Abstract
Hypoxia/reoxygenation causes cellular injury and death associated with many pathophysiologic conditions, including respiratory disorders, myocardial ischemia, and tumour progression diseases. Neuronal pheochromocytoma (PC12) cells are widely used as a model system for neurologic research and are subject to chemical hypoxia induced with sodium hydrosulfite (Na2S2O4), a common oxygen-consuming agent. Salidroside, which is the main active component of the famous traditional Chinese herb Rhodiola rosea. L. (Crassulaceae), has been proved to possess many bioactivities. In this article, we studied the protective effects of salidroside on hypoxia/reoxygenation injury in PC12 cells induced by Na2S2O4. Cultures of PC12 cells were exposed for 1 h to 10 mM Na2S2O4 for hypoxia, followed by reoxygenation for 2 h. The results showed that salidroside was very stable in medium and was not harmful to PC12 cells at the experimental concentrations of 0 ∼ 200 µg/mL. The cytoprotection by salidroside was dose-dependent, and the cell viability was 41.8 ± 5.7%, 62.4 ± 4.1%, and 92.2 ± 3.7% at 0, 50, and 100 µg/mL of salidroside, respectively. The level of released LDH significantly decreased from 513.5 ± 5.5% (without salidroside) to 258.1 ± 6.3% (with 100 µg/mL salidroside). Flow cytometry was performed to measure apoptotic rate. The results of flow cytometry assay indicated that the apoptotic rate was 17.0 ± 1.2% after hypoxia/reoxygenation injury. When the cells were treated with salidroside 12.5, 50 and 100 µg/mL, the apoptotic rate was 9.5 ± 0.9%, 7.4 ± 0.5%, and 4.5 ± 0.4%, respectively. In addition, our results were confirmed by inspection of cell morphology of PC12 cells. Treatment with salidroside (12.5, 50, 100 µg/mL) significantly prevented the cells from morphologic changes. All the above results showed salidroside could effectively protect PC12 cell against hypoxia/reoxygenation injury.
Introduction
Mammalian cells are critically dependent on oxygen for survival. Hypoxia, or hypoxia/reoxygenation, arises during a variety of pathophysiologic states such as ischemia, respiratory disease, and tumorigenesis (Xue, Citation2004). It has been reported that hypoxia/reoxygenation can generate superoxide, promote the recruitment of inflammatory leukocytes, cause cell death involving both necrotic and apoptotic events, and induce specific gene expression in the neuronal cells (Cazevieille et al., Citation1993; Yun et al., Citation1997). Thus, hypoxia/reoxygenation is an important physiologic factor in the respiratory disorders, ischemia, and tumor progression diseases (Dana et al., Citation2002). In an effort to prevent or diminish hypoxia/reoxygenation damage, investigators have evaluated many compounds that could reduce cellular damage. In recent years, more and more researchers have investigated the function of natural compounds. Many studies show a number of products provide antihypoxia action, including sesamin, seasmolin, ginkgolid, and an extract of Curcuma longa. L. (Zingiberaceae) (Chen et al., Citation2001; Hou et al., Citation2003; Koo et al., Citation2004).
Rhodiola rosea. L. (Crassulaceae), also known as “Arctic root” or “rose root,” is a medicinal plant in Asia and Eastern Europe. It is a member of the Crassulaceae family, a perennial herbaceous plant growing in the Arctic and the mountainous regions throughout Europe and Asia. The genus Rhodiola. consists of about 200 species (German & Ramazanov, Citation1999), in which nearly 70 species are distributed in the southwest, northwest, and northeast regions in China (Li & Yu, Citation2002). In Siberia, folklore states that people who drink a cup of Rhodiola rosea. tea every day will live for more than 100 years. Strong evidence indicates this plant possesses biological activity with few side effects (German & Ramazanov, Citation1999; Darbinyan, Citation2000). Traditionally, the plant has been documented as useful in the treatment of many illnesses including enhancing work performance, decreasing depression, eliminating fatigue, preventing high-altitude sickness, and so on (Kelly, Citation2001). It has long been known to have adaptogenic properties (Brekhman & Dardymov, Citation1969). Recently, researchers have found that it can elevate concentrations of norepinephrine, dopamine, and serotonin in the brain, act as a nicotinic cholinergic agonist in the central nervous system, reduce the level of C-reactive protein and creatinine kinase in the blood, increase the ATP content in the muscle mitochondria, and has antiarrhythmic activity, anti–prostate cancer activity, and antibacterial activity (Maslov et al., Citation1998; Abidov et al., Citation2003Citation2004; Ming et al., Citation2005).
The main active component of R. rosea. is salidroside [2-(4-hydroxyphenyl)-ethyl-β-D-glucopyranoside]. The chemical structure of this component is shown in . Salidroside has been shown to possess medical functions such as resisting microwave radiation and fatigue (Saratikov, Citation1968; Ming et al., Citation1988). It also has been reported to be used in such special positions such as diver, astronaut, pilot, and mountaineer to enhance the body's ability to survive in adverse environments (Ming et al., Citation1988). Furthermore, its effect on extending human life was reported (Kurkin, Citation1986). Also, salidroside, which has been shown to have antioxidant activity, can reduce the intracellular accumulation of reactive oxygen species and malondialdehyde, and prevent decline of antioxidant enzyme activities (Jang et al., Citation2003). However, until now, the effect of salidroside against hypoxia/reoxygenation insult has not been reported. Neuronal pheochromocytoma (PC12) cells are widely used as a model system for neurologic research, and they are subject to chemical hypoxia induced with sodium hydrosulfite (Na2S2O4), a common oxygen-consuming agent. Thus, we used PC12 cells to evaluate the effect of salidroside in preventing the hypoxia/reoxygenation injury induced by Na2S2O4.
There is an important relevancy among cell viability, the percentage of apoptotic level, and the extracellular lactate dehydrogenase LDH activity of PC12 cells. When the cells are damaged, those indexes will be changed. In this study, cell viability, apoptotic rate, and the extracellular LDH activity of PC12 cells were examined. The purpose was to determine whether treatment with salidroside would reduce hypoxia/reoxygenation injury induced by Na2S2O4in PC12 cells.
Materials and Methods
Materials
Standard extracts of Rhodiola rosea. were provided by Tianjin Jianfeng Natural Product R&D Co., Ltd. (Tianjin, China), and the content of salidroside was about 2.0%. Salidroside was isolated and identified from these extracts by our lab, and the purity was above 98% (HPLC). 3-(4,5-Dimethylthiazol-2-yl)-2,5-diphenyl (MTT) was purchased from Bioseen Technology, Inc. (Shanghai, China). PC12 cell lines were purchased from Chinese Type Culture Collection (Shanghai Institute of Cell Biology, Chinese Academy of Science, Shanghai, China). RPMI 1640 Medium was purchased from GIBCO BRL (Grand Island, NY, USA). Fetal bovine serum was purchased from Hangzhou Sijiqing Co., Ltd. (Hangzhou, China). LDH activity kit was purchased from Shanghai Rongsheng Biotechnology Co., Ltd. The other regents were analytic reagents (AR).
Cell and cell culture
PC12, which is a rat cell line derived from a pheochromocytoma, was cultured in RPMI 1640 Medium with 10% dialyzed heat-inactivated bovine serum, penicillin (100 U/mL), and streptomycin (100 U/mL) at 37°C in a humidified atmosphere of 95% air and 5% CO2. In every experiment, the cells were in their exponential growth phase. Salidroside was freshly prepared in medium before the experiment. Control cultures were performed in the presence of normal medium under the same culture conditions. To produce hypoxia/reoxygenation stress, Na2S2O4 was freshly prepared in medium prior to each experiment. The cells were preincubated in different concentrations of salidroside for 16 h before Na2S2O4 was added. After hypoxic incubation for 1 h, the medium was changed with the former concentration of salidroside for reoxygenation 2 h. Assays for cell viability, LDH activity, and apoptotic rate were performed after hypoxia/reoxygenation.
Isolation, purification, and identification of salidroside from the Rhodiola rosea. extract
The Rhodiola rosea. extract (100 g) was dissolved in 300 mL water by ultrasonication and extracted subsequently with petroleum ether and ethyl acetate. Only the water layer contained salidroside. Therefore, this fraction was chosen and applied to a macroporous resin column to enrich salidroside (column 50 × 5 cm i.d.). The column was eluted with 0% (2.0 l), 10% (2.0 l), 20% (2.0 l), 30% (2.0 l), 50% (2.0 l) ethanol in water to obtain five different fractions as shown in . Fraction 2 and fraction 3 were detected to contain salidroside by HPLC, so we combined these two fractions. The fraction was further separated with a silica gel column (40 × 10 cm i.d.) and eluted with 40% (5.0 l) methanol in chloroform to obtain 1.5 g of about 95% salidroside. Then, it was recrystallized many times in methanol at room temperature, and 0.8 g salidroside (> 98%, detected by HPLC) was obtained.
The identification of salidroside was accomplished by direct HPLC comparisons with a standard from Sigma and by spectroscopic methods (UV, IR, FAB-MS, 1H NMR and 13C NMR). The spectral data of salidroside corresponded with published values (Chen et al., Citation1999).
Assay for cells survival
The exponentially growing PC12 cells were plated in 96-well plates at a density of 1.5 × 103 to 2.0 × 103 cells/well. When the cells were grown to approximately 80% confluence, culture medium was changed with various concentrations of salidroside and further cultured for 16 h. Then, the cells were exposed to different concentrations of Na2S2O4 for 1 h. After hypoxic incubation, the medium was replaced with the former concentration of salidroside for reoxygenation 2 h. Cell viability was measured by the conventional MTT reduction assay. After incubation, cells were treated with 0.5 mg/mL MTT (dissolved in PBS and filtered through an 0.22-µm membrane) for 4 h. The dark-blue formazan crystals formed in intact cells were dissolved in dimthyl sulfoxide (DMSO), and absorbance at 550 nm was measured with an automated Bio-Rad 550 microtiter plater reader (CA, USA).
Assay for the stability of salidroside
Salidroside (100 µg/mL) was dissolved in RPMI 1640 medium and incubated at 37°C in a humidified atmosphere of 95% air and 5% CO2 for different times. The solution was centrifuged at 10,000 rpm for 15 min at 4°C, and then the supernatant was filtrated through an 0.45-µm membrane. The supernatant was assayed by HPLC to test the stability of salidroside. The chromatographic data were recorded and processed with Agilent Chromatographic Work Station software (Palo Alto, CA, USA). Analysis was carried out at 35°C on an Agilent Eclipse XDB-C18 column (5.0 µm, 150 × 4.6 mm, i.d.), which was protected by a guard column (3.5 µm, 12.5 × 4.6 mm, i.d.). The mobile phase was methanol and water (20:80 v/v), with a flow rate of 1.0 mL min−1. It was detected at 278 nm with a retention time of 5.4 min.
Assay for LDH
Cell damage was evaluated via the leakage of LDH using a colorimetric assay. PC12 cells with various concentrations of salidroside were treated with Na2S2O4 for hypoxia/reoxygenation. The culture medium was collected. Subsequently, 100 µL of supernatant for extracellular samples was added to a polystyrene cuvette containing 900 µL of LDH reagent (50 mmol/L lactate, 7 mmol/L NAD+ in 0.05% sodium azide buffer, pH = 8.9). Spectrophotometric analysis was performed at room temperature, and the absorption values were determined at 340 nm. The absorbance was recorded at 1-min intervals for 3 min after reaction for 1 min. Then the change in absorbance was expressed in concentration units per liter and converted to percentage of control levels.
Analysis of apoptosis by flow cytometry
Apoptotic rate was measured by flow cytometry. Briefly, the PC12 cells that were pretreated with different concentrations of salidroside and then treated with Na2S2O4 for hypoxia/reoxygenation (1 h/2 h) were collected by centrifugation at 1000 rpm for 10 min, then rinsed with PBS (pH = 7.4). The cell pellets were resuspended in cold 70% (v/v) ethanol and fixed at − 20°C for 24 h. The cells were then centrifuged (1000 rpm for 15 min), and ethanol was removed by rinsing thoroughly with PBS. The cell pellets were resuspended in 2 mL PBS containing 100 µg/mL RNase A, 0.1% Triton X-100, 0.1 mM EDTA, and incubated at 37°C for 30 min. After incubation, the cells were stained with 100 µg/mL propidium iodide (PI) in the dark for 30 min at 4°C. The fluorescence of cells was measured with a FACScan (Becton Dickinson, Mountain View, CA, USA). The relative content of DNA indicated the distribution of a population of cells throughout the cell cycle. Apoptotic cells caused the appearance of a subdiploid peak in the cell-cycle profile. The percentage of apoptotic cells was determined by using Cell Fit software.
Statistical analysis
All experiments were done in triplicate. Data were expressed as mean ± SD and were analyzed by paired t.-test. The scientific statistical software Origin version 7.0 was used to evaluate the significance of differences between groups. The criterion of statistical significance was *p < 0.05, **p < 0.01, and ***p < 0.001 in comparing with control group (control group: without salidroside and Na2S2O4).
Results
Cell toxicity induced by Na2S2O4
It has been reported that chemical hypoxia/reoxygenation was simulated by the addition and the removal of chemicals such as NaN3 or CoCl2 or Na2S2O4 (Rose et al., Citation1998; Wang et al., Citation2000; Zhang et al., Citation2005). The neurons are extremely sensitive to hypoxia and reoxygenation insult (Faroqui et al., Citation1994). In order to investigate the protective effects of salidroside on hypoxia/reoxygenation injury, we use PC12 cells induced by Na2S2O4. The cell viability was measured by the conventional MTT assay. As shown in , the viability of cells exposed to 10 mM Na2S2O4 for 1 h for hypoxia and reoxygenation for 2 h was 51% of the control group. Thus, we chose 10 mM Na2S2O4 for hypoxia 1 h and reoxygenation 2 h to treat PC12 cells in subsequent experiments.
Figure 3 Effects of Na2S2O4-induced cytotoxicity on PC12 cells. Confluent cells in 96-well plates were treated with different concentrations in the presence of Na2S2O4 and then changed to normal medium for 2 h. Cell viability was determined by the MTT assay, and the data were expressed as X ± SD, n = 3. *p < 0.05, **p < 0.01, and ***p < 0.001 in comparison with normal cells.
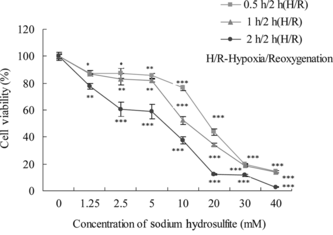
Toxicologic effects of salidroside on PC12 cells
We concluded that salidroside had no harmful or inhibitory effects on the growth of PC12 cells with increasing dose (). The cells were treated with salidroside at the concentrations of 0–200 µg/mL for 24 h, and the cell viability was not changed at all. The results demonstrated that salidroside was not harmful to PC12 cells at the experimental concentration range.
The stability of salidroside in RPMI 1640 medium
Based on the standard curve (not shown in this article, Y. = 4.5233X. − 2.9086; R2 = 0.9999), the stability of salidroside is shown in . It was very stable in RPMI 1640 medium at 37°C in a humidified atmosphere of 95% air and 5% CO2 for 0–48 h. After incubation for 48 h, the residual concentration of salidroside in culture medium was 97.0%, compared with the control group (not incubated). The study showed salidroside was stable in medium in the experimental time range and the subsequent experiment was practicable.
Figure 5 The stability of salidroside in RPMI 1640 medium. Salidroside (100 µg/mL) was dissolved in RPMI 1640 medium and then incubated at 37°C in a humidified atmosphere of 95% air and 5% CO2. The solution was centrifuged at 10,000 rpm for 15 min at 4°C, and then the supernatant was filtrated through an 0.45-µm membrane. The supernatant was assayed by HPLC.
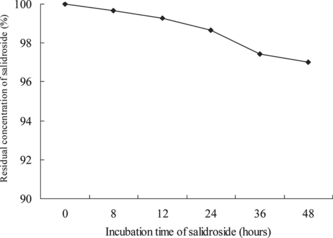
Protective effects of salidroside on hypoxia/reoxygenation injury and morphologic inspection of PC12 cells
To examine whether salidroside has protective effects on Na2S2O4-treated PC12 cells, the connection between Na2S2O4 and salidroside was demonstrated. With cells exposed to hypoxia/reoxygenation insult alone (in the absence of salidroside), viability was 41.8 ± 5.7% of control group (). With increasing concentrations at 3.13–100 µg/mL, the protective role of salidroside on the PC12 cells increased in a dose-dependent manner. Compared with the control group, salidroside increased cell viability by 49.0 ± 7.4%, 62.4 ± 4.1%, and 92.2 ± 3.7% at 6.25, 50, and 100 µg/mL, respectively (). These results indicated that salidroside had a protective effect against hypoxic/reoxygenation stress on PC12 cells. The hypoxia/reoxygenation insult was accompanied by typical morphologic changes, such as cell body detachment, damage to neurite outgrowths, and collapse of growth cones. But these morphologic changes were prevented when the cells were treated with different concentrations of salidroside (). The results corresponded with the MTT assy.
Figure 6 Protective effects of salidroside on hypoxia/reoxygenation injury. Confluent PC12 cells were pretreated with different concentrations of salidroside in 96-well plates for 16 h. Then, the cells were exposed to 10 mM Na2S2O4 for 1 h for hypoxic damage. After hypoxic incubation, the medium was replaced with the former concentration of salidroside for reoxygenation for 2 h. Cell viability was determined by the MTT assay and the data were expressed as X ± SD, n = 3. *p < 0.05, **p < 0.01, and ***p < 0.001 in comparison with hypoxia/reoxygenation injury group cells.
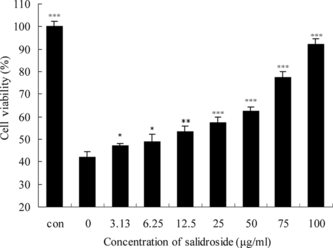
Figure 7 Morphologic inspection of PC12 cells. Confluent PC12 cells were pretreated with different concentrations of salidroside in 96-well plates for 16 h. Then, the cells were exposed to 10 mM Na2S2O4 for 1 h for hypoxic damage. After hypoxic incubation, the medium was replaced with the former concentration of salidroside for reoxygenation for 2 h. Salidroside could prevent the cells from morphologic changes: (1) control group (without salidroside and Na2S2O4), (2) hypoxia/reoxygenation injury group, (3) hypoxia/reoxygenation injury and 100 µg/mL salidroside, (4) hypoxia/reoxygenation injury and 50 µg/mL salidroside, (5) hypoxia/reoxygenation injury and 12.5 µg/mL salidroside.
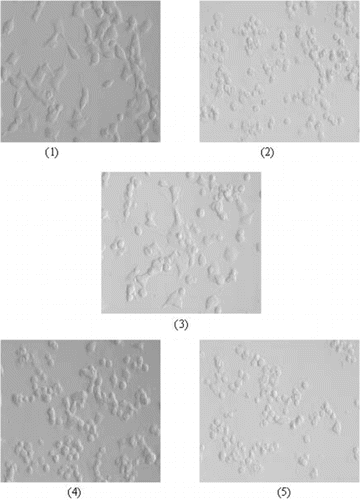
Effects of salidroside on the extracellular LDH levels in PC12 cells
To further investigate the protective effects of salidroside on hypoxia/reoxygenation injury in PC12 cells, the LDH assay was considered as an indicator. LDH is a stable cytoplasmic enzyme in all cells, and when the cell plasma membrane was damaged. LDH was rapidly released in the culture medium. Thus, the increase of LDH activity in the culture medium can indicate the degree of cellular damage. After exposure to hypoxia/reoxygenation insult, LDH activity increased to 513.5 ± 5.5% of control group, indicating that the cells were damaged markedly. The result was similar to that obtained by morphologic inspection (). The treatment with salidrosede at concentrations between 3.13 and 100 µg/mL had significant effects on the decrease of LDH activity in culture medium, from 400.0 ± 6.5% (with 3.13 µg/mL salidroside) to 258.1 ± 6.3% (with 100 µg/mL salidroside) (). These results suggest that salidroside effectively reduced the release of LDH by hypoxia/reoxygenation in a concentration-dependent manner.
Figure 8 Effects of salidroside on extracelluar LDH activity in PC12 cells. Confluent cells were preincubated with various concentrations of salidroside for 16 h. After hypoxia/reoxygenation injury, the medium was collected and the activity of LDH was determined using LDH test kits according to the manufacturer's protocol. The data were expressed as X ± SD, n = 3. *p < 0.05, **p < 0.01, and ***p < 0.001 in comparison with hypoxia/reoxygenation injury group cells.
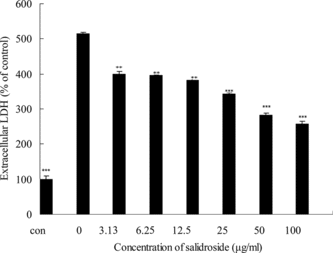
Effects of salidroside on the apoptotic rate in PC12 cells
In order to determine whether salidroside can block Na2S2O4-induced apoptosis in PC12 cells, we measured the apoptotic rate by flow cytometry. In recent years, flow cytometry has emerged as a rapid and sensitive technique for studying cell membranes and intracellular changes of various cell types. The apoptotic rate in flow cytometry detection is considered as an indicator of cell damage. Analyzed by flow cytometry, the apoptotic rate increased significantly in PC12 cells treated with hypoxia/reoxygenation insult, up to 17.0 ± 1.2% [(1)]. As shown in (3), (4), and (5), the treatment with salidroside 12.5, 50, and 100 µg/mL decreased the Na2S2O4-induced apoptotic rate, 9.5 ± 0.9%, 7.4 ± 0.5%, and 4.5 ± 0.4%, respectively. These results are consistent with MTT, LDH assay, and the morphologic inspection.
Figure 9 Effects of salidroside on the apoptotic rate in PC12 cells. Apoptotic rate was measured by flow cytometry. (1) control group (without salidroside and Na2S2O4), (2) hypoxia/reoxygenation injury group, (3) hypoxia/reoxygenation injury and 12.5 µg/mL salidroside, (4) hypoxia/reoxygenation injury and 50 µg/mL salidroside, (5) hypoxia/reoxygenation injury and 100 µg/mL salidroside. The data were expressed as X ± SD, n = 3. **p < 0.01 and ***p < 0.001 in comparison with hypoxia/reoxygenation injury group cells.
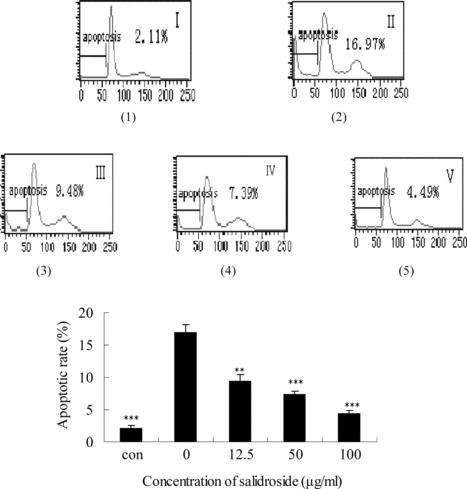
Discussion
It has been reported that hypoxia/reoxygenation injury involved a number of factors, including reactive oxygen species, nitric oxide, oxygen free radical, N.-methyl-D-aspartate, intracellular free calcium, and protein kinase C (Cazevieille et al., Citation1993; Yun et al., Citation1997; Bossenmeyer, Citation1998; Wang & Shum, Citation2002). But recently, most researchers consider oxidative stress as a major factor after hypoxia/reoxygenation injury (Cazevieille et al., Citation1993; Chan, Citation1996; Yun et al., Citation1997). Oxidative stress can be defined as the pathogenic outcome of the overproduction of oxidants that overwhelm the antioxidant capacities of free radical quenching enzymes such as superoxide dismutase (SOD), catalase (CAT), and glutathione peroxidase (GPx) (Machlin & Bendich, Citation1987; Koo et al., Citation2004). The major cytotoxic effect of oxidative stress on cells is supposed to be the peroxidation of lipid components of cellular and subcellular membranes. The resulting loss of cellular integrity could lead to irreversible cell injury. Changes induced by hypoxia/reoxygenation injury can include an increase in oxidant factors, and impairment of endogenous antioxidant systems. PC12, which is a tumor cell line derived from rat pheochromocytoma, is widely applied in neurologic research (Wang et al., Citation2000). Chemical hypoxia/reoxygenation was simulated by the addition and the removal of chemicals such as NaN3or CoCl2 or Na2S2O4 (Rose et al., Citation1998; Wang et al., Citation2000; Zhang et al., Citation2005).
In the current study, salidroside, one of the major compounds from the roots of a famous traditional Chinese herb Rhodiola rosea., was investigated for its cytoprotection against Na2S2O4-induced hypoxia/reoxygenation on PC12 cells. The toxic effects of Na2S2O4 resulted in decrease in the viability of PC12 cells (). An incubation of the PC12 cells with different doses of salidroside protected the cells from the cytotoxic effects of hypoxia/reoxygenation insult in a dose-dependent manner (), and the corresponding extracelluar LDH levels decreased concurrently (). Interestingly, we observed the higher apoptotic rate after exposure of the cells to hypoxia/reoxygenation insult, and with the increased concentration of salidroside the values gradually decreased. Thus, salidroside appeared to play a protective role against neuronal injury after hypoxia/reoxygenation.
In the previous study, the Rhodiola rosea. aqueous extract was proven to have antioxidant activity. It could protect human erythrocytes against in vitro. damage induced by HOCl (Roberta, Citation2004). Salidroside, which is the main active component in Rhodiola rosea., has been shown to have antioxidant activity. It can reduce the intracellular accumulation of reactive oxygen species and malondialdehyde and prevent decline of antioxidant enzyme activities (Jang et al., Citation2003). The antioxidant mechanism may be the structure similarity of salidroside to p.-tryrosol, which is a superior antioxidant.
In conclusion, our findings suggest that salidroside reduced PC12 cells from death induced by Na2S2O4 in a concentration-dependent manner, and the potential mechanism of this neuroprotection may relate to its antioxidant capacity. Its neuroprotective effects against hypoxia/reoxygenation injury might be of importance and contribution to their clinical efficacy for the treatment of ischemic brain injury. Our results indicate that salidroside may be a valuable natural therapeutic product for remedying ischemia and respiratory disease.
Acknowledgment
This work is supported by the key disciplinary foundation of Shanghai (03DZ19546).
References
- Abidov M, Crendal F, Grachev S, Seifulla R, Ziegenfuss T (2003): Effect of extracts from Rhodiola Rosea. and Rhodiola crenulata. roots on ATP content in mitochondria of skeletal muscles. Bull Exp Biol Med 6: 585–587.
- Abidov M, Grachev S, Seifulla R, Ziegenfuss T (2004): Extract of Rhodiola Rosea. radix reduces the level of C-reactive protein and creatinine kinase in the blood. Bull Exp Biol Med 7: 63–64.
- Bossenmeyer C (1998): Hypoxia/reoxygenation induces apoptosis through biphasic induction of protein synthesis in cultured rat brain neurons. Brain Res 1: 107–116.
- Brekhman II, Dardymov IV (1969): New substances of plant origin which increase nonspecific resistance. Annu Rev Pharmacol 9: 419–430.
- Cazevieille C, Muller A, Meynier F, Bonne C (1993): Superoxide and nitric oxide cooperation in hypoxia/reoxygenation induced neuron injury. Free Radic Biol Med 14: 389–395.
- Chan PH (1996): Role of oxidants in ischemic brain damage. Stroke 27: 1124–1129.
- Chen HSh, Wu XM, Zhu L (2001): Protective effects of ginkgolid on hypoxic injury of cultured mouse cortical neurons. Acta Acad Med Nantong 21: 226–228.
- Chen JJ, Chen JS, Zhou J (1999): The chemical constituents of Rhodiola atuntsuensis.. Acta Bot Yunn 4: 525–530.
- Dana BJ, Tsuneo F, Randy TR, Shuichi K, David EM (2002): Calcium-dependent activation of Pyk2 hypoxia. Cell Sig 14: 133–137.
- Darbinyan V (2000): Rhodiola rosea. in stress induced fatigue-a double blind cross-over study of standardized extract SHR-5 with repeated low dose regimen on the mental performance of healthy physicians during night duty. Phytomedicine 7: 365–370.
- Faroqui AA, Hau SE, Horrocks LA (1994): In: Basic Neurochemistry: Molecular, Cellular, and Medical Aspects, 5th ed. New York, Raven Press, pp. 867–883.
- German C, Ramazanov Z (1999): Arctic Root (Rhodiola rosea)—The Powerful Ginseng Alternative. New York, Kensington Press, p. 206.
- Hou RC, Huang HM, Tzen J, Jeng KG. (2003): Protective effects of sesamin and sesamolin on hypoxic neuronal and PC12 cells. J Neuro Res 74: 123–133.
- Jang SI, Pae HO, Choi BM (2003): Salidroside from Rhodiola sachalinensis. protects neuronal PC12 cells against cytotoxicity induced by amyloid-β.. Immunopharmacol Immunotoxicol 25: 295–304.
- Kelly GS (2001): Rhodiola rosea.: A possible plant adaptogen. Autern Med Rev 6: 293–302.
- Koo BS, Lee WCh, Chung KH (2004): A water extract of Curcuma longa L.. (Zingiberaceae) rescues PC12 cell death caused by pyrogallol or hypoxia/reoxygenation and attenuates hydrogen peroxide induced injury in PC12 cells. Life Sci 75: 2363–2375.
- Kurkin VA (1986): The chemical composition and pharmacological properties of Rhodiola. plants. J Pharm Chem 10: 1231–1235.
- Li J, Yu HSh (2002): Novel method of preparing high-quality salidroside. J Dalian Insti Light Indus 3: 189–192.
- Machlin LJ, Bendich A (1987): Free radical tissue damage: Protective role of antioxidant nutrient. FASEB J 6: 1441–1446.
- Maslov LN, Lishmanov YB, Maimessulova LA, Krashov EA (1998): A mechanism of antiarrhythmic effect of Rhodiola Rosea.. Bull Exp Biol Med 125: 374–376.
- Ming DS, Hillhouse BJ, Gus ES, Eberding A, Xie S, Vimalanathan S, Towers GHN (2005): Bioactive compounds from Rhodiola Rosea. (Crassulaceae). Phytother Res 19: 740–743.
- Ming HQ, Xia GC, Zhang RD (1988): Advanced research on Rhodiola.. Chin Traditional Herbal Drug 5: 229–234.
- Roberta DS (2004): In vitro. protective effect of Rhodiola rosea. extract against hypochlorous acid-induced oxidative damage in human erythrocytes. Biofactors 20: 147–159.
- Rose CR, Waxma SG, Ranson BR (1998): Effects of glucose deprivation, chemical hypoxia, and simulate ischemia on Na+ homeostasis in rat spinal cord astrocytes. J Neurosci 10: 3554–3562.
- Saratikov AS (1968): Rhodioloside, a new glycoside from Rhodiola rosea. and its pharmacological properties. Die Pharmazie 69: 394–398.
- Wang G, Hazra TK, Mitra S (2000): Mitochondrial DNA damage and a hypoxic response are induced by CoCl2 in rat neuronal PC12 cells. Nucleic Acids Res 10: 2135–2140.
- Wang JY, Shum AY (2002): Hypoxia/reoxygenation induces cell injury via different mechanisms in cultured rat cortical neurons and glial cell. Neurosci Lett 3: 187–191.
- Xue W (2004): Hepatocyte growth factor protects against hypoxia/reoxygenation induced apoptosis in endothelial cells. J Biol Chem 7: 5237–5243.
- Yun JK, McCormick TS, Judware R, Lapetina EG (1997): Cellular adaptive responses to low oxygen tension: Apoptosis and resistance. Neurochem Res 22: 517–521.
- Zhang Zh, Yan Yf, Wei Y (2005): Protective effects of saponin L1 of Radix codonopsis on the apoptosis induced by hypoxia/hypoglycemia and reoxygenation in cultured rat nerve cells. Chin J Basic Med Trad Chin Med 5: 341–344.