Abstract
Plants are universally recognized as a vital part of the world’s natural heritage and up to 80% of the population rely on plants for their primary healthcare. Varieties of medicinal plants are recognized as a source of natural antioxidants that can protect from oxidative stress, thus playing an important role in chemoprevention of diseases. In the present investigation, 22 extracts from different parts of eight medicinal plants (Punica granatum Linn. (Punicaceae), Caesalpinia bonducella Flem. (Fabaceae), Hibiscus subdariffa Linn. [(Malvaceae), Moringa oleifera Lam. (Moringaceae), Garcinia indica Linn. (Clusiaceae), Emblica officinalis Gaertn. (Euphorbiaceae), Momordica charantia Linn. (Cucurbitaceae), and Matricaria chamomilla Linn. (Asteraceae)] were screened for their protection against oxidative stress in erythrocytes induced by hydrogen peroxide (2 mM) and tert-butyl hydroperoxide (0.01 mM). The effect was also compared with known antioxidants and flavonoids. Subjecting erythrocytes to oxidative stress by incubation with hydrogen peroxide and tert-butyl hydroperoxide caused a significant alteration in reduced glutathione (GSH) and malondialdehyde (MDA) concentration compared to the control. Our results show that medicinal plant extracts protect erythrocytes from hydrogen peroxide and tert-butyl hydroperoxide induced oxidative stress; known antioxidant (vitamin C, E, and β-carotene) and flavonoid (quercetin) also showed a similar protective effect. Our observations may, in part, suggest the use of the spent/waste parts of medicinal plants. This could be an economically viable source of natural and potent antioxidants effective against complications arising from oxidative stress. The results may also improve the ethanopharmacological knowledge of medicinal plants.
Introduction
Plants, universally recognized as a vital part of world’s natural heritage, supply our basic needs such as food, fibers, fuel, and many important medicines. The traditional systems of medicine such as Ayurveda, Siddha, Unani, homeopathy, and folklore of various countries, depend on plants for their medicinal preparation. The medicinal properties of plants are mainly attributed to the presence of flavonoids, coumarins, phenolic acids, and antioxidant micronutrients such as Cu, Zn and Mn (CitationRepetto & Llesuy, 2002; CitationSeth & Sharma, 2004). Varieties of medicinal plants are recognized as a source of natural antioxidants that can protect from oxidative stress and thus play important role in chemoprevention of diseases (CitationLee et al., 2003; CitationKatalinic et al., 2006).
Oxidative stress arising from free radicals like reactive oxygen species (ROS) now appears to be a fundamental mechanism underlying degenerative diseases such as diabetes, viral infection, autoimmune pathologies and probably aging. Evidence suggests that ROS can be scavenged through chemoprevention utilizing antioxidant compounds present in foods and medicinal plants (CitationSurh, 1999; CitationFinkel & Holbrook, 2000). Antioxidant principles from herbal resources are multifaceted in their effects and their proper intake helps us to fight against various metabolic imbalances. It has been reported that nutritional intervention to increase intake of phyto-antioxidants may reduce the threat of free radicals (CitationNg et al., 2000; CitationArora et al., 2003). Plants play a significant role in maintaining human health and improving the quality of human life. They serve humans as valuable components of food, cosmetics, dyes, and medicines. The World Health Organization estimated that 80% of the earth’s inhabitants rely on traditional medicine for their primary healthcare needs, and most of this therapy involves the use of plant extracts and their active components (CitationWinston, 1999; CitationTripathi et al., 2007).
Erythrocytes are well equipped to degrade reactive oxygen species via the actions of superoxide dismutase that converts O2 into H2O2, which is further catabolized by catalase and glutathione peroxidase. Mammalian cells contain 1-10 mM cytosolic glutathione (GSH) under normal physiological conditions, depending on the cell type and metabolic factors. GSH represents approximately 95% of total non-protein thiols and is the main modulator of the cellular redox environment. The cytoplasmic ratio of reduced to oxidized glutathione (GSH/GSSG) of approximately 100/1, maintains the cysteine residues of intracellular proteins in the reduced form. GSSG generation from GSH can be favored during mild oxidative stress conditions. The oxidation of GSH to GSSG can dramatically change the GSH/GSSG ratio and consequently the redox status within the cells. As a result, the thiol group of cytosolic proteins is modified by the reversible formation of protein GSH mixed disulfides, a process known as S-glutathionylation (CitationDavies & Goldberg, 1987; CitationRohn et al., 1998; CitationDi Simplicio et al., 1998; CitationTelci et al., 2000). Due to oxidative stress, polyunsaturated fatty acid of erythrocyte membrane is damaged resulting in a steep increase in malondialdehyde (MDA) concentration, a biomarker currently used for studying oxidation of lipids under different conditions (CitationPradhan et al., 1990; CitationKonukoglu et al., 1998; CitationLopez-Revuelta et al., 2005).
In the present study, erythrocytes are used as a model system for studying oxidative damage and its pathophysiology. Twenty-two extracts prepared from different parts of eight medicinal plants – Punica granatum Linn. (Punicaceae), Caesalpinia bonducella Flem. (Fabaceae), Hibiscus subdariffa Linn. (Malvaceae), Moringa oleifera Lam. (Moringaceae), Garcinia indica Linn. (Clusiaceae), Emblica officinalis Gaertn. (Euphorbiaceae), Momordica charantia Linn. (Cucurbitaceae) and Matricaria chamomilla Linn. (Asteraceae) – were screened for their protection against oxidative stress in erythrocytes induced by hydrogen peroxide and tert-butyl hydroperoxide and the effect was compared with standard antioxidants like vitamin E, vitamin C, β-carotene, and quercetin (a flavonoid). Evaluation of GSH and MDA concentration was done and the protective effect of antioxidants and plant extracts in erythrocytes subjected to oxidation by hydrogen peroxide/tert-butyl hydroperoxide were compared and recorded. The results demonstrated that some of the extracts showed significant protection against hydrogen peroxide/tert-butyl hydroperoxide-induced oxidative stress.
Materials and methods
Collection of plant material and extract preparation
The plant materials were collected either from the research farm of CIMAP (Central Institute of Medicinal and Aromatic Plants, Lucknow) or purchased from the local market during January to September 2006. The plant materials were authenticated by J. Singh, S.P. Jain, and S.C. Singh, and the voucher specimens were deposited in Gyan Surabhi (). Extracts were prepared as described previously with slight modification (CitationLuqman et al., 2005). Plant samples were washed and shade-dried at 40°C. Then, dried plant samples were extracted with ethanol (99.5%, Merck Specialities, Mumbai, India) and/or distilled water. Three-step extractions were done and extracts were filtered and concentrated in a rotary evaporator (Buchi, Flavil, Switzerland) at 45°C. All the extracts were dried at 40°C in a hot air oven and stored at 4°C. Plant extract were prepared by dissolving 100mg of extracts either in dimethyl sulfoxide (DMSO 99.5%) or in methanol (99.8%; Merck Specialities, Mumbai, India). The final concentration of DMSO and/or methanol was always < 0.05% in the experimental solution.
Table 1. List of plants and plant parts.
Collection of blood and isolation of packed erythrocytes
Retino-orbital blood from healthy male mice (aged 120–145 days, bodyweight 35 ± 6 g, hemoglobin 14 ± 3 g/mL) was collected for experiments using heparin (10 units/mL) as the anti-coagulant. The collected blood was stored at 4°C and was used for experiments within four hours of collection (CitationLuqman & Rizvi, 2006; CitationRizvi & Luqman, 2002). Blood samples were centrifuged at 4°C for 10 min at 100 g to remove plasma and buffy coat and the isolated erythrocytes were washed 3–4 times with 0.154 M NaCl, and finally packed erythrocytes were obtained. The animals were authenticated and approved by the Institutional Animal Ethical Committee (IAEC) of the Central Institute of Medicinal and Aromatic Plants (CIMAP), Lucknow.
Estimation of reduced glutathione concentration
The reduced glutathione concentration in erythrocytes was estimated using the standard method of CitationBeutler et al. (1963) as reported previously (CitationRizvi & Luqman, 2002) with slight modification. To 100 μL of packed RBC, 900 μL of phosphate solution was added. Tubes were centrifuged at 5,000 rpm for 5 min and supernatant was discarded. To 100 μL of RBC (pellet), 100 μL of phosphate solution was added. Next, 900 μL of distilled water was added to the suspension, followed by the addition of 1.5 mL precipitation solution, and the tubes were centrifuged at 5,000 rpm for 3 min. To the 50 μL of supernatant, 200 μL phosphate solution and 25 μL of freshly prepared DTNB were added. This method is based on the ability of the sulfhydryl group to reduce 5, 5´-dithiobis 2-nitro benzoic acid (DTNB) and form a yellow-colored anionic product whose absorbance is measured at 412 nm. The concentration of GSH is expressed as μmol/mL of packed erythrocytes and was determined from a standard plot.
Estimation of malondialdehyde concentration
Erythrocyte malondialdehyde formed during lipid peroxidation was measured according to the method of CitationEsterbauer and Cheeseman (1990) as described earlier (CitationLuqman & Rizvi, 2006). Packed erythrocytes (200 μL) were suspended in 3 mL of PBS-glucose solution (pH 7.4). To 1 mL of the suspension, 1 mL of 10% TCA was added. Centrifugation was done for 5 min at 5,000 rpm. To 1 mL of supernatant, 1 mL of 0.67% TBA in 0.05 M NaOH was added. Tubes were kept in a boiling water bath for 20 min at a temperature greater than 90°C and then cooled. Absorbance was measured at 532 nm (OD1) and 600 nm (OD2) against a blank. The net optical density (OD) was calculated after subtracting absorbance at OD2 from that of OD1. The concentration of MDA in erythrocytes was determined from a standard plot and expressed in terms of nmol/mL of packed erythrocytes.
In vitro experiments with plant extracts, vitamins, quercetin and induction of oxidative stress
The protective effect of plant extracts on erythrocyte GSH and MDA concentration was investigated as follows: blood samples were washed three times with 5 mM phosphate buffer saline containing glucose (pH 7.4). Erythrocytes were then suspended in 10 volumes of phosphate buffer saline. The protective effect of plant extracts (100 μg/mL), vitamins (10 μg/mL) and quercetin (10 μg/mL) were evaluated by pre-incubating erythrocytes separately for 30 min at 37°C. Oxidative stress was induced in vitro by incubating erythrocytes with hydrogen peroxide (2 mM)/tert-butyl hydroperoxide (0.01 mM) in the above experiments. The concentration of hydrogen peroxide and tert-butyl hydroperoxide used in the present study to induce oxidative stress was in the range of concentrations used in previously published reports (CitationMueller et al., 1997; CitationMendiratta et al., 1998; CitationDomanski et al., 2005; CitationIglesias & Catala, 2005; CitationRizvi et al., 2005; CitationRizvi & Kumar, 2005).
Statistical analysis
Statistical analysis of the data obtained was performed by employing mean ± standard deviation (SD). A Student’s t-test was used to make a statistical comparison between two-tailed paired groups. A comparison was done with the control group versus oxidized group and oxidized group versus extract treated group. The significance levels were set at p <0.001, <0.01 and/or <0.05.
Results and discussion
Erythrocytes are unique cells well equipped to degrade reactive oxygen species through superoxide dismutase, catalase, glutathione/glutathione peroxidase, methemoglobin reductase, and membrane bound α-tocopherol (CitationHalliwell & Gutteridge, 1986). Induction of oxidative stress following incubation with hydrogen peroxide and tert-butyl hydroperoxide resulted in a decrease in GSH concentration (57.8% and 49.4% respectively) in erythrocytes as compared to control (). The ability of standard antioxidant to maintain reduced GSH levels in erythrocytes was found to be in the following order: β-carotene > quercetin > vitamin C > vitamin E (hydrogen peroxide), β-carotene > vitamin C > quercetin = vitamin E (tert-butyl hydroperoxide).
Figure 1. Protective effect of vitamins and flavonoid on erythrocyte reduced glutathione concentration stressed with hydrogen peroxide/tert-butyl hydroperoxide. * Concentration of GSH is expressed as μmol/ml of packed erythrocytes; Values are mean ± SD of three experiments in replicates; ¤ p < 0.01.
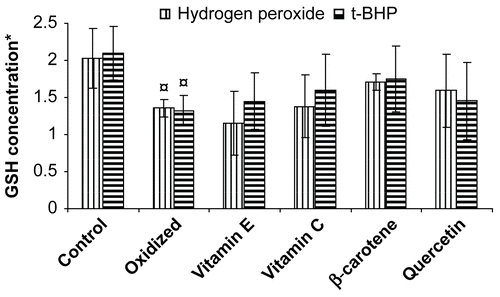
β-Carotene was found to be a more potent antioxidant, which is effective against oxidative stress generated by tert-butyl hydroperoxide and hydrogen peroxide. Our finding corroborates with previous reports on protection of β-carotene against radiation-induced oxidative stress which is attributed to the quenching of singlet oxygen (CitationGerster, 1993). In addition, β-carotene has also been reported to scavenge a variety of free radical species (CitationKrinsky & Deneke, 1982). Epidemiological studies have shown a link between dietary carotenoids and the reduced incidence of certain disease, including cancers (CitationManda & Bhatia, 2003). However, few reports (CitationOmenn et al., 1996) have shown β-carotene supplementation has little or no beneficial effect. The presence of plant extracts in the incubation medium protected the erythrocytes from hydrogen peroxide and tert-butyl hydroperoxide-induced oxidative stress, as evidenced by maintaining GSH concentration; known antioxidants also showed a similar effect (; ). The protective effect of medicinal plant extract was found to be significant at 100 μg/mL. The alcoholic and aqueous seed extract of Caesalpinia bonducella showed maximum protection of GSH concentration against the stress induced by hydrogen peroxide whereas when the stress was induced by t-BHP, the aqueous extract of Punica granatum seed showed maximum protection. Lower concentration of medicinal plant extracts did not show significant protection of GSH concentration in erythrocytes against the stress induced by hydrogen peroxide and tert-butyl hydroperoxide (data not shown).
Table 2. Protective effects of plant extracts on reduced glutathione and malondialdehyde concentration in erythrocytes stressed by hydrogen peroxide and tert-butyl hydroperoxide.
Reduced glutathione is a major intracellular non-protein sulfhydryl compound having many biological functions, including maintenance of membrane protein -SH groups in the reduced form, the oxidation of which can otherwise cause altered cellular structure and function (CitationReglinski et al., 1988; CitationDi Simplicio et al., 1996). Membrane -SH group oxidative damage may be an important molecular mechanism inducing changes in the membrane microelasticity or whole cell deformability of erythrocytes under conditions of physiological and pathological oxidative stress. The importance of protein -SH groups as critical buffers to maintain the intracellular redox state has been suggested by the report of protein thiol having reactivity similar to or greater than that of GSH (CitationWang et al., 1999).
The erythrocyte membrane is prone to lipid peroxidation under oxidative stress that leads to the formation of MDA, a biomarker used for studying the oxidation of lipids under different conditions. Subjecting erythrocytes to oxidative stress by incubating them with hydrogen peroxide and/or tert-butyl hydroperoxide caused an increase in MDA (110/76%) concentration above the basal level in erythrocytes. MDA concentration did not increase in vitamin C (10 μg/mL) treated erythrocytes stressed with hydrogen peroxide. The ability of standard antioxidant to maintain MDA concentration in erythrocytes was found to be in the following order: vitamin C > quercetin > β-carotene > vitamin E. Our results suggest that cellular injury caused by hydrogen peroxide through generation of superoxide ion and OH radical can be checked by increasing vitamin C dosage and even reduced GSH level can be efficiently replenished in erythrocytes. Vitamin C reacts with the α-tocopheroxyl radical to regenerate α-tocopherol (CitationBuettner, 1993). Further recycling of vitamin C occurs from the ascorbate free radical (one-electron oxidized form) and dehydroascorbic acid (two-electron oxidized form), respectively (CitationMay et al., 1998). Ascorbate free radical can be reduced by NADH-dependent cytochrome b5 reductases or thioredoxin reductase (CitationMay et al., 2004). Dehydroascorbic acid can be reduced directly by GSH and GSH-dependent enzymes (CitationWashburn & Wells, 1999), or by NADPH-dependent dehydroascorbate reductase (CitationDel Bello et al., 1994).
Quercetin (10 μg/mL) was found to be most efficient in protecting the lipid peroxidation, thereby limiting MDA formation in erythrocytes stressed with tert-butyl hydroperoxide. This suggests that quercetin may inhibit peroxynitrite radical formation that usually increases due its presence and even normal activity of Ca++-ATPase pump can be attained. Agents like superoxide ion and hydrogen peroxide that are produced due to interaction of tert-butyl hydroperoxide with Hb and MetHb can be decreased in the presence of quercetin and thus cellular injury can be checked (CitationDeliconstantinos et al., 1996). Protective effects of quercetin against hydrogen peroxide-induced DNA damage have been reported in previous studies (CitationMusonda & Chipman, 1998; CitationBlasiak et al., 2002; CitationPeng & Kuo, 2003). It was also found that quercetin efficiently protects hydrogen peroxide-induced DNA damage in RLE cells (CitationBoots et al., 2007). The plant extracts, when present in the incubation medium at a concentration of 100 μg/mL, were found to protect the erythrocytes from the damage induced by hydrogen peroxide and tert-butyl hydroperoxide (; ). The alcoholic extract of Hibiscus subdariffa sepal was able to protect the erythrocytes from oxidative stress induced by both hydrogen peroxide and tert-butyl hydroperoxide, to the maximum extent among all other plant extracts ().
Figure 2. Protective effect of vitamins and flavonoid on erythrocyte malondialdehyde concentration stressed with hydrogen peroxide/tert- butylhydroperoxide. *Concentration of MDA is expressed as nmol/ml of packed erythrocytes; Values are mean ± SD of three experiments in replicates; ¤ p < 0.01.
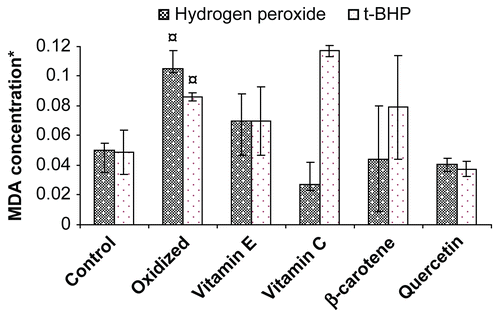
Table 3. Ascending order of effectiveness of medicinal plant extract providing protection to erythrocytes stressed by hydrogen peroxide and tert-butyl hydroperoxide.
Oxidation with hydrogen peroxide and tert-butyl hydroperoxide resulted in increased erythrocyte MDA concentrations and lower level of intracellular GSH. Alteration in the normal level of MDA and GSH concentration in stressed erythrocytes are indicators of an increased pro-oxidant/antioxidant ratio compared with normal erythrocytes. Our results are consistent with those of previous reports on GSH and MDA concentration in erythrocytes (CitationReglinski et al., 1988; CitationWang et al., 1999; CitationBryszewska et al., 1995). The protective effects of medicinal plant extracts on erythrocyte GSH and MDA concentration from hydrogen peroxide and tert-butyl hydroperoxide-induced oxidative stress may be attributed to the presence of polyphenols, tannins, anthocyanin, and glycosides which either have the capacity to scavenge free radicals or activate antioxidant enzymes or inhibit oxidases (CitationLiu et al., 1992, Citation2006; CitationRice-Evans, 1995; CitationScartezzini & Speroni, 2000; CitationKulkarni et al., 2004; CitationAmin & Hamza, 2005).
High polyunsaturated fatty acid, high cellular concentration of oxygen and hemoglobin in erythrocytes make them highly susceptible for oxidative damage which results in changes in membrane fluidity and inactivation of membrane-bound receptors and enzymes, ionic parameters (CitationMaridonneau et al., 1983), an increase in lipid peroxidation (CitationRohn et al., 1998), oxidation of glutathione and protein sulphydryl group (CitationTelci et al., 2000) and activation of proteolysis (CitationDavies & Goldberg, 1987). Increased erythrocyte MDA concentration decreases the membrane fluidity of the lipid bilayer (CitationBryszewska et al., 1995) which causes the development of long-term complications in diseases like diabetes, hypertension, atherosclerosis, cardiovascular, cancer and neurological disorders (CitationHalliwell & Gutteridge, 1990; CitationKrouf et al., 2003; CitationSiemianowicz et al., 2003).
Based on our observations, we hypothesize that use of medicinal plant extracts as a supplementary/dietary antioxidant in nutraceutical and/or cosmoceutical for protection against complications arising from oxidative stress may not only in part improve the ethnopharmacological knowledge of medicinal plants, but also pave the way for commercial utilization of medicinal plants. It seems worthwhile to mention that plants, which are more exposed to radical-forming radiation processes, are able to produce many types of scavenger molecules, mainly phenolic compounds. Mammals lack the ability to generate phenolic compounds (except estrogens), but this deficiency may be substituted for, in part, by the plants (CitationSalah et al., 1995; CitationSpiteller, 2003). Our observation may also, in part, explain the use of spent/waste part(s) of medicinal plants as an economically viable source of natural and potent antioxidant against complications arising from the oxidative stress.
Conclusion
The aqueous and alcohol extracts of Punica granatum, Caesalpinia bonducella, Hibiscus subdariffa, Moringa oleifera, Garcinia indica, and Matricaria chamomilla significantly protects the erythrocytes against oxidative stress induced by hydrogen peroxide and tert-butyl hydroperoxide. The extracts are capable of protecting erythrocytes against oxidative damage, thereby maintaining the basal levels of GSH and MDA concentration. The protection of erythrocytes GSH and MDA oxidation by medicinal plant extracts may be due to the presence of high amounts of polyphenolics, anthocyanin, tannins, glycosides to which its antioxidant activity may be ascribed. Further investigations on isolation, characterization and identification of active phytoceuticals responsible for the antioxidant activity are warranted for future work.
Acknowledgements
This article was first presented as an abstract during October 2006 at the National Interactive Meet (NIM-2006), CIMAP, Lucknow, India. The work was supported by the Department of Science and Technology (DST), Government of India under the Fast Track Scheme for Young Scientists (SR/FT/L-06/2005). The authors are also grateful to Drs. A.K. Gupta and Dinesh Kumar for providing some of the plant material from the National Gene Bank set up by the Department of Biotechnology (DBT), Government of India and CIMAP (CSIR) for infrastructural facilities.
References
- Amin A, Hamza AA (2005): Hepatoprotective effects of Hibiscus, Rosmarinus and Salvia on azathioprine-induced toxicity in rats. Life Sci 77: 266–278.
- Arora S, Kaur K, Kaur S (2003): Indian medicinal plants as a reservoir of protective phytochemicals. Terato Carcinog Mutagen Suppl 1: 295–300.
- Beutler E, Duron O, Kefly BM (1963): Improved method for the determination of blood glutathione. J Lab Clin Med 61: 882–888.
- Blasiak J, Arabski M, Pertynski T, Malecka-Panas E, Wozniak K, Drzewoski J (2002): DNA damage in human colonic mucosa cells evoked by nickel and protective action of quercetin-involvement of free radicals. Cell Biol Toxicol 18: 279–288.
- Boots AW, Li H, Schins RP, Duffin R, Heemskerk JW, Bast A, Haenen GR (2007): The quercetin paradox. Toxicol Appl Pharmacol 222: 89–96.
- Bryszewska M, Zavodnik IB, Niekurzak A, Szosland K (1995): Oxidative processes in red blood cells from normal and diabetic individuals. Biochem Mol Biol Int 37: 345–354.
- Buettner GR (1993): The pecking order of free radicals and antioxidants: Lipid peroxidation, alpha tocopherol, and ascorbate. Arch Biochem Biophys 300: 535–543.
- Craig WJ (1999): Health-promoting properties of common herbs. Am J Clin Nutr 70: 491S–499S.
- Davies KJ, Goldberg AL (1987): Oxygen radicals stimulate intracellular proteolysis and lipid peroxidation by independent mechanisms in erythrocytes. J Biol Chem 262: 8220–8226.
- Del Bello B, Maellaro E, Sugherini L, Santucci A, Comporti M, Casini AF (1994): Purification of NADPH-dependent dehydroascorbate reductase from rat liver and its identification with 3-alpha-hydroxysteroid dehydrogenase. Biochem J 304: 385–390.
- Deliconstantinos G, Villiotou V, Stavrides JC (1996): Tumour promoter tert-butyl-hydroperoxide induces peroxynitrite formation in human erythrocytes. Anticancer Res 16: 2969–2979.
- Di Simplicio P, Lupis E, Rossi R (1996): Different mechanisms of formation of glutathione-protein mixed disulfides of diamide and tert-butyl hydroperoxide in rat blood. Biochim Biophys Acta 1289: 252–260.
- Di Simplicio P, Cacace MG, Lusini L, Giannerini F, Giustarini D, Rossi R (1998): Role of protein -SH groups in redox homeostasis: The erythrocyte as a model system. Arch Biochem Biophys 355: 145–152.
- Domanski AV, Lapshina EA, Zavodnik IB (2005): Oxidative processes induced by tert-butyl hydroperoxide in human red blood cells: Chemiluminescence studies. Biochemistry 70: 761–769.
- Esterbauer H, Cheeseman KH (1990): Determination of aldehydic lipid peroxidation products: Malondialdehyde and 4-hydroxynonenal. Methods Enzymol 186: 407–421.
- Finkel T, Holbrook NJ (2000): Oxidants, oxidative stress and the biology of ageing. Nature 408: 239–247.
- Gerster H (1993): Anticarcinogenic effect of common carotenoids. Int J Vitam Nutr Res 63: 93–121.
- Halliwell B, Gutteridge JM (1986): Oxygen free radicals and iron in relation to biology and medicine: Some problems and concept. Arch Biochem Biophys 246: 501–514.
- Halliwell B, Gutteridge JM (1990): Role of free radicals and catalytic metal ions in human disease: An overview. Methods Enzymol 186: 1–85.
- Iglesias BF, Catala A (2005): Rat, caprine, equine and bovine erythrocyte ghosts exposed to t-butyl hydroperoxide as a model to study lipid peroxidation using a chemiluminescence assay. Res Vet Sci 79: 19–27.
- Katalinic V, Milos M, Kulisic T, Jukic M (2006): Screening of 70 medicinal plant extracts for antioxidant capacity and total phenols. Food Chem 94: 550–557.
- Konukoglu D, Akçay T, Erdem MT (1998): Susceptibility of erythrocyte lipids to oxidation and erythrocyte antioxidant status in myocardial infarction. Clin Biochem 31: 667–671.
- Krinsky NI, Deneke SM (1982): Interaction of oxygen and oxy-radicals with carotenoids. J Natl Cancer Inst 69: 205–210.
- Krouf D, Bouchenak M, Mohammedi B, Cherrad A, Belleville JL, Prost JL (2003): Changes in serum lipids and antioxidant status in west Algerian patients with essential hypertension treated with acebutolol compared to healthy subjects. Med Sci Monit 9: PI 109–115.
- Kulkarni AP, Aradhya SM, Divakar S (2004): Isolation and identification of a radical scavenging antioxidant – punicalagin from pith and carpellary membrane of pomegranate fruit. Food Chem 87: 551–557.
- Lee SE, Hwang HJ, Ha JS, Jeong HS, Kim JH (2003): Screening of medicinal plant extracts for antioxidant activity. Life Sci 73: 167–179.
- Liu GT, Zhang TM, Wang BE, Wang YW (1992): Protective action of seven natural phenolic compounds against peroxidative damage to biomembranes. Biochem Pharmacol 43: 147–152.
- Liu JY, Chen CC, Wang WH, Hsu JD, Yang MY, Wang CJ (2006): The protective effects of Hibiscus subdariffa extract on CCl4-induced liver fibrosis in rats. Food Chem Toxicol 44: 336–343.
- Lopez-Revuelta A, Sánchez-Gallego JI, Hernández-Hernández A, Sánchez-Yagüe J, Llanillo M (2005): Increase in vulnerability to oxidative damage in cholesterol-modified erythrocytes exposed to t-BuOOH. Biochim Biophys Acta 1734: 74–85.
- Luqman S, Rizvi SI (2006): Protection of lipid peroxidation and carbonyl formation in proteins by capsaicin in human erythrocytes subjected to oxidative stress. Phytother Res 20: 303–306.
- Luqman S, Srivastava S, Darokar MP, Khanuja SPS (2005): Detection of antibacterial activity in spent roots of two genotypes of aromatic grass Vetiveria zizanioides. Pharm Biol 43: 732–736.
- Manda K, Bhatia AL (2003): Role of β-carotene against acetaminophen-induced hepatotoxicity in mice. Nutr Res 23: 1097–1103.
- Maridonneau I, Barquet P, Garray RP (1983): Na+ and K+ transport damage induced by oxygen free radicals in human red cell membranes. J Biol Chem 258: 3107–3113.
- May JM, Cobb CE, Mendiratta S, Hill KE, Burk RF (1998): Reduction of the ascorbyl free radical to ascorbate by thioredoxin reductase. J Biol Chem 273: 23039–23045.
- May JM, Qu ZC, Cobb CE (2004): Human erythrocyte recycling of ascorbic acid: Relative contributions from the ascorbate free radical and dehydroascorbic acid. J Biol Chem 279: 14975–14982.
- Mendiratta S, Qu ZC, May JM (1998): Erythrocyte defenses against hydrogen peroxide: The role of ascorbic acid. Biochim Biophys Acta 1380: 389–395.
- Mueller S, Riedel HD, Stremmel W (1997): Determination of catalase activity at physiological hydrogen peroxide concentrations. Anal Biochem 245: 55–60.
- Musonda CA, Chipman JK (1998): Quercetin inhibits hydrogen peroxide-induced NF-kappa B DNA binding activity and DNA damage in HepG2 cells. Carcinogenesis 19: 1583–1589.
- Ng TB, Liu F, Wang ZT (2000): Antioxidative activity of natural products from plants. Life Sci 66: 709–723.
- Omenn GS, Goodman GE, Thornquist MD, Balmes J, Cullen MR, Glass A, Keogh JP, Meyskens FL, Valanis B, Williams JH, Barnhart S, Hammar S (1996): Effect of a combination of beta carotene and vitamin A on lung cancer and cardiovascular disease. N Engl J Med 334: 1150–1155.
- Peng IW, Kuo SM (2003): Flavonoid structure affects the inhibition of lipid peroxidation in Caco-2 intestinal cells at physiological concentrations. J Nutr 133: 2184–2187.
- Pradhan D, Weiser M, Lumley-Sapanski K, Frazier D, Kemper S, Williamson P, Schlegel RA (1990): Peroxidation-induced perturbations of erythrocyte lipid organization. Biochim Biophys Acta 1023: 398–404.
- Reglinski J, Hoey S, Smith WE, Sturrock RD (1988): Cellular response to oxidative stress at sulfhydryl group receptor sites on the erythrocyte membrane. J Biol Chem 263: 12360–12366.
- Repetto MG, Llesuy SF (2002): Antioxidant properties of natural compounds used in popular medicine for gastric ulcers. Braz J Med Biol Res 35: 523–534.
- Rice-Evans C (1995): Plant polyphenols: Free radical scavengers or chain-breaking antioxidants? Biochem Soc Symp 61: 103–116.
- Rizvi SI, Luqman S (2002): Antioxidative property of capsaicin. Med Chem Res 11: 301–307.
- Rizvi SI, Kumar N (2005): Altered redox status in erythrocytes from hypertensive subjects: Effect of (-) epicatechin. Prog Biochem Biophys 32: 235–238.
- Rizvi SI, Zaid MA, Anis R, Mishra N (2005): Protective role of tea catechins against oxidation-induced damage of type 2 diabetic erythrocytes. Clin Exp Physiol Pharmacol 32: 70–75.
- Rohn TT, Nelson LK, Waeg G, Quinn MT (1998): U-101033E (2,4-diaminopyrrolopyrimidine), a potent inhibitor of membrane lipid peroxidation as assessed by the production of 4-hydroxynonenal, malondialdehyde, and 4-hydroxynonenal-protein adducts. Biochem Pharmacol 56: 1371–1379.
- Salah N, Miller NJ, Paganga G, Tijburg L, Bolwell GP, Rice-Evans C (1995): Polyphenolic flavanols as scavengers of aqueous phase radicals and as chain-breaking antioxidants. Arch Biochem Biophys 322: 339–346.
- Scartezzini P, Speroni E (2000): Review on some plants of Indian traditional medicine with antioxidant activity. J Ethnopharmacol 71: 23–43.
- Seth SD, Sharma B (2004): Medicinal Plants in India. Indian J Med Res 120: 9–11.
- Siemianowicz K, Gminski J, Francuz T, Wojcik A, Posielezna B (2003): Activity of antioxidant enzymes in children from families at high risk of premature coronary heart disease. Scand J Lab Invest 63: 151–158.
- Spiteller G (2003): Are lipid peroxidation processes induced by changes in the cell wall structure and how are those processes connected with disease. Med Hypotheses 60: 69–83.
- Surh Y (1999): Molecular mechanisms of chemopreventive effects of selected dietary and medicinal phenolic substances. Mutat Res 428: 305–327.
- Telci A, Cakatay U, Kayali R Erdogan C, Orhan Y, Sivas A, Akcay T (2000): Oxidative protein damage in plasma of type 2 diabetic patients. Horm Metab Res 32: 40–43.
- Tripathi R, Mohan H, Kamat JP (2007): Modulation of oxidative damage by natural products. Food Chem 100: 81–90.
- Wang X, Wu Z, Song G, Wang H, Long M, Cai S (1999): Effects of oxidative damage of membrane protein thiol group on erythrocyte membrane viscoelasticities. Clin Hemorheol Microcirc 21: 137–146.
- Washburn MP, Wells WW (1999): The catalytic mechanism of the glutathione-dependent dehydroascorbate reductase activity of thioltransferase (glutaredoxin). Biochemistry 38: 268–274.
- Winston JC (1999): Health-promoting properties of common herbs. Am J Clin Nutr 70: 491–499.