Abstract
An altered intracellular calcium metabolism is known to play an essential role in the pathophysiology of diabetes and its complications. Tea [Camellia sinensis L. (Theaceae)] contains polyphenolic compounds collectively known as catechins belonging to the flavonoids family. Catechins have been reported to possess several biological properties including an antidiabetic effect. The present paper reports the effect of tea catechins on erythrocyte membrane Ca++-pump in normal and type 2 diabetic patients. The activity of erythrocyte Ca++-ATPase was significantly decreased in type 2 diabetes. In vitro incubation with tea catechins (10−3-10−8 M) caused an increase in the activity of Ca++-ATPase in both normal and type 2 diabetic subjects; the effect was more pronounced in type 2 diabetes and was observed at much lower concentrations (10−5 M) compared to normal (10−3 M). The order of effectiveness of individual catechins was in the order: EGCG > ECG > EC > EGC. The increase in Ca++-ATPase activity after incubation with tea catechins may be explained on the basis of alteration in fluidity due to a direct effect of catechins on the membrane.
Introduction
Diabetes mellitus makes an individual prone to various complications such as macro and microvascular disease, hypertension, neuropathy, cataracts, cardiomyopathy, and premature aging, thereby indicating that these complications develop through a similar pathway common to diabetic conditions (CitationBrownlee, 2005). It is known that intracellular calcium concentration is increased in most tissues in the diabetic condition, and this altered intracellular calcium metabolism seems to result from a common, underlying abnormality linking the metabolic, cardiovascular, ocular, and neural manifestations of the diabetic disease process (CitationLevy, 1994).
The red blood cell along with its membrane has always been an important medium for study due to the important role it plays in various physiological and metabolic aspects. Several alterations in erythrocytes have been reported in type 1 and type 2 diabetes mellitus (CitationWatala, 1993).
Membrane-bound calcium-transporting proteins are important in regulating various signal functions of calcium (CitationCarafoli, 1987). The regulation of this calcium is performed by Ca++-ATPase or calcium pump. An altered activity of erythrocyte membrane Ca++-ATPase has been reported in the diabetic condition (CitationGonzalez-Flecha et al., 1990). Reduced membrane Ca++-ATPase activity, as seen in type 2 diabetics, may be responsible for an increase in intracellular calcium and, consequently, for elevated vascular resistance which is frequently associated with hypertension (CitationZemel et al., 1990).
Tea - Camellia sinensis L. (Theaceae) - is the most popular beverage worldwide. Epidemiological studies indicate that tea consumption may reduce the risk of cardiovascular disease (CitationTijburg et al., 1997). Tea contains polyphenolic compounds (collectively known as catechins) belonging to the flavonoid family. Quantitatively, the most important catechins in green tea are: epigallocatechin gallate (EGCG), epigallocatechin (EGC), epicatechin gallate (ECG) and epicatechin (EC) (). Catechins are known to possess antioxidant, anticancer, cardioprotective, vasorelaxant, and hypoglycemic activities (CitationCabrera et al., 2006). The exact mechanisms underlying these remain speculative. In earlier reports we have demonstrated the effect of tea catechins on biomarkers of oxidative stress on diabetic erythrocytes (CitationRizvi et al. 2005) and on erythrocyte Na/H antiport (CitationRizvi & Zaid, 2005). The present study was undertaken to evaluate the effect of tea catechins (EGCG, EGC, ECG, and EC) on erythrocyte Ca++-ATPase in normal (control) and type 2 diabetic patients.
Materials and methods
Selection of subjects
The criteria for selection of type 2 diabetic patients were the same as reported earlier (CitationRizvi et al., 2005; CitationRizvi & Zaid, 2005). Blood from 31 diabetic patients (18 men, 13 women) was taken after informed consent had been obtained from all patients, with the following characteristics: mean age 58 ± 7 years, fasting plasma glucose level 183.5 ± 42.4 mg/dL, BMI 27 ± 4 kg/m2, total plasma cholesterol 5.4 ± 1.3 mM/L and duration of diabetes was 12 ± 5 years. None of the patients had high blood pressure or microalbuminuria. Care was also taken to exclude patients who had a family history of hypertension.
The control group consisted of 31 healthy volunteers age and sex matched with diabetic subjects, mean age 56 ± 8 years, fasting plasma glucose level 85.2 ± 14.4 mg/dL, BMI 24.8 ± 3.8 kg/m2, and total plasma cholesterol 5.3 ± 1.3 mM. None of the controls was affected by hypertension. Care was taken to select control subjects with no family history of diabetes mellitus or hypertension (two generations). None of the women studied was receiving any hormonal treatment. All volunteers (diabetic patients and normal subjects) were informed about the nature of the study.
Collection of blood, isolation of packed RBC and preparation of ghosts
Venous blood was collected from control and type 2 diabetic patients after an overnight fast using ACD (citric acid/sodium citrate/dextrose) as anticoagulant. The blood sample was kept at 37°C for 3 h prior to experiments for degradation of endogenous insulin. The blood sample were centrifuged at 4°C for 10 min at 1000 g to remove plasma and buffy coat and the isolated erythrocytes were washed 4 to 5 times with 0.154 M NaCl, and finally packed erythrocytes were obtained. The erythrocyte membrane from leukocyte-free red cells were prepared following the method of CitationMarchesi and Palade (1967), that involves the principle of osmotic shock treatment with hypotonic and hypertonic buffers (pH 7.4).
Determination of erythrocyte membrane Ca++-ATPase activity
Ca++-ATPase activity was assayed as described earlier (CitationRizvi & Luqman, 2003). The assay mixture (2.25 mL) contained 80 mM NaCl, 15 mM KCl, 3 mM MgCl2, 18 mM Tris-HCl (pH 7.4), 0.1 mM ouabain, 0.1 mM EGTA, 0.2 mL of the membrane solution containing 0.4 to1.5 mg membrane protein per mL and ± 0.2 mM CaCl2. The reaction was initiated by addition of 0.1 mL of 30 mM ATP. The incubation of the assay mixture was carried out at 37°C for 30 min. The reaction was stopped by adding 3.5 mL of a solution containing 0.5 M H2SO4, 0.5% ammonium molybdate and 2% SDS. Liberated inorganic phosphate was estimated by a modified method of CitationFiske and Subbarow (1925) and Ca++-ATPase activity is expressed in terms of μmol of Pi released/h/mg membrane protein at 37°C.
In vitro experiments were carried out by adding the indicated concentration of tea catechins to the enzyme assay medium and incubating at 37°C for 30 min prior to enzyme assay. Appropriate control experiments were set for each experiment where assay medium was incubated without tea catechins. Blood glucose values were determined by Accu Check Glucometer (Roche). Erythrocyte membrane protein was estimated following the established method of CitationLowry et al. (1951).
Statistical analysis was carried out by employing Students t-test, with a probability of 0.01 being used as a level of significance.
Results and discussion
The activity of erythrocyte membrane Ca++-ATPase was significantly decreased in type 2 diabetic subjects (control 0.343 ± 0.017; type 2 diabetes 0.199 ± 0.027). Our results are in agreement with other reports (CitationGonzalez-Flecha et al., 1990; CitationZemel et al., 1998). Reduced Ca++-ATPase activity in type 2 diabetes may be responsible for increase in intracellular calcium and consequently for elevated vascular resistance which is frequently associated with hypertension (CitationZemel et al., 1990). An altered erythrocyte Ca++-ATPase activity has been linked to the development of neuropathy in diabetes (CitationJain & Lim, 2000). The decrease in Ca++-ATPase activity in type 2 diabetes may be due to altered membrane properties including functional and compositional changes (CitationShin et al., 2007; CitationBakan et al., 2006). A relationship has been reported between the blood glucose levels and erythrocyte membrane ATPases (CitationAdamson et al., 1986). It is reported that glycosylation of erythrocyte membrane proteins significantly inhibit Ca++-ATPase activity (CitationDavis et al., 1985). The decreased Ca++-ATPase observed in our studies in diabetic erythrocytes may be due to glycosylation of membrane protein.
The concentration-dependent effect of tea catechins (EGCG, EGC, ECG, and EC) on the activity of Ca++-ATPase in normal (control) subjects is shown in and type 2 diabetic subjects in . In vitro incubation with tea catechins caused an increase in the activity of Ca++-ATPase in both normal and type 2 diabetic subjects: the effect was more pronounced in type 2 diabetes. The effect was concentration-dependent, and a significant activation was visible at concentrations up to 10−3 M in normal (control) and 10−5 M in type 2 diabetes. The order of effectiveness of individual catechins was in the order: EGCG > ECG > EC > EGC. Catechins are bioavailable to humans after drinking tea. Catechin levels in human plasma reach their peak 2 to 4 h after ingestion (CitationYang et al., 1998). A study comparing the pharmacokinetics of equimolar doses of pure EGC, ECG, and EGCG in healthy humans reported that the peak plasma levels of each catechin reached micromolar concentrations, although there was a difference in the plasma level of individual catechins (CitationHigdon & Frei, 2003). CitationChow et al. (2005) report a peak plasma level of 7.4 μmol/L of EGCG after a dose of 1,200 mg of EGCG in the fasting condition.
Figure 2. Concentration-dependent effect of tea catechins on erythrocyte membrane Ca++-ATPase activity. (A) Normal human: EGCG, EGC show significant (p <0.01) activation of Ca2+ ATPase at 10−5 M concentration. ECG and EC show significant activation only at 10−3 and 10−4 M. (B) Type 2 diabetic patients: EGCG, EGC, ECG, EC show significant activation of Ca2+ ATPase at 10−3 – 10−5 M concentration. *Ca++ ATPase activity is expressed as μmol of Pi released /h/mg membrane protein at 37°C. Values are mean ± SD.
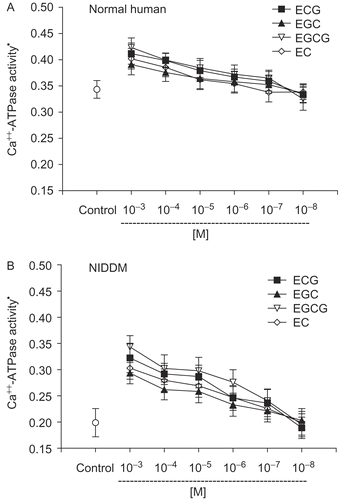
Catechins have been reported to modulate membrane fluidity and this property has been suggested to contribute to their medicinal utility (CitationTsuchiya, 2001). An altered membrane fluidity has been speculated to explain the effect of tea catechins (EGCG, EGC, ECG, EC) on erythrocyte membrane Na+K+ATPase and Na/H antiport activity (CitationRizvi & Zaid, 2005). Our observation of an increase in Ca++-ATPase activity after incubation with tea catechins may be explained on the basis of alteration in fluidity due to a direct effect of catechins on the membrane. It has been shown that EGCG is capable of protecting erythrocyte membrane-bound ATPases against oxidative stress (CitationSaffari & Sadrzadeh, 2004). This effect may also contribute to the elevation in activity of Ca++-ATPase after treatment with catechins.
The importance of our finding on the effect of tea catechins on Ca++-ATPase lies in the fact that altered intracellular calcium metabolism plays an essential role in the pathophysiology of diabetic complications (CitationLevy, 1994) and other diseases (CitationFujita, 2000). The effect on Ca-ATPase may help to explain, in part, the antidiabetic effects of tea catechins.
Acknowledgements
This research was supported by the International Foundation for Science, Stockholm, Sweden, and the Organization for the Prohibition of Chemical Weapons, The Hague, The Netherlands, through a grant to S. I. Rizvi. Mohd Abu Zaid is a recipient of a Research Associate fellowship from the Council for Scientific and Industrial Research, New Delhi.
Declaration of interest: The authors report no conflicts of interest. The authors alone are responsible for the content and writing of the paper.
References
- Adamson I, Okafor C, Abi-Bakre A (1986): Erythrocyte membrane ATPase in diabetes: Effect of dikanut (Irvingia gabonensis). Enzyme 36: 212–215.
- Bakan E, Yildirim A, Kurtul N, Polat MF, Dursun H, Cayir K (2006): Effects of type 2 diabetes mellitus on plasma fatty acid composition and cholesterol content of erythrocyte and leucocyte membranes. Acta Diabetol 43: 109–113
- Brownlee M (2005): The pathobiology of diabetic complications. A unifying mechanism. Diabetes 54: 1615–1625.
- Cabrera C, Artacho R, Gimenez R (2006): Beneficial effects of green tea—a review. J Am Coll Nutr 25: 79–99.
- Carafoli, E (1987): Intracellular calcium homeostasis. Annu Rev Biochem, 56: 395–433.
- Chow HHS, Hakim IA, Vining DR, Crowell JA, Ranger-Moore J, Chew WM, Celaya CA, Rodney SR, Hara Y, Alberts DS (2005): Effects of dosing condition on the oral bioavailability of green tea catechins after single-dose administration of polyphenon E in healthy individuals. Clin Cancer Res 11: 4627–4633.
- Davis FB, Davis PJ, Nat G, Blas SD, MacGillivaray M, Gutman S, Feldman MJ (1985): The effect of in vivo glucose administration on human erythrocyte Ca++-ATPase activity on enzyme responsiveness in vitro to thyroid and calmodulin. Diabetes 34: 639–646.
- Fiske C, Subbarow Y (1925): The colorimetric determination of phosphorus. J Biol Chem 66: 375–400.
- Fujita T (2000): Calcium paradox: Consequences of calcium deficiency manifested by a wide variety of diseases. J Bone Miner Metab 18: 234–246
- Gonzalez-Flecha FL, Bermudaz CM, Cedola NV, Gargliardino JJ, Rossi JPFC (1990): Decreased Ca++-ATPase activity after glycosylation of erythrocyte membranes in vivo and in vitro. Diabetes 39: 707–711.
- Higdon JV, Frei B (2003): Tea catechins and polyphenols: Health effects, metabolism, and antioxidant functions. Crit Rev Food Sci Nutr 43: 89–143.
- Jain SK, Lim G (2000): Lipoic acid decreases lipid peroxidation and protein glycosylation and increases (Na(+) + K(+))- and Ca(++)-ATPase activities in high glucose-treated human erythrocytes. Free Radic Biol Med 29: 1122–1128.
- Levy J (1994): Diabetes mellitus: A disease of abnormal cellular calcium metabolism? Am J Med 96: 260–273.
- Lowry OH, Rosebrough NJ, Farr AL, Randall RJ (1951): Protein measurement with Folin-phenol reagent. J Biol Chem 193: 265–275.
- Marchesi VT, Palade GE (1967): The localization of Mg-Na-K-activated adenosine triphosphatase on red cell ghost membranes. J Cell Biol 35: 385–404.
- Rizvi SI, Abu Zaid M, Anis R, Mishra N (2005): Protective role of tea catechina against oxidation-induced damage of type 2 diabetic erythrocytes. Clin Exp Pharmacol Physiol 32: 70–75.
- Rizvi SI, Abu Zaid M, (2005): Impairment of sodium pump and Na/H exchanger in erythrocytes from NIDDM patients. Effect of tea catechins. Clin Chim Acta 354: 59–67.
- Rizvi SI, Luqman S (2003): Capsaicin-induced activation of erythrocyte membrane sodium/potassium and calcium adenosine triphosphatases. Cell Mol Biol Lett 8: 919–925.
- Saffari Y, Sadrzadeh SM (2004): Green tea metabolite EGCG protects membranes against oxidative damage in vitro. Life Sci 74: 1513–1518.
- Shin S, Ku Y, Babu N, Singh M (2007): Erythrocyte deformability and its variation in diabetes mellitus. Indian J Exp Biol 45: 121–128.
- Tsuchiya H (2001): Stereospecificity in membrane effects of catechins. Chem Biol Interact 134: 41–54.
- Watala C (1993): Altered structural and dynamic properties of blood cell membranes in diabetes mellitus. Diabet Med 10: 13–20.
- Tijburg LBM, Mattern T, Folts JD, Weisgerber UM, Katan MB (1997): Tea flavonoids and cardiovascular diseases: A review. Crit Rev Food Sci 37: 771–785.
- Yang CS, Chen L, Lee MJ, Balentine D, Kuo M, Schantz SP (1998): Blood and urine levels of tea catechins after ingestion of different amounts of green tea by human volunteers. Cancer Epidemiol Biomarkers Prev 7: 351–354.
- Zemel MB, Bedford BA, Zemel PC, Marwah O, Sowers JR (1998): Altered cation transport in non-insulin dependent diabetic hypertension: Effects of dietary calcium. J Hypertens 6: S228–S230.
- Zemel MB, Sowers JR, Shehin S, Walsh MF, Levy J (1990): Impaired calcium metabolism associated with hypertension in Zucker obese rats. Metabolism 39: 704–708.