Abstract
In order to evaluate the potential of medicinal plants of Tamil Nadu as sources of antiviral activities, we used seven different viruses to evaluate the methanol extracts of 30 plants, derived from 22 families and recognized for their local medical applications. Antiviral activity was the minimum concentration of extracts required to completely inhibit viral cytopathic effects (CPE), i.e., MIC100 values. Many extracts showed strong activities against Herpes simplex virus (HSV) and mouse corona virus (MCV, the surrogate for human SARS virus). Some extracts were also active against influenza virus and Sindbis virus (SINV, surrogate for hepatitis C virus), but fewer were active against the non-membrane viruses feline calicivirus (FCV, the surrogate for Norovirus), rhinovirus (common cold virus), and poliovirus. The most potent extracts (low MIC100 and broad spectrum of activity) were obtained from Gymnema sylvestre R. Br. (Asclepiadaceae), Pergularia daemia (Forsskal) Chiov. (Asclepiadaceae), Sphaeranthus indicus L. (Asteraceae), Cassia alata L. (Caesalpiniaceae), Evolvulus alsinoides L. (Convolvulaceae), Clitoria ternatea L. (Fabaceae), Indigofera tinctoria L. (Euphorbiaceae), Abutilon indicum G. Don. (Malvaceae), Vitex trifolia L. (Verbenaceae), Clerodendrum inerme (L.) Gaertn (Verbenaceae), and Leucas aspera Spr. (Lamiaceae), which showed anti-MCV and anti-HSV activities at a concentration as low as 0.4 μg/mL. In some cases the activities were enhanced by light, suggesting the presence of photosensitizers. Some of these antiviral activities could contribute to the medicinal properties of the plants, and also provide more support for the concept of scientific validation of traditional plant medicines in the fight against infectious diseases.
Introduction
India has a rich and unique collection of flora, with an estimated 45,000 plant species, among which are numerous species of medicinal plants spread over many different geographical and climatic zones. Many of these species have been used in the traditional medicine systems of Ayurveda, Unani, and others (CitationPal & Jain, 1998).
There is a growing global trend toward the evaluation of medicinal plants for the presence of potentially useful bio-active materials, and this trend has been encouraged by the limitations in uses of synthetic compounds as antibiotics and antivirals, partly because the latter are generally more expensive and less accessible than traditional materials, and also because of the emergence of drug-resistant microbes. The antimicrobial properties of certain Indian medicinal plants have been reported (CitationRamchandra et al., 1993; CitationValsaraj et al., 1997; CitationPerumal Samy et al., 1998; CitationMehmood et al., 1999; CitationPerumal Samy & Ignacimuthu, 2000; CitationVaijayanthimala et al., 2000; CitationSrinivasan et al., 2001; CitationVonshak et al., 2003; CitationRajesh Dabur et al., 2004).
A few Indian medicinal plants have also been examined for antiviral activities (CitationSubba Rao et al., 1974), but little work has been reported on the plants of Tamil Nadu, in spite of their common uses by many tribal groups throughout the region for the treatment of various diseases, including possible virus infections (CitationJain, 1994; CitationKamboj, 2000; CitationVedapathy, 2003).
We therefore decided to examine extracts of 30 different species of medicinal plant known for their uses in Tamil Nadu in the treatment of diseases. We used techniques developed in our laboratory and others over the last 20 years for the evaluation of significant antiviral activities in crude extracts (CitationHudson, 1990; CitationVlietinck & Vanden Berghe, 1991; CitationHudson, 1995; CitationTaylor et al., 1995). These techniques allow for flexibility and also permit detection of photo-active compounds, which are common in some families of medicinal plants (CitationTowers et al., 1997; CitationHudson & Towers, 1999), and which could conceivably contribute to the efficacy of medicinal plant preparations, which are often administered in the presence of sunlight in tropical countries.
Materials and methods
Plants and extracts
The plants were collected during different seasons in 2002-2003 from the hills in and around Papanasam village, Tirunelveli district, following the method of CitationJain (1964). The medicinal plants were identified, photographed, and sample specimens were collected for the preparation of a herbarium. Authentication of the plants was carried out by M. Ayyanar, plant taxonomist at the Entomology Research Institute, Loyola College, Chennai. The specimens were deposited in the herbarium of Entomology Research Institute, Loyola College, Chennai (India). The tribal information (CitationAyyanar & Ignacimuthu, 2005; CitationIgnacimuthu et al., 1998) is also kept in the same institute.
Plant materials were air-dried and ground in a Wiley grinder with a 2 mm wire mesh. A 20 g sample of each powder was soaked in 500 mL of methanol (MeOH) for a minimum of 24 h. The sample was then suction filtered through Whatman No. 1 filter paper, and washed with another 500 mL of methanol. The filtrate was evaporated to near dryness under reduced pressure. Dried extract (100 mg) was re-dissolved in 1 mL of methanol.
Cells and viruses
Cell lines
Vero (monkey kidney cell line), H-1 (a sub-clone of HeLa cells which is particularly sensitive to rhinovirus replication), DBT (mouse delayed brain tumor cells) and MDCK (Madin-Darby canine kidney cells), were obtained from the American Type Culture Collection (ATCC). The Crandell feline kidney cell line was obtained from Martin Petric, BC Centre for Disease Control, Vancouver. All cells were propagated in Dulbecco MEM with 5-10% fetal bovine serum, without antibiotics or anti-fungal agents. Herpes simplex virus type 1 (HSV), and polio virus were assayed in Vero cells. Influenza virus (FLU) was propagated and assayed in MDCK cells. Rhinovirus (RV) type 14 was propagated and assayed in H-1 cells at 34°C. Mouse corona virus (MCV) was propagated and assayed in DBT cells, and feline calicivirus (FCV) was propagated and assayed in feline kidney cells.
Antiviral assays
The methods were developed on the basis of principles explained in CitationVanden Berghe et al. (1986) and CitationHudson (1990). Two different protocols were used in the present study.
In the “virucidal protocol”, cell monolayers were grown in 96-well microtest trays (Falcon), 0.2 mL per well (CitationAnani et al., 2000). When the cells formed confluent monolayers, they were used for the assays. A solution of 1000 μg/mL of each methanol extract was prepared in DMEM and filtered through a sterile syringe filter 0.2 μm pore diameter, from which serial 2-fold dilutions were made in DMEM (in duplicate) across a row of wells in an empty 96-well microtest tray. Then, 0.1 mL of virus (HSV, SINV, polio, RV, influenza virus, FCV or MCV), comprising 100 pfu (plaque forming units) in DMEM, was added to each well (except for the controls, which received medium or medium plus solvent only). The diluted extracts were transferred to the aspirated cell monolayers.
The trays containing these virus extract mixtures were transferred to an environmental chamber (37°C) and exposed to a combination of visible light plus UVA (long-wave ultraviolet light) for 30 min, with continuous gentle shaking of the tray. The lamps were arranged to give approximately 2 W/m2 incident radiation of both visible and UVA. Following the incubations, the contents of each well were transferred by means of multi-pipettors to aspirated wells containing cells only, and returned to the incubator to allow development of characteristic viral cytopathic effects (CPE). MIC (minimal inhibitory concentration) was recorded as the end-point at which viral CPE appeared, i.e., virus was not completely inactivated (CitationHudson, 1990). Controls included cells with no virus, cells infected with untreated virus, and in some cases trays were covered with aluminum foil during incubations to exclude light (“dark” antiviral activity).
In the “pre-exposure” protocol, diluted extracts were prepared in an empty 96-well tray as above. They were then transferred to aspirated cell monolayers and incubated as above with light exposure. Then the virus, 100 pfu in 0.1 mL was added to each well (except the controls), and the trays were immediately returned to the incubator.
All cultures were examined periodically under the microscope for viral CPE. The time of incubation was characteristic for each virus, and the examinations were terminated when viral CPE in the untreated-virus controls reached 100% (i.e., all cells showed CPE). The end-point was the highest dilution of extract giving complete elimination of viral CPE, i.e., 100% killing of the virus inoculum. This allowed us to calculate MIC (minimum inhibitory concentration) in μg/mL. Generally, duplicate samples gave identical end points. In cases where there were two-fold differences between duplicates, then simple arithmetic means were calculated. However, in all cases experiments were replicated, with identical results.
In additional experiments, virus plaque assays were used to corroborate the results of the end-point methods.
Antiviral photosensitizers
To determine the presence of photosensitizers, the virucidal test protocol was used. The light exposure was omitted (dark anti-viral activity) in half the test trays by wrapping them in aluminum foil. These trays were otherwise treated identically.
Cytotoxicity assay
To test for cytotoxicity, cell monolayers were grown in 96-well microtiter plates, and exposed to serial dilutions of the extracts, starting at 1000 μg/mL crude plant extract. The treated cells were then incubated at 37°C for 1 h, exposed to UVA light and visible light for 30 min and then re-incubated for 24 h. The cells were examined microscopically for periodic assessment of changes in cell morphology or visible toxic effects (obvious cellular damage or lysis).
Results
lists the botanical names of the plants from which extracts were made, the plant parts used, and the traditional uses of the plant in Tamil Nadu. Evidently many of the plants were used for treatment of diseases, including infections, and some of them might therefore contain antiviral activities.
Table 1. Relevant characteristics of the species used in this study.
Antiviral activity: Virucidal protocol
This technique measures direct antiviral (i.e., virus-killing) activity of the extracts, and has proven in the past to be very efficient in highlighting plant materials with significant antiviral activity (CitationHudson, 1990; CitationTaylor et al., 1995). The extracts were subjected to two-fold dilutions and evaluated for their ability to completely inactivate 100 infectious virus particles, as indicated by neutralization of the characteristic viral cytopathic effects in cell culture. Results were expressed as minimum inhibitory concentration (MIC). In the reciprocals of the MIC values are plotted, so that the higher the bars the greater the antiviral activity.
Figure 1. Anti-HSV plant extracts (virucidal protocol). Results for each of the indicated species are plotted as reciprocals of the MIC (minimum inhibitory concentration) in μg/mL. The higher the bar, the greater the antiviral activity. Thus a value of 2.0 represents an MIC of 0.5 μg/mL (or less in some cases) other plant species showed little or no activity.
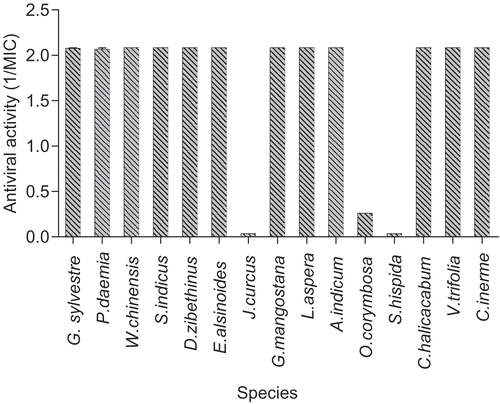
Figure 2. Anti-coronavirus (MCV) extracts (virucidal protocol). Results for each of the indicated species are plotted as reciprocals of the MIC (minimum inhibitory concentration) in μg/mL. The higher the bar, the greater the antiviral activity. Thus a value of 2.0 represents a MIC of 0.5 μg/mL (or less in some cases) other plant species showed no activity.
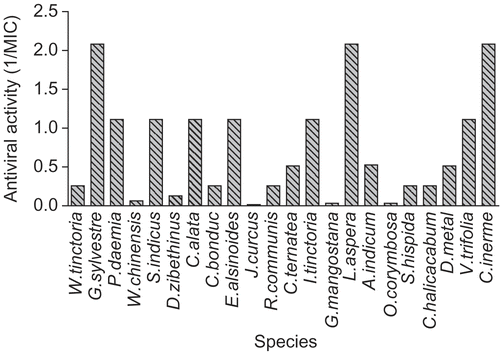
Figure 3. Anti-calicivirus (FCV) extracts (virucidal protocol). Results for each of the indicated species are plotted as reciprocals of the MIC (minimum inhibitory concentration) in μg/mL. The higher the bar, the greater the antiviral activity. Other plant species showed no activity.
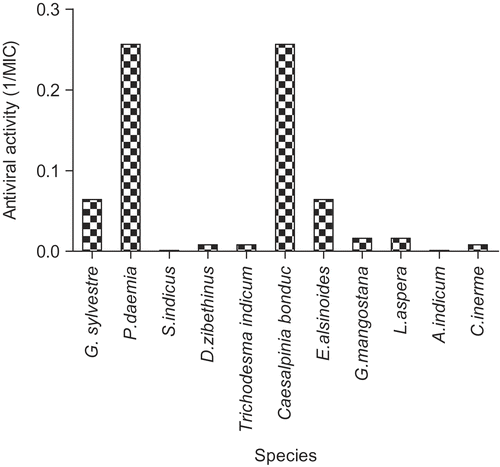
Figure 4. Antiviral activities (pre-exposure protocol). Results for each of the indicated species are plotted as reciprocals of the MIC (minimum inhibitory concentration) in μg/mL. The higher the bar, the greater the antiviral activity. Other plant species showed no activity.
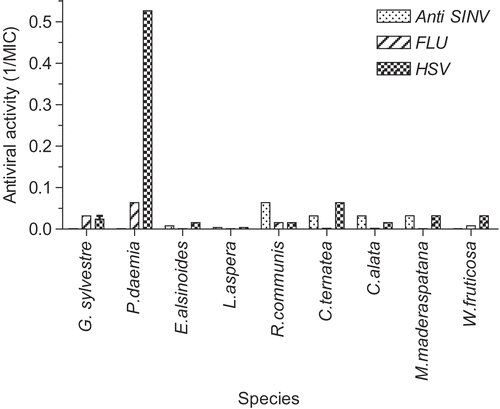
Figure 5. Antiviral photosensitizers (virucidal protocol). MIC values were measured against HSV and MCV (virucidal protocol) both in light and in dark (details in Materials and methods section), and the results plotted as reciprocals as in . All extracts except O. corymbosa and G. mangostana showed marked light-enhanced or light-dependent activity typical of photosensitizers.
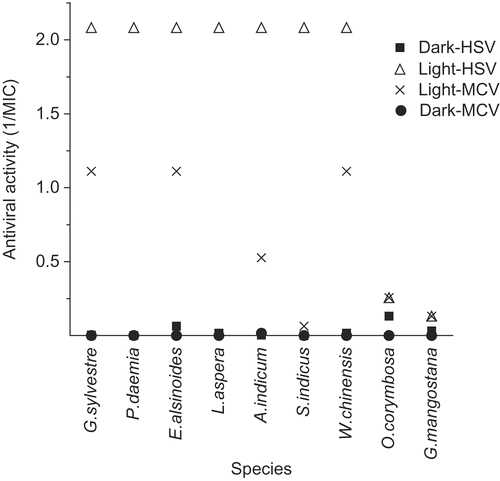
The antiviral activities varied considerably between extracts, and between different target viruses. The most active species are shown in and , for the membrane-containing viruses Herpes simplex virus (HSV) and mouse coronavirus (MCV), respectively. Error bars on the graphs are too small to be visible in most cases. Twelve of the extracts were still active down to concentrations of less than 0.5 μg/mL. The others were moderately active (MIC ~ 7.8 to 62 μg/mL), or had little or no activity (MIC > 62 μg/mL). Seven of these highly active extracts, Gymnema sylvestre R. Br. (Asclepiadaceae), Pergularia daemia (Forsskal) Chiov. (Asclepiadaceae), Sphaeranthus indicus L. (Asteraceae), Evolvulus alsinoides L. (Convolvulaceae), Leucas aspera Spr. (Lamiaceae), Vitex trifolia L. (Verbenaceae), and Clerodendrum inerme (L.) Gaertn (Verbenaceae) were also the most active against MCV. In contrast, Cassia alata L. (Caesalpiniaceae) was active against MCV, but not HSV.
shows the corresponding results for activity against the non-membrane virus FCV (feline calicivirus). In this case, only two extracts had antiviral activity, P. daemia, and Caesalpinia bonduc (L.) Roxb. (Caesalpiniaceae) and several others showed weak activity. Antiviral activity against rhinovirus was confined only to G. sylvestre, S. indicus, and V. trifolia and was relatively moderate to weak (data not shown). Only two extracts, Rhinacanthus communis Nees. (Acanthacea) (MIC = 15.6 μg/mL) and Woodfordia fructicosa Kurz. (Lythraceae) (MIC = 250 μg/mL), displayed antiviral activity against polio virus (data not shown).
Antiviral activity: Pre-exposure protocol
In this test system, cells are exposed to the diluted extracts for one hour before the addition of virus, and left on during incubation in order for viral cytopathic effects to develop. Extracts that possess virus replication-inhibition properties would show up in this protocol, in addition to virucidal properties.
In all cases, the relative activities against HSV and MCV were substantially lower than in the virucidal tests, suggesting that the active extracts in and were targeting the viruses directly, rather than their replication.
shows activity against two additional membrane-containing viruses, influenza virus and Sindbis virus. Several of the very active extracts were also active against these two viruses.
Antiviral photosensitizers
We have reported previously that many antiviral plant extracts contain photosensitizers, compounds whose activity is either dependent on, or is enhanced significantly by, exposure to light, especially those wavelengths found in sunlight, i.e., UVA (long-wave ultraviolet) and visible range (CitationTowers et al., 1997; CitationHudson & Towers, 1999).
shows the relative MIC values in light and dark (plotted as reciprocals as before), for the most active anti-HSV and anti-MCV activities. The ratios for Garcinia mangostana L. (Clusiaceae) and Oldenlandia corymbosa L. (Rubiaceae) were within the normal range for light independent activities (1.9 to 4.0). But the other extracts showed much higher light/dark ratios, indicative of the presence of antiviral photosensitizers.
Cytotoxicity
All extracts were evaluated for cytotoxic activity against all four cell lines used in the study. However, most of the permutations showed no microscopically visible cellular damage, the exceptions being P. daemia, G. mangostana, and Abutilon indicum G. Don. (Malvaceae), all of which showed some effect in Vero cells only, and these were not sufficient to interfere in the antiviral assays.
Discussion
We employed two different methods to test for antiviral activity in the extracts, as well as confirmatory plaque assays for some samples. In the so-called virucidal protocol, the virus was incubated with the extract for one hour before adding the mixture to the cells. In the pre-exposure protocol, the cells were exposed to extract for one hour before the addition of the standard virus inoculum. By means of this combination of methods, we should be able to detect antiviral compounds that work by a variety of different mechanisms, including direct inactivation of the virus particles, inhibition of attachment of virus to cellular receptors, and any intracellular stage involved in virus replication and egress from the cell (CitationVanden Berghe et al., 1986; CitationHudson, 1990). In addition, we tested some active extracts for the presence of antiviral photosensitizers, which are often found in certain families of medicinal plants (CitationTowers et al., 1997; CitationHudson & Towers, 1999).
indicates the frequency of active extracts for each of the target viruses, i.e., the number of potentially useful “hits”. Every extract contained antiviral activity to a certain degree, although some of these activities were weak and specific for only one or two viruses. However, the following species contained impressive activity (less than 1 μg/mL) against several viruses: G. sylvestre, P. daemia, Clitoria ternatea L. (Fabaceae), L. aspera, C. alata, E. alsinoides, V. trifolia, and C. inerme. In addition, Durio zibethinus L. (Bombacaceae), Trichodesma indicum R. Br. (Boraginaceae), G. mangostana, O. corymbosa, Spermacoce hispida L. (Rubiaceae), and C. bonduc showed moderate activity against more than one virus.
In most of these cases we were able to evaluate the extracts in both antiviral test protocols, and in all cases, the virucidal activity was substantially greater, which implies the active components interacted directly with the virus. In common with our previous experience, we found that the membrane-containing viruses, with either DNA or RNA genomes were more susceptible than the non-membrane viruses, suggesting that the primary targets for the active compounds were lipids or lipoprotein components. The MCV is often used as a surrogate test virus for the human corona viruses such as SARS virus. The vulnerability of this virus and influenza virus suggests that these very active plant species could be useful in controlling respiratory infections. Herpes simplex virus (representative of skin herpes viruses) was also very vulnerable. However, the relative resistance of the rhinovirus (common colds), FCV (surrogate for Norovirus) and poliovirus suggests that they might be less efficacious against gastro-intestinal infections.
The majority, but not all, of the very active species showed the presence of light-dependent or light-enhanced components. We have reported previously that medicinal plants of several families contain photosensitizers with anti-microbial and antiviral activities, such as thiophenes and polyacetylenes from the Asteraceae, complex anthraquinones from the Guttifereae, furanocoumarins and related compounds from the Rutaceae and Apiaceae (CitationTowers et al., 1997; CitationHudson & Towers, 1999). Some of these compounds have been used as leads to the development of useful anticancer compounds, and other useful properties, based on their selective activation by light. The fact that these species tested in this study are tropical plants suggests that some of their applications could incorporate sunlight. This could apply for example to Wedelia chinensis (Osbeck) Merr. and S. indicus, both members of the Asteraceae.
In addition, the presence of photoactive compounds narrows the focus of attention on the nature of the active compounds. Presumably one can rule out the likelihood that the active compounds are known plant polysaccharides, proteins and lipids, and consequently we should focus on the secondary metabolites of these plants.
Cytotoxic activities among these plant extracts were rare, and consequently they may serve as the source of useful and safe antiviral materials. This study adds more support to the concept that traditional medicinal plants can be potentially useful in the fight against infectious diseases.
Declaration of interest: The authors report no conflicts of interest. The authors alone are responsible for the content and writing of the paper.
References
- Anani K, Hudson JB, de Souza C, Akpagana K, Tower GHN, Arnason JT, Gbeassor M (2000): Investigation of medicinal plants of Togo for antiviral and antimicrobial activities. Pharm Biol 38: 40–45.
- Ayyanar M, Ignacimuthu S (2005): Traditional knowledge of Kani tribals in Kouthalai of Tirunelveli hills, Tamil Nadu, India. J Ethnopharmacol 102: 246–255.
- Hudson JB, Towers GHN (1999): Phytomedicines as antivirals. Drugs of the Future 24: 295–320.
- Hudson JB (1995): The phytochemical approach to antiviral chemotherapy. In: Chessin M, eds., Antiviral Proteins in Higher Plants: Boca Raton, FL, CRC Press, pp. 161–174.
- Hudson JB (1990): Antiviral Compounds from Plants. Plant Extracts. Boca Raton, CRC Press, pp. 179–192.
- Ignacimuthu S, Sankarasivaraman K, Kesavan L (1998): Medico-ethnobotanical survey among Kanikar tribals of Mundanthurai Sanctuary. Fitoterapia 69: 409–414.
- Jain SK (1994): Ethnobotany and research on medicinal plants in India. Ciba Found Symp 185: 153–64; discussion 164-168.
- Jain SK (1964): The role of botanist in folklore research. Folklore 5: 145–150.
- Kamboj VP (2000): Herbal medicine. Curr Sci 78: 35–39.
- Mehmood Z, Ahmad I, Mohammad F, Ahmad S (1999): Indian medicinal plants a potential source for anticandidal drugs. Pharm Biol 37: 237–242.
- Pal DC, Jain SK (1998): Tribal Medicine. Naya Prokash, 206, Bidhan Sarani, Calcutta, India, p. 316.
- Perumal Samy R, Ignacimuthu S (2000): Antibacterial activity of some folklore medicinal plants used by tribals in Western Ghats of India. J Ethnopharmacol 69: 63–71.
- Perumal Samy R, Ignacimuthu S, Sen A (1998): Screening of 34 Indian medicinal plants for antibacterial properties. J Ethnopharmacol 62: 173–181.
- Rajesh Dabur, Singh H, Chhillar AK, Ali M, Sharma GL (2004): Antifungal potential of Indian medicinal plants. Fitoterapia 75: 389–391.
- Ramchandra P, Basheermiya M, Krupadanam GLD, Srimannarayana G (1993): Wrightial, a new terpene from Wrightia tinctoria. J Nat Prod 56: 1811–1812.
- Srinivasan D, Nathan S, Suresh T, Perumalsamy PL (2001): Antimicrobial activity of certain Indian medicinal plants used in folkloric medicine. J Ethnopharmacol 74: 217–220.
- Subba Rao G, Sinsheimer JE, Cochran KW (1974): Antiviral activity of triterpenoid saponins containing acylated β-amyrin aglycones. J Pharm Sci 63: 471–473.
- Taylor RS, Manandhar NP, Towers GHN (1995): Screening of selected medicinal plants of Nepal for antimicrobial activities. J Ethnopharmacol 46: 153–159.
- Towers GHN, Page JE, Hudson JB (1997): Light-mediated biological activities of natural products from plants and fungi. Curr Org Chem 1: 395–414.
- Vaijayanthimala J, Anandi C, Udhaya V, Pugalendi KV (2000): Anticandidal activity of certain South Indian medicinal plants. Phytother Res 14: 207–209.
- Valsaraj R, Pushpangadan P, Smitt UW, Adsersen A, Nyman U (1997): Antimicrobial screening of selected medicinal plants from India. J Ethnopharmacol 58: 75–83.
- Vanden Berghe DA, Vlietinck AJ, Van Hoof L (1986): Plant products as potential antiviral agents. Bull Inst Pasteur 84: 101–147.
- Vedapathy S (2003): Scope and importance of Traditional medicine. Indian J Trad Know 2: 236–239.
- Vlietinck AJ, Vanden Berghe DA (1991): Can ethnopharmacology contribute to the development of antiviral drugs? J Ethnopharmacol 32: 141–153.
- Vonshak A, Barazani O, Sathiyamoorthy P, Shalev R, Vardy D, Golan-Goldhirsh A (2003): Screening South Indian medicinal plants for antifungal activity against cutaneous pathogens. Phytother Res 17: 1123–1125.