gomes PC, Seabra RM, Andrade PB, Fernandes-Ferreira M (2003): Determination of phenolic antioxidant compounds produced by calli and cell suspensions of sage (Salvia officinalis. L.). J Plant Physiol 160: 1025–1032. [INFOTRIEVE], [CSA], [CROSSREF]
- Schwarz K, Ternes W (1992a): Antioxidative constituents of Rosmarinus officinalis. and Salvia officinalis.. I. Determination of phenolic diterpenes with antioxidative activity amongst tocochromanol using HPLC. Z Lebens-unters Forsch 195: 95–98. [CSA], [CROSSREF]
- Schwarz K, Ternes W (1992b): Antioxidative constituents of Rosmarinus officinalis. and Salvia officinalis.. II. Isolation of carnosic acid and formation of other phenolic diterpenes. Z Lebens-unters Forsch 195: 99–103. [CSA], [CROSSREF]
- Schwarz K, Ternes W, Schmauderer E (1992): Antioxidative constituents of Rosmarinus officinalis. and Salvia officinalis.. III. Stability of phenolic diterpenes of rosemary extracts under thermal stress required for technological process. Z Lebens-unters Forsch 195: 104–107. [CSA], [CROSSREF]
- Shetty K (1997): Biotechnology to harness the benefits of dietary phenolics; Focus on Lamiaceae. Asia Pacific J Clin Nutr 6: 162–171. [CSA]
- Valledor AF, Comalda M, Xaus J, Celda A (2000): The different time-course of extracellular-regulated kinase activity correlates with the macrophage response toward proliferation of activation. J Biol Chem 275: 7403–7409. [INFOTRIEVE], [CSA], [CROSSREF]