Abstract
Solanum aculeastrum. Dunal is a medicinal plant that has long been used to treat various cancers and many other conditions in the Eastern Cape Province of South Africa. In this study, two steroid glycosides were isolated from the berries of this plant, which were identified as tomatidine and solasodine by spectroscopic techniques. Effects on cell growth of these compounds were investigated with HeLa, MCF7, and HT29 cancer cell lines. The IC50 values confirmed that tomatidine and solasodine had the highest inhibitory effect on HeLa cells and the IC50 of the combined compounds was lower than the value for solasodine and unchanged from that of tomatidine. However, the IC50 values of the two compounds combined was also lower in HT29 and MCF7 cells than for the individual compounds. Both tomatidine and solasodine inhibited cell growth by blocking the cell cycle in the G0/G1 phase after 24-h exposure with an increase from 55.6% to 64.2% and 66.8%, respectively. Using annexin V–FITC/PI staining by flow cytometry, the compounds showed very low apoptotic indices.
Introduction
Solanum aculeastrum. Dunal is a medicinal plant that occurs from tropical Africa down to South Africa (Koduru et al., Citation2006d). It is a thorny perennial plant widely distributed in South Africa and grows up to 2–5 m in height. Local healers use the extremely bitter berries for the treatment of various diseases in human beings and domestic animals (Hutchings et al., Citation1996). The fresh and boiled ripe berries are used to treat jigger wounds and gonorrhea (Agnew & Agnew, Citation1994). Previous studies have reported on antimicrobial and antioxidant activities of this plant using crude extracts of its berries (Koduru et al., Citation2006aCitationc). Earlier discussions with traditional healers of the Eastern Cape Province in South Africa revealed that the plant is also used for the treatment of cancer (Koduru et al., Citation2006a). This claim was confirmed in our recent bioassay of its crude extracts on three tumour cell lines (Koduru et al., Citation2006c). In continuation of our research, this study was aimed at isolating the cytotoxic compounds in this plant and investigating their antitumor activity on cultured carcinoma cell lines.
Induction of apoptosis, or programmed cell death, is one approach to cancer therapy (Los et al., Citation2003). Apoptotic cell death is a physiologic mechanism that eliminates unwanted cells by triggering the cell's intrinsic “suicide program” (Kerr et al., Citation1972). Impairment of the apoptotic mechanism ultimately generates a pathologic condition that includes developmental defects such as autoimmune diseases, neurodegeneration, or cancerous neoplasia (Reed et al., Citation2001). Apoptosis is characterized by morphologic changes such as membrane blebbing, cell shrinkage, protein fragmentation, chromatin condensation, and DNA degradation followed by rapid engulfment of cell debris by neighboring cells (Christop, Citation2003). It is therefore possible to take advantage of this intrinsic mechanism by manipulating the apoptotic process for therapeutic gains. Another objective of this study was to broaden the understanding of the relationship between Solanum aculeastrum. and induced apoptosis, which could be beneficial in anticancer therapy.
Materials and Methods
Plant material
The berries of S. aculeastrum. were collected from plants naturally occurring in the wild at Kayalethu village, near the town of Alice, in the Eastern Cape Province of South Africa (latitudes 30°00′–34°15′S and longitudes 22°45′–30°15′E). The plant was identified by Professor DS Grierson at the Department of Botany, University of Fort Hare, and a voucher specimen (Vedic Med 2005/16) was prepared and deposited in the Griffen Herbarium of the university.
Extraction and isolation of tomatidine and solasodine
S. aculeastrum. berries were oven dried to constant weight at 60°C and ground to a powder using a blender. Following the method of Weissenberg (Citation2001), 100 g portion of the powder was added to 300 g toluene plus 200 mL water and 100 mL of 32% HCl and refluxed with stirring for 5 h. The reaction mixture was subsequently alkalinized with 40% aq. NaOH (200 mL) and refluxed again with stirring for 2 h. After phase separation, the upper, pale-yellow toluene layer was siphoned off, and the remaining dark-brown aqueous mixture was further extracted three-times with 100-mL portions of toluene. The purity of the formed precipitate was ascertained by TLC and NMR spectroscopy. The procedure gave consistently colorless and crystalline steroid glycosides with 90–95% recovery (Weissenberg, Citation2001). The white precipitate was separated by preparative TLC using the solvent system CHCl3-EtOAc (9:1), and the compounds were identified using NMR spectroscopy.
NMR spectroscopy
1H and 13C NMR spectra were recorded on a Bruker AMX400 spectrometer (Sarstedt, Numrecht, Germany) at 400 and 100.60 MHz, respectively. Deuterated chloroform was used as the solvent for all the NMR experiments. Chemical shifts are reported in ppm, using TMS as internal references. Preparative TLC–silica gel 60 F254 + 366 was used for preparative thin-layer chromatography (Merck, Darmstadt, Germany) and visualized using sulfuric acid spray (1% H2SO4 in MeOH).
Human carcinoma cell lines and culture medium
HT-29 (colonic adenocarcinoma), HeLa (cervical carcinoma), and MCF-7 (breast adenocarcinoma) cells were cultured in 10-cm culture dishes in growth medium [antibiotic-free RPMI 1640 medium (Sigma, Darmstadt, Germany)] containing 10% heat-inactivated fetal bovine serum (Highveld Biological, Johannesburg, South Africa), 25 mM HEPES, and 2 mM glutamine in a humidified 5% CO2 incubator at 37°C. All cancerous cells were obtained from the American Type Culture Collection (ATCC).
Cytotoxicity assay
For the determination of cell viability, cells were seeded into 96-well culture plates (Nunclon, Roskilde, Denmark) at a density of 6000 cells/well in 200-µL aliquots. Cells were allowed to attach for 24 h in a humidified 5% CO2 incubator at 37°C. Test compounds were solubilized in DMSO before further dilution with growth medium. The final concentration of DMSO in the wells never exceeded 0.25%. Cisplatin (positive control), tomatidine, or solasodine, at concentrations ranging between 0.1 and 1000 µM, were added to test wells whereas no compound was added to the control cells. Cells were exposed to the test compounds for 48 h. Immediately after the 48-h incubation period, cell numbers were determined using the MTT [3-(4,5-dimethylthiazol-2-yl)-2,5-diphenyl tetrazolium bromide] assay as previously described (Alley et al., Citation1988; Brauns et al., Citation2004). Briefly, cells were incubated with 200 µL MTT (Sigma) (0.5 mg/mL in growth medium) for 4 h at 37°C. The formazan product was then dissolved in DMSO, and plates were agitated on a shaker for 5 min, before the absorbance was read at 540 nm on a multiwell scanning spectrophotometer (Multiskan MS; Labsystems). The values obtained were used to determine the percentage inhibition of cell growth caused by the compounds (Hagopian et al., Citation1999; Huq et al., Citation2004).
Annexin V–FITC/PI
HeLa cells were seeded into 10 cm dishes (Sarstedt, Numbrecht, Germany) in growth medium at 1.9 × 106 cells per plate. After a recovery period of 24 h, 2.5 µM cisplatin, 500 µM tomatidine or 500 µM solasodine, or the two alkaloids combined at 100 µM each were added to test plates, whereas no compound was added to the control cells. The cells were incubated for a further 24 h before the Annexin V–FITC Apoptosis Detection Kit (Beckman Coulter, Fullerton, CA, USA) was used to distinguish between apoptotic and necrotic cells. The reagents were prepared as per kit instructions. The cells were collected in ice-cold PBSA and centrifuged for 5 min at 500 × g at 4°C. The supernatant was discarded and the pellet resuspended in ice-cold 1X binding buffer to 2 × 106 cells/mL. The tubes were kept on ice. To 100 µL of the cell suspensions, 1 µL of annexin V–FITC solution and 5 µL of dissolved propidium iodide (PI) were added and mixed gently. The tubes were incubated for 15 min on ice and in the dark before 400 µL of ice-cold 1X binding buffer was added. The samples were analyzed on a Beckman Coulter FC500 flow cytometer. A minimum of 10,000 events were acquired per sample.
Cell cycle analysis
The cell suspensions prepared for annexin V–FITC/PI staining were also used for cell cycle analysis. Cells were fixed and stained using the Coulter DNA Prep Reagents Kit (Beckman Coulter). The cells were analyzed on a Beckman Coulter FC500 Flow Cytometer. A minimum of 10,000 events were acquired for each sample.
Calculations and statistics
Cytotoxicity tests were performed in quadruplicate. Results were expressed as percentage growth inhibition of control, and treatment values were compared with control values using the two-sample Student's t.-test. IC50 values for growth inhibition were derived from a nonlinear regression model (curvefit) based on sigmoidal dose response curve (variable) and computed using GraphPadPrism 4 (Graphpad, San Diego, CA, USA).
Results
Tomatidine and solasodine
The phytochemical analysis of ground berries of S. aculeastrum. afforded two steroid glycosides, tomatidine and solasodine. The structures of the compounds were established with the aid of NMR spectroscopic techniques in addition to comparison with data found in the literature (V'azquez et al., Citation1997; Weissenberg, Citation2001; Wanyonyi et al., Citation2002).
In vitro. antitumor assay
The comparative growth inhibition results () suggest that, at 10 and 100 µM concentrations, tomatidine and solasodine were both most effective against HeLa cervical cancer cells. There were no significant differences between the results obtained with tomatidine and solasodine on any of the three cell lines. The positive control, cisplatin, which is a well-known anticancer agent, consistently performed better than tomatidine and solasodine at the same concentrations (p < 0.001). By combining the two alkaloids in a 1:1 ratio to obtain final concentrations of 10 or 100 µM, the inhibitory effect was higher than the individual effects, especially at 100 µM. It should be noted that the total concentration of the two alkaloids was 10 or 100 µM (). Therefore it appears as if the two compounds had a synergistic effect, at least at the higher concentration of 100 µM (50 µM of each of tomatidine and solasodine).
Figure 1 Comparison of the growth inhibitory effects of two concentrations of tomatidine and solasodine alone and in combination (T + S) with that of cisplatin. HeLa, HT29, and MCF7 cells were exposed to 10 or 100 µM of cisplatin, tomatidine, solasodine, or the latter two combined for 48 h. Mean ± SD (n = 4). **p < 0.001; *p < 0.05 compared with control.
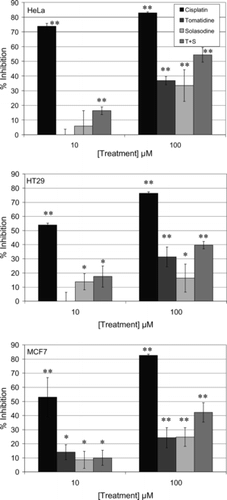
The IC50 values confirmed that tomatidine and solasodine had the greatest inhibitory effect on HeLa cells (). In HeLa cells, the IC50 of the combined alkaloids was lower than the value for solasodine and unchanged from that of tomatidine. The IC50 values of the two compounds combined was also lower in HT29 and MCF7 cells than for the individual compounds, again suggesting synergistic effects.
Table 1.. IC50 values for the test compounds as obtained from log dose response curves using GraphPad Prism.
Cell cycle analysis
To establish whether the alkaloids inhibited cell growth by blocking cells in a certain phase of the cell cycle and/or inducing apoptosis, cellular DNA was stained with PI and cells analyzed using flow cytometry. The sub-G1 phase of the cell cycle was used to calculate the percentage of apoptotic cells (Chang et al., Citation1998). shows that at 24 h exposure to cisplatin, the positive control increased the number of apoptotic cells from 1.7% in control samples to 21.2%. At the same time, there was a delay in the S phase, with an increase from 19.2% to 52.1%. This was expected according to previously published reports (Shapiro & Harper, Citation1999). Tomatidine and solasodine on their own (500 µM) and in a 1:1 combination (100 µM each) caused an increase in the percentage inhibition of cells in the G0/G1 phase, but no increase in apoptosis was observed ().
Table 2.. Results of cell cycle analysis using nuclear PI staining.
Annexin V–FITC assay
To confirm apoptosis, annexin V–FITC/PI staining was done and, again, the results obtained after the flow cytometry showed very low apoptotic indices for the two compounds on their own (). In this assay, however, there was a moderate increase in the apoptotic index when the two alkaloids were both present at 100 µM each. The percentages of cells that stained with PI and, therefore, underwent necrotic death, were higher than the control for all treatments. Cisplatin was the only treatment that gave a higher percentage of apoptotic cells when compared with necrotic cells.
Table 3.. Apoptosis results from annexin V–FITC/PI analysis.
Discussion
S. aculeastrum. grows widely in South Africa and is used for the treatment of many infectious illnesses (Koduru et al., Citation2006a). In recent years, it has been used clinically to treat cancers and has demonstrated the ability to inhibit tumor development (Koduru et al., Citation2006b). However, the mechanism underlying its anticancer effect is not clear. The results of this study have demonstrated that compounds from S. aculeastrum. had significant inhibition on tumor cell growth by blocking certain phase of the cell cycle. Previous studies have shown that solasodine inhibits the growth of Hep3B (Chang et al., Citation1998), HT29, HepG2 (Lee et al., Citation2004), and human 1547 osteosarcoma cells (Trouillas et al., Citation2005). The aglycones tomatidine and solasodine were shown to be less active than their respective glycoalkaloids in inhibiting HT29 and HepG2 cell growth (Lee et al., Citation2004). Contradictory reports were found regarding the induction of apoptosis by solasodine. Chang et al. (Citation1998) found that 11 and 22 µM solamargine, but not its aglycone solasodine, was able to induce apoptosis in HepG2 cells even though they had comparable growth inhibitory properties. Trouillas et al. (2004) showed DNA fragmentation when human 1547 osteosarcoma cells were treated with 40 µM solasodine for 24 h, suggesting apoptosis induction. Our results on HeLa cells confirmed those of Chang et al. (Citation1998), with no evidence of apoptosis, even at the much higher concentration of 500 µM solasodine used in this study. Instead, the cell cycle was blocked in the G0/G1 phase by both tomatidine and solasodine. It is possible that the contradictory results were obtained because of the different cell types that were used in the different studies and that these compounds are only capable of inducing apoptosis in specific cell types. No previous reports were found in the literature about the ability of tomatidine to induce apoptosis; the current study showed no apoptosis induction in HeLa cells despite the block in G0/G1 phase. Friedman et al. (Citation2005) have shown that specific ratios of α.-solanine and α.-chaconine exhibit synergistic anticarcinogenic activities. This study showed for the first time that the two alkaloids, tomatidine and solasodine, have a synergistic effect on growth inhibition. The relatively high percentages of necrotic cells observed with the annexin V–FITC assay can possibly be explained by the observation made by Friedman et al. (Citation2005) that some glycoalkaloids disrupt cell membranes.
Inhibiting tumor growth has been a continuous effort in cancer treatment. A reduction of cell growth and an induction of cell death are two major means to inhibit tumor growth. In this study, we demonstrated that, at low concentrations, some compounds from S. aculeastrum. caused significant inhibition of growth in HeLa, MCF7, and HT29 cells. The inhibitory effect of these compounds on cell growth implies that they may have a general function in antitumor cell growth. This is not unexpected, as cancer cells have developed the capacity of increased proliferation through a variety of growth signal pathways. This includes elevated external growth factors, increased intracellular matrix signal via integrin (Lukashev & Werb, Citation1998), and Ras protein mutation-derived constitutive mitogenic signals (Medema & Bos, Citation1993), resulting in growing neoplasm that causes destruction and atrophy of the surrounding tissue and adjacent organs. In a specific tumor, one pathway may play a more important role than the others. Tomatidine and solasodine may act on more than one pathway. Nevertheless, different sensitivities of tumor cells to the growth inhibitory effect of treatments of the pure compounds were observed. It is worth mentioning that there are several reports on solasodine and tomatidine derivatives possessing pharmacological effects, including significant cytotoxic activity in vitro. against a variety of cancer cell lines (Nakamura et al., Citation1996; Chang et al., Citation1998; Lee et al., Citation2004; Trouillas et al., Citation2005; Koduru et al., Citation2006c). It thus has a great advantage over other types of treatments such as chemotherapy and, more recently, hormonal treatments. S. aculeastrum. contains a variety of compounds that may act on different pathways of tumor cell growth and survival. The molecular mechanisms underlying these effects await further investigation.
Acknowledgments
This research was supported by the National Research Foundation of South Africa. The authors would like to thank Ms. Debbie du Plessis and Ms. A. Afolayan for technical assistance and Prof. J.G.H. du Preez from the Unit for Platinum Group Chemistry, Nelson Mandela Metropolitan University, for providing cisplatin.
References
- Agnew ADQ, Agnew S (1994): Upland Kenya Wild Flowers. A Flora of the Ferns and Herbaceous Flowering Plants of Upland Kenya. Nairobi, East Africa Natural History Society.
- Alley MC, Scudiero DA, Monks A, Hursey ML, Czerwinski MJ, Fine DL, Abbott BJ, Mayo JG, Shoemaker RH, Boyd MR (1988): Feasibility of drug screening with panels of human tumour cell lines using a microculture tetrazolium assay. Cancer Res 48: 589–601.
- Brauns SC, Milne P, Naude R, Van de Venter M (2004): Selected cyclic dipeptides inhibit cancer cell growth and induce apoptosis in HT-29 colon cancer cells. Anticancer Res 24: 1713–1720.
- Chang LC, Tsai TR, Wang JJ, Lin CN, Kuo KW (1998): The rhamnose moiety of solamargine plays a crucial role in triggering cell death by apoptosis. Biochem Biophys Res Commun 242: 21–25.
- Christop B (2003): The Bcl-2 protein family: Sensors check point for life or death decision. Molecular Immunol 39: 615–647.
- Friedman M, Lee KR, Kim HJ, Lee IS, Kozukue N (2005): Anticarcinogenic effects of glycoalkaloids from potatoes against human cervical, liver, lymphoma, and stomach cancer cells. J Agric Food Chem 53: 6162–6169.
- Hagopian GS, Mills GB, Khokhar AR, Bast RC, Siddik ZH Jr (1999): Expression of p53. in cisplatin-resistant ovarian cancer cell lines: Modulation with the novel platinum analogue (1R, 2R.-diaminocyclohexane)(trans.-diacetato) (dichloro)-platinum (IV). Clin Cancer Res 5: 655–663.
- Huq F, Daghriri H, Yu JQ, Tayyem H, Beale P, Zhang M (2004): Synthesis, characterization, activities, cell uptake and DNA binding of [{trans.-PtCl(NH3)2} {µ-(H2N(CH2)6NH2)}{trans.-PdCl(NH3)2](NO3)Cl. Eur J Med Chem 39: 947–958.
- Hutchings A, Scott AH, Lewis G, Cunningham AB (1996): Zulu Medicinal Plants, an Inventory. Pietermaritzburg, University of Natal Press.
- Kerr JFR, Wyllie AH, Currie AR (1972): Apoptosis: A basic biological phenomenon with wide-ranging implications in tissue kinetics. Bri J Cancer 26: 239–257.
- Koduru S, Grierson DS, Afolayan AJ (2006a): Antimicrobial activity of Solanum aculeastrum.. Pharm Biol 44: 283–286.
- Koduru S, Grierson DS, Aderogba MA, Eloff JN, Afolayan AJ (2006b): Antioxidant activity of Solanum aculeastrum. (Solanaceae) berries. Int J Pharmacol 2: 262–264.
- Koduru S, Grierson DS, van de Venter M, Afolayan AJ (2006c): In vitro. antitumour activity of Solanum aculeastrum. berries on three carcinoma cells. Int J Cancer Res (in press).
- Koduru S, Grierson DS, Afolayan AJ (2006d): The foliar micro-morphology of Solanum aculeastrum., a medicinal plant of South Africa. Pakistan J Biol Sci 9: 1056–1060.
- Lee KR, Kozukue N, Han JS, Park JH, Chang EY, Baek EJ, Chang JS, Friedman M (2004): Glycoalkaloids and metabolites inhibit the growth of human colon (HT29) and liver (HepG2) cancer cells. J Agric Food Chem 52: 2832–2839.
- Los M, Burek CJ, Strol C, Bnedyk K, Hug H, Mackiewicz A (2003): Anticancer drugs of tomorrow: Apoptotic pathways as targets for drug design. Drug Discovery Today 8: 67–77.
- Lukashev ME, Werb Z (1998): ECM signalling: Orchestrating cell behaviour and misbehaviour. Trends Cell Biol 8: 437–441.
- Medema RH, Bos JL (1993): The role of p21ras in receptor tyrosine kinase signaling. Critical Rev Oncol 4: 615–661.
- Nakamura T, Komori C, Lee YY, Hashimoto F, Yahara S, Nohara T, Ejima A (1996): Cytotoxic activities of Solanum. steroidal glycosides. Biol Pharm Bull 19: 564–566.
- Reed JC (2001): Apoptosis regulatory proteins as targets for drug discovery. Trends Mol Med 7: 314–318.
- Shapiro GI, Harper JW (1999): Anticancer drug targets: Cell cycle and checkpoint control. J Clin Invest 104: 1645–1653.
- Trouillas P, Corbiere C, Liagre B, Duroux JL, Beneytout JL (2005): Structure-function relationship for saponin effects on cell cycle arrest and apoptosis in the human 1547 osteosarcoma cells: A molecular modelling approach of natural molecules structurally close to diosgenin. Bioorg Med Chem 13: 1141–1149.
- V'azquez A, Gonźalez G, Ferreira F, Moyna P, Kenne L (1997): Glycoalkaloids of Solanum commersonii. Dun. ex Poir. Euphytica 95: 195–201.
- Wanyonyi AW, Sumesh CC, Gerld M, Udo E, Wilson MN (2002): Bioactive steroidal alkaloid glycosides from Solanum aculeastrum.. Phytochemistry 59: 79–84.
- Weissenberg M (2001): Isolation of solasodine and other steroidal alkaloids and sapogenins by direct hydrolysis-extraction of Solanum. plants or glycosides therefrom. Phytochemistry 58: 501–508.