Abstract
Numerous preparations of Echinacea (Asteraceae), mainly derived from three species, Echinacea purpurea ( L.) Moench, Echinacea angustifolia (D.C.) and Echinacea pallida (Nutt.) Nutt., are available for the treatment of symptoms of colds and influenza which, along with a number of other respiratory conditions, have been ascribed to the induction of pro-inflammatory cytokines. We evaluated various chemically characterized extracts and fractions, derived from the three species of Echinacea, for their possible inhibitory effects on the secretion of pro-inflammatory cytokines IL-6 and IL-8 (CXCL-8) by human bronchial epithelial cells infected with rhinovirus type 14. All of the E. purpurea fractions, comprising aqueous or ethanol extracts of roots, leaves and stems, but to a lesser degree flowers, strongly inhibited the secretion of both cytokines. In contrast, corresponding fractions derived from E. angustifolia and E. pallida showed relatively weak cytokine-inhibitory activity, whereas their aqueous fractions significantly enhanced cytokine secretion, both in virus-infected and in uninfected cells. These properties did not correlate with the presence or absence of alkylamides or specific caffeic acid derivatives, although the alkylamide rich fraction of E. angustifolia showed a significant inhibitory effect. However, there was some correlation between anti-cytokine effects and our previously reported anti-viral activities. Thus, none of the groups of compounds traditionally used as “markers” for Echinacea are responsible for anti-inflammatory activity. Consequently, we believe that other constituents of Echinacea should be evaluated.
Introduction
Different species and parts of Echinacea (Asteraceae) have been used traditionally in North America for the treatment of symptoms of upper respiratory infections such as colds and influenza, as well as inflammatory conditions (CitationBarrett, 2003; CitationBarnes et al., 2005). A number of Echinacea marker compounds have been characterized, including polysaccharides, caffeic acid derivatives (CADs) and alkylamides (CitationBauer, 1998; CitationBinns et al., 2002), and these have all been shown to possess infection-related bio-activities in various tests in vitro and in vivo, including immune-modulating activities, anti-viral activities, and antibiotic activities (CitationBurger et al., 1997; CitationBauer, 1998; CitationRininger et al., 2000; CitationGoel et al., 2002; CitationMerali et al., 2003; CitationGertsch et al., 2004; CitationVimalanathan et al., 2005; CitationHudson et al., 2005; CitationRaduner et al., 2006; Sharma et al., 2006; CitationLaLone et al., 2007).
Rhinoviruses have been implicated as major players in common colds and various types of allergic rhinitis and bronchial syndromes (CitationMessage & Johnston, 2004; CitationSchaller et al., 2006). However, numerous studies have shown that rhinovirus infection in cultured epithelial cells, and in nasal epithelial tissues in vivo, results in relatively low levels of virus replication and cytopathology, apparently due to the small number of cells supporting virus replication (CitationMosser et al., 2005). In contrast, there is substantial induction of secretion of certain pro-inflammatory cytokines and chemokines, including the important multifunctional cytokines implicated in various inflammatory conditions, interleukin-6 (IL-6) and interleukin-8, CXCL-8 (IL-8) (CitationMessage & Johnston, 2004; CitationSharma et al., 2006; CitationSchaller et al., 2006; CitationEdwards et al., 2007). Thus, the typical symptoms of a common cold, such as sneezing, coughing, runny nose, stuffed nasal passages and others are not the direct result of viral pathology, but rather the indirect stimulation of pro- inflammatory cytokines and chemokines. Consequently, successful treatment of colds and influenza might be obtained by appropriate use of an anti-inflammatory material.
Certain formulations of Echinacea could fulfill this role. However, there are hundreds of Echinacea products in the market, mostly prepared from aerial parts of E. purpurea or from roots of E. purpurea (L) Moench, E. angustifolia (D.C.) and E. pallida (Nutt.) Nutt (CitationBarrett, 2003; CitationBarnes et al., 2005). They are also available as tinctures, teas, and powders in capsules. However, their chemical composition is significantly different (CitationBauer, 1998; CitationBinns et al., 2002).
We previously evaluated aqueous and ethanol fractions, and various solvent sub-fractions, derived from the three species, for antiviral activities, which we found in many of the fractions, although there was no apparent correlation with the presence or composition of the principal marker compounds, the alkylamides, polysaccharides and caffeic acid derivatives (CitationVimalanathan et al., 2005; CitationHudson et al., 2005).
In this study, we investigated the same kinds of extract for their possible immune modulation effects, by measuring their effects on rhinovirus-induced secretion of the key pro-inflammatory cytokines IL-6 and IL-8, in human bronchial epithelial cells.
Materials and methods
Echinacea sources
Echinacea purpurea (L.) Moench, E. angustifolia (D.C.) and E. pallida (Nutt.) Nutt. (voucher numbers, UO #010502-18; UO #010410-12; UO #010410-11, respectively) were grown by a commercial grower in North America. Species were identified by Shannon Binns, University of Ottawa.
Echinacea extracts and fractions
Complete extraction details were described previously (CitationVimalanathan et al., 2005; CitationHudson et al., 2005). Briefly, 10 g of the root materials were extracted exhaustively at 40°C with an accelerated solvent extractor (Dionex, ASE100) with either distilled water or 70% ethanol and extracts brought to a final volume of 50 mL. The 70% ethanol fractions were sequentially extracted three times in a separatory funnel with equal volumes of water and hexane to produce a combined hexane extract. The hydroalcoholic extract was extracted twice with ethyl acetate to produce an ethyl acetate extract, and an aqueous residue as described in CitationHudson et al. (2005) (all adjusted to 5 mL/g root). Analysis of the extracts was performed by reverse phase HPLC/DAD on a 7. 5 cm reverse phase C18 column (3 μm particle size) using acetonile:water (6:1) eluted at 1 mL/min (Agilent 1100 HPLC) with detection at 210 and 260 nm (CitationBinns et al., 2002).
Cells and viruses
BEAS-2B human epithelial cells, originally obtained from ATCC, were grown in Dulbecco MEM (DMEM) in 10% fetal bovine serum. For the experiments, cells were sub-cultured in 6-well trays, and when confluent the medium was changed to DMEM without serum. The H-1 sub-clone of HeLa cells American Type Culture Collection (ATCC) was cultivated in the same medium. No antibiotics or antimycotic agents were used.
Rhinovirus type 14 (RV 14, from ATCC), was propagated and assayed, by plaque assay (CitationHudson et al., 1982), in H-1 cells. The stock virus had a titer of 2 × 107 pfu/mL.
Experimental system
Details of the test system were described previously (CitationSharma et al., 2006). BEAS-2B cells were grown in complete medium, in 6-well trays, to produce confluent monolayers. The medium was then replaced with serum free DMEM for the experiments. Virus was added to the cells at 1 infectious virus per cell (1 pfu/cell), for one hour at 35°C, followed by a dilution of the Echinacea fraction to yield a final concentration of 250 μg/mL. Culture supernatants were harvested after 48 h, which we determined to be the optimum time of cytokine secretion, and assayed for IL-6 and IL-8. Controls included cells with no virus and cells (+/- virus) with equivalent amounts of solvent only.
All cultures were in duplicate and each supernatant was assayed in duplicate. All data presented are from individual experiments, but all experiments were repeated at least once, with consistent results.
ELISA assays were carried out with commercial kits, according to the instructions supplied by the companies (Immunotools, Friesoythe, Germany, and e-Bioscience, San Diego, USA).
Results
shows the anti-inflammatory effects of the different Echinacea purpurea (L.) Moench root fractions. In this culture system the levels of IL-8 secreted were usually several-fold more than IL-6. The virus itself stimulated the secretion of both pro-inflammatory cytokines substantially, but all the fractions significantly reduced these levels, particularly the aqueous extracts. They had little effect, however, on the low control levels (in uninfected cells) of cytokine secretion. This anti-inflammatory effect was clearly not influenced by the concentrations of caffeic acid derivatives, which varied widely between the fractions (see tabulated data beneath ), and furthermore did not require alkylamides, which were absent in these fractions.
Figure 1. Anti-cytokine effects of E. purpurea root extracts. BEAS-2B cells were infected with rhinovirus type 14 (1 pfu/cell) for 60 min at 35°C, followed by 250 μg/mL of the indicated fraction of E. purpurea root extract. Controls received no virus. Solvent controls showed no effect. After 48 h, cell supernatants were removed for assay of IL-6 and IL-8 by standard ELISA tests. Standard curves were constructed for each experiment and the absorbance readings at 450 nm were converted into pg/mL. Composition of marker compounds is shown below the figure.
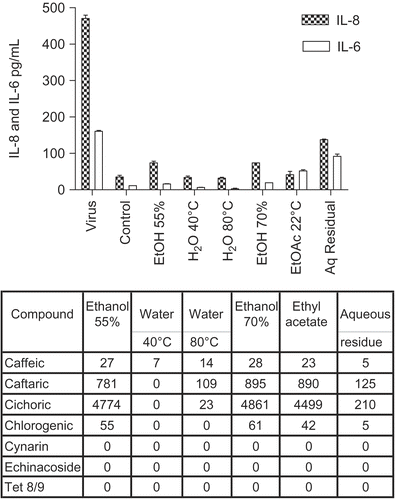
shows the IL-8 results for cultures treated with E. purpurea leaf and stem combined extracts (essentially the same results were obtained for IL-6, not shown). In this case also, there was a consistent and marked inhibition in cytokine release, including the control levels. Thus the results were very similar to the root extracts shown in , although the leaf plus stem fractions showed greater inhibitory activity than root fractions, since all fractions were evaluated at the same concentration. Again, these impressive anti- inflammatory effects did not correlate with specific CADs, and occurred in the absence of alkylamides.
Figure 2. Anti-cytokine effects of E. purpurea leaf plus stem extracts. BEAS-2B cells were infected with rhinovirus type 14 (1 pfu/cell) for 60 min at 35°C, followed by 250 μg/mL of the indicated fraction of E. purpurea extract. Controls received no virus. After 48 h, cell supernatants were removed for assay of IL-6 and IL-8 by standard ELISA tests. Standard curves were constructed for each experiment and the absorbance readings at 450 nm were converted into pg/mL. Not all solvent fractions were available for these tests. Only IL-8 is shown in this graph. Similar results were obtained for IL-6. Composition of marker compounds is shown below the figure.
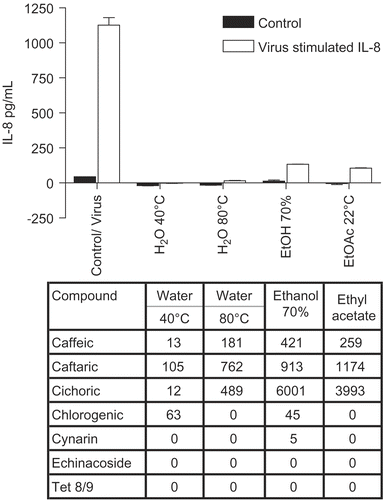
E. purpurea flower extracts showed the same pattern ( for IL-8; similar results were obtained for IL-6, not shown), except that in this case the degree of inhibition was uniformly much less. To determine if polysaccharides could play a role in these effects, we tested polysaccharide enriched and purified AGP (arabinogalactan fraction) for effects on cytokine secretion (). The starting crude extract, the E. purpurea pressed juice extract, showed the usual inhibitory effect, whereas the polysaccharide enriched fraction ( > 100 kDa mol. wt. polysaccharides derived from the juice; CitationVimalanathan et al., 2005) was much less inhibitory. Furthermore the arabinoglactan fraction (AGP), derived by SEC column chromatography of the previous polysaccharide fraction, showed no inhibition. In addition none of these fractions had a significant effect on the control level of cytokine secretion.
Figure 3. Anti-cytokine effects of E. purpurea flower extracts. BEAS-2B cells were infected with rhinovirus type 14 (1 pfu/cell) for 60 min at 35°C, followed by 250 μg/mL of the indicated fraction of E. purpurea flower extract. Controls received no virus. After 48 h, cell supernatants were removed for assay of IL-6 and IL-8 by standard ELISA tests. Standard curves were constructed for each experiment and the absorbance readings at 450 nm were converted into pg/mL. Only IL-8 is shown in this graph; similar results were obtained for IL-6. Composition of marker compounds is shown below the figure.
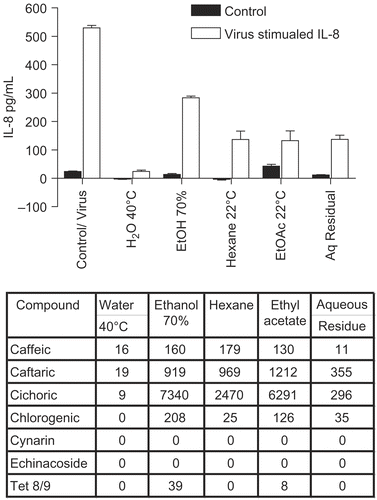
Figure 4. Anti-cytokine effects of polysaccharide-enriched fractions. BEAS-2B cells were infected with rhinovirus type 14 (1 pfu/cell) for 60 min at 35°C, followed by 250 μg/mL of the indicated fraction derived from E. purpurea aqueous pressed juice. Controls received no virus. After 48 h, cell supernatants were removed for assay of IL-6 and IL-8 by standard ELISA tests. Standard curves were constructed for each experiment and the absorbance readings at 450 nm were converted into pg/mL. Polysaccharide-enriched fraction, P50 fraction (polysaccharides > 100 kDa) isolated from pressed juice; AGP fraction, arabinogalactan isolated by SEC 1 column from the polysaccharide fraction.
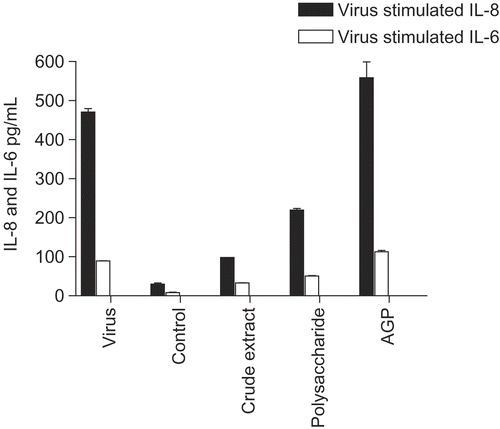
The corresponding results for E. angustifolia and E. pallida root fractions were quite different from E. purpurea ( for E. angustifolia and for E. pallida). Data are shown for IL-8 only, although IL-6 gave similar results. Only the ethanol fraction of E. angustifolia was inhibitory (anti-inflammatory), although to a much smaller degree than the corresponding E. purpurea root fractions. However, the aqueous fractions had no inhibitory activity, and in fact some of them induced even higher levels of cytokine secretion, in the case of E. pallida by 2-3-fold. In other words, E. pallida aqueous fractions enhanced the pro-inflammatory response, in spite of the complete absence of CADs and alkylamides.
Figure 5. Anti-cytokine effects of E. angustifolia root extracts. BEAS-2B cells were infected with rhinovirus type 14 (1 pfu/cell) for 60 min at 35°C, followed by 250 μg/mL of the indicated fraction of E. angustifolia root extract. Controls received no virus. After 48 h, cell supernatants were removed for assay of IL-6 and IL-8 by standard ELISA tests. Standard curves were constructed for each experiment and the absorbance readings at 450 nm were converted into pg/mL. Only IL-8 is shown; the results for IL-6 were similar. Not all solvent fractions were available for this experiment. Composition of marker compounds is shown below the figure.
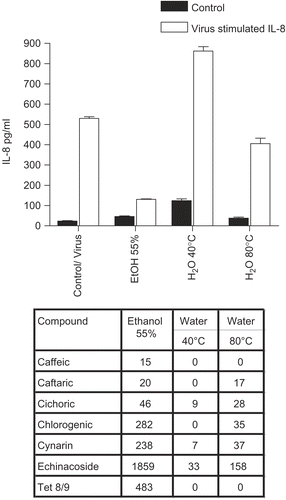
Figure 6. Anti-cytokine effects of E. pallida root extracts. BEAS-2B cells were infected with rhinovirus type 14 (1 pfu/cell) for 60 min at 35°C, followed by 250 μg/mL of the indicated fraction of E. pallida root extract. Controls received no virus. After 48 h, cell supernatants were removed for assay of IL-6 and IL-8 by standard ELISA tests. Standard curves were constructed for each experiment and the absorbance readings at 450 nm were converted into pg/mL. Only IL-8 data are shown; the results for IL-6 were similar. Composition of marker compounds is shown below the figure.
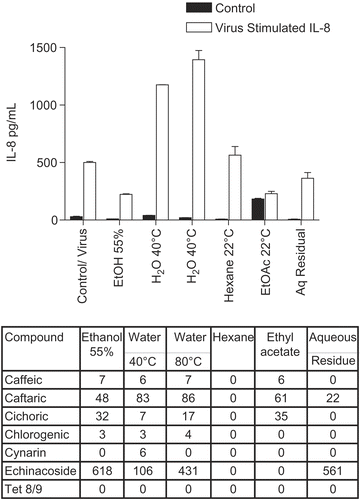
Discussion
Several conclusions can be derived from these results. Probably the most important is that we cannot correlate cytokine inhibitory effects, i.e., anti-inflammatory properties, with specific individual compounds or groups of marker compounds, namely, alkylamides, polysaccharides, and CADs. In the case of E. purpurea, all the fractions derived from roots, leaves plus stems, and flowers, were anti-inflammatory according to IL-6 and IL-8 measurements. The cytokine IL-6 is one of the principal pro-inflammatory cytokines, and is considered to be an excellent indicator of inflammatory status (CitationMessage & Johnston, 2004; CitationSchaller et al., 2006). The cytokine IL-8 is one of the most important chemokines which attracts neutrophils and other inflammatory cells to sites of infection (CitationSchaller et al., 2006). Numerous viral and bacterial infections, as well as wounds, result in substantial stimulation in levels of these two cytokines, which are consequently considered to represent markers of an inflammatory condition. Thus, any compound or herbal extract which can inhibit or reverse this elevation in IL-6/8 may be considered as a potential anti-inflammatory agent. Consequently, any preparation derived from roots or aerial parts of E. purpurea would be expected to possess anti-inflammatory properties, although flowers would be a considerably less potent source. This suggests that, if the same components were responsible for the inhibition, then substantially lower concentrations must be present in flowers. Some fractions contained small amounts of alkylamides, but these clearly did not enhance the anti-inflammatory effect. The fact that such preparations contain few if any alkylamides (CitationBinns et al., 2002; CitationVimalanathan et al., 2005) argues against the concept that alkylamides could be largely responsible for the immune modulation properties of Echinacea. Furthermore these extracts were all devoid of cynarin and echinacoside, while the flowers, with significantly less anti-inflammatory effect, were particularly rich in cichoric acid, all of which strongly suggests that CADs are also not important individually for anti-inflammatory activity.
In contrast, E. angustifolia and E. pallida fractions showed little or no such anti-inflammatory effects, and in fact some fractions from these extracts stimulated the already elevated levels of IL-6 and -8, especially those from E. pallida. Nevertheless, it is possible that E. angustifolia ethanol fraction possesses significant anti-inflammatory activity by virtue of its high concentration of alkylamides. In general, however, these observations support the conclusion that the inflammatory modulation ascribed to certain Echinacea extracts cannot be explained in terms of alkylamides, polysaccharides, or CADs individually.
It is also interesting to compare the anti-inflammatory activities described here with the previous antiviral results. E. purpurea root extracts contained substantial antiviral activity in their aqueous fractions, although much less in the ethanol fractions (CitationHudson et al., 2005). In contrast, roots of E. angustifolia did contain ethanol soluble but no water soluble antiviral activity, while E. pallida contained no antiviral activity at all. All aerial parts of E. purpurea contained antiviral activities, and these were found in most of the aqueous and ethanol fractions tested (CitationVimalanathan et al., 2005), but we were not able to correlate these activities with marker compounds.
Thus, it appears that the antiviral and anti- inflammatory activities do correspond, in that those fractions containing significant antiviral activity also have anti-inflammatory activity, but it is not clear which components of the Echinacea are responsible for them. Further studies need to be done with individual pure compounds and mixtures.
Although we cannot ascribe immune modulation properties to specific classes of marker compounds in the Echinacea preparations, on the basis of these results we could predict that most authentic preparations of E. purpurea roots, and aqueous preparations of E. purpurea aerial parts, should be effective anti-inflammatory mediators, at least in rhinovirus infected tissues, and therefore can be recommended for the alleviation of cold symptoms.
Acknowledgements
Declaration of interest: The authors report no conflicts of interest. The authors alone are responsible for the content and writing of the paper.
References
- Barrett B (2003): Medicinal properties of Echinacea: A critical review. Phytomedicine 10: 66–86.
- Barnes J, Anderson LA, Gibbons S, Phillipson JD (2005): Echinacea species (Echinacea angustifolia (DC.) Hell. Echinacea pallida (Nutt.) Nutt., Echinacea purpurea (L.) Moench: A review of their chemistry, pharmacology and clinical properties. J Pharm Pharmacol 57: 929–954.
- Bauer R (1998): Echinacea: Biological effects and active principals. Phytomedicines of Europe: Chemistry and biological activity. In: Lawson LD, Bauer R, eds., ACS Symposium Series 691. Washington, DC, American Chemical Society, pp. 140–157.
- Bauer R, Khan IA, Wagner H (1988): TLC and HPLC analysis of E. pallida, and E. angustifolia roots. Planta Med 54: 426–430.
- Binns SE, Livesey JF, Arnason JT, Baum BR (2002): Phytochemical variation in Echinacea from roots and flowerheads of wild and cultivated populations. J Agric Food Chem 50: 3673–3687.
- Burger RA, Torres AR, Warren RP, Caldwell VD, Hughes BD (1997): Echinacea-induced cytokine production by human macrophages. Int J Immunopharmacol 19: 371–379.
- Edwards MR, Hewson CA, Laza-Stanca V, Lau HTH, Mukaida N, Hershenson MB, Johnston SL (2007): Protein kinase R, IkB kinase b, and NFkB are required for human rhinovirus induced pro-inflammatory cytokine production in bronchial epithelial cells. Mol Immunol 44: 1587–1597.
- Gertsch J, Schoop R, Kuenzle U, Suter A (2004): Echinacea alkylamides modulate TNF-alpha gene expression via cannabinoid receptor CB2 and multiple signal transduction pathways. FEBS Lett 577: 563–569.
- Goel V, Chang C, Slama JV, Barton R, Bauer R, Gahler R, Basu TK (2002): Echinacea stimulates macrophage function in the lung and spleen of normal rats. J Nutr Biochem 13: 487–492.
- Hudson JB, Graham A, Towers GHN (1982): Nature of the interaction between the photoactive compound phenylheptatriyne and animal viruses. Photochem Photobiol 36: 181–185.
- Hudson J, Vimalanathan S, Kang L, Treyvaud Amiguet V, Livesey J, Arnason JT (2005): Characterization of antiviral activities in Echinacea root preparations. Pharm Biol 43: 790–796.
- LaLone CA, Hammer KDP, Wu L, Bae J, Leyva M, Liu Y, Solco AKS, George A, Kraus GA, Murphy PA, Wurtele ES, Kim O-K, Seo K, Widrlechner MP, Birt DF (2007): Echinacea species and alkamides inhibit prostaglandin E2 production in RAW 264.7 mouse macrophage cells. J Agric Food Chem 55: 7314–7322.
- Merali S Binns SE, Paulin-Levasseur M, Ficker C, Smith M, Baum BR, Brovelli E, Arnason JT (2003): Antifungal and anti-inflammatory activity of the genus Echinacea (Asteraceae). Pharm Biol 41: 412–420.
- Message SD, Johnston SL (2004): Host defense function of the airway epithelium in health and disease clinical. backgroundJ Leukocyte Biol 75: 1–13.
- Mosser AG, Vrtis R, Burchell L, Lee WM, Dick CR, Weisshaar E, Bock D, Swenson CA, Cornwell RD, Meyer KC, Jarjour NN, Busse WW, Gern JE (2005): Quantitative and qualitative analysis of rhinovirus infection in bronchial tissues. Am J Respir Crit Care Med 171: 645–651.
- Raduner S, Majewska A, Chen JZ, Xie XQ, Hamon J, Faller B, Altmann KH, Gertsch J (2006): Alkylamides from Echinacea are a new class of cannabinomimetics. J Biol Chem 281: 14192–14206.
- Rininger JA, Kickner S, Chigurupati P, McLean A, Franck Z (2000): Immunopharmacological activity of Echinacea preparations following simulated digestion on murine macrophages and human peripheral blood cells. J Leukocyte Biol 68: 503–510.
- Schaller M, Hogaboam CM, Lukacs N, Kunkel SL (2006): Respiratory viral infections drive chemokine expression and exacerbate the asthmatic response. J Allergy Clin Immunol 118: 295–302.
- Sharma M, Arnason JT, Burt A, Hudson JB (2006): Echinacea extracts modulate the pattern of chemokine and cytokine secretion in rhinovirus-infected and uninfected epithelial cells. Phytother Res 20: 147–152.
- Vimalanathan S, Kang L, Treyvaud Amiguet V, Livesey J, Arnason JT, Hudson J (2005): Echinacea purpurea aerial parts contain multiple antiviral compounds. Pharm Biol 43: 740–745.