Abstract
Preparations of Echinacea (Asteraceae) are frequently consumed for the control and prevention of rhinovirus-induced colds and other respiratory disorders. Since it is now generally believed that the symptoms of rhinovirus colds are due to the enhanced secretion of inflammatory chemokines and cytokines, we decided to analyze the effects of rhinovirus infection and Echinacea treatment [defined extracts of E. purpurea (L.) Moench] on cytokine/chemokine gene expression and protein secretion in a line of human tracheo- bronchial epithelial cells. Among the collection of more than 50 cytokines and chemokines present in the gene arrays, 12 showed significant induction of expression by the virus (> 2-fold), some of them by more than 5-fold. However, not all of these resulted in similar changes in the corresponding proteins, presumably as a consequence of post-transcriptional changes. A total of 16 cytokines, mostly chemokines, showed substantial protein increases, including several, such as the well known pro-inflammatory cytokines IL-6 and IL-8 (CXCL8), which were induced in the absence of additional transcription. These results support the concept that virus-induced multiple inflammatory cytokines are responsible for the cold symptoms. In most cases, one or both Echinacea preparations reversed the viral stimulation, thus providing a basis for the anti-inflammatory properties attributed to Echinacea.
Introduction
Echinacea (Asteraceae) is one of the most popular herbal medicines for the prevention and treatment of colds and flu, and has often been advocated for the alleviation of asthmatic and other respiratory conditions (CitationBarnes et al., 2005; CitationSchoop et al., 2006; CitationShah et al., 2007). However, although more than 100 common cold viruses, the rhinoviruses, have been isolated and characterized, research has shown that these viruses cause little or no cytopathology in nasal tissues in vivo or in epithelial cell lines of nasal or tracheo-bronchial origin (CitationGwaltney, 2002; CitationMosser et al., 2005). These observations have led to the belief that the familiar symptoms of a common cold, the running nose, stuffiness, sneezing, sore throat, and mucus membrane irritation, result indirectly from the ability of these viruses to stimulate the secretion of pro-inflammatory cytokines (CitationMessage & Johnston, 2004; CitationSharma et al., 2006). Evidence also indicates that rhinoviruses can play a role in exacerbating asthmatic, allergic, and other chronic respiratory conditions (CitationJohnston, 2005; CitationSchaller at al., 2006; CitationWark et al., 2007; CitationXatzipsalti et al., 2007; CitationHansbro et al., 2008).
The cytokines comprise a large family of multi-functional proteins, which can be produced and secreted by many cells, including epithelial cells, in response to stimuli such as rhinoviruses and other respiratory viruses (CitationMessage & Johnston, 2004; Schaller et al., 2007). Among this class of proteins is a group of smaller proteins, the chemokines (chemo-attractant cytokines), which share a common property of attracting inflammatory cells, usually neutrophils, eosinophils, or various T-lymphocytes, to the site of an infection or wound (Balestrieri et al., 2008; CitationVandercappelen et al., 2008). More than 50 chemokines and 18 chemokine receptors have been identified in humans (CitationColobran et al., 2007; Balestrieri et al., 2008), and their inflammation-related functions appear to be quite redundant.
We decided to make a detailed analysis of gene expression in rhinovirus infected epithelial cells, by means of a combination of cytokine gene arrays and corresponding protein antibody arrays. Both approaches are necessary to obtain a complete picture since it is well known that gene expression can be controlled at various post-transcription and post-translation stages (CitationFan et al., 2005; CitationProost et al., 2006; CitationGomez-Martin et al., 2008). Consequently, changes in transcription of cytokine genes might not be reflected in corresponding changes of the functional proteins. Our hypothesis was that rhinovirus infection would activate or enhance the expression and secretion of specific inflammatory cytokines or chemokines, and that these events could be reversed by Echinacea treatment.
Materials and methods
Echinacea extracts
Two commercial preparations (E1 and E2) were obtained from North American commercial sources and analyzed for their major constituents. These are the same preparations used in our previous antiviral studies (CitationVimalanathan et al., 2005). E1 was an aqueous expressed juice extract of the aerial parts of E. purpurea [Echinacea purpurea (L.) Moench, University of Ottawa Herbarium accession number UO 19180]. The total extractable polysaccharide content of the pressed juice product was 23.7% w/w. After hydrolysis the monosaccharide content was determined and found to be consistent with a mixture of starch and Echinacea hemicelluloses and AGP (arabinose 8%, galactose 17.1%, glucose 25.4%, mannose 46.7%, rhamnose 2.3%, xylose 2.3%).
E2 was a 50% ethanol tincture, derived from E. purpurea roots (1:9 w/v) (University of Ottawa Herbarium accession number UO19181). This preparation was analyzed by HPLC as described previously, and showed a typical combination of caffeic acid derivatives and alkylamides (CitationBinns et al., 2002). Both extracts were diluted in cell culture medium and stored frozen at -30°C.
Extracts E1 and E2, at the concentrations used, did not show any cytotoxic effects, or other microscopically-visible effects, on the cells. They were devoid of endotoxin activity, as determined by means of a commercial endotoxin test kit (Lonza).
Cell culture and virus
BEAS-2B, a human bronchial epithelial cell line, and the rhinovirus-sensitive H-1 derivative of HeLa cells, were both obtained from American Type Culture Collection (ATCC, Rockville, MD). The standard growth medium was Dulbecco’s MEM with 5-10% fetal bovine serum (endotoxin-free). All culture reagents were obtained from Invitrogen (Ontario). For experimental purposes BEAS-2B cells were used, at 90-100% confluency, between passages 25 and 40. All cultures were maintained in a 5% CO2 : 95% air atmosphere, at 37°C or, in the case of RV-infected cultures, 34°C. Rhinovirus type 14 (RV 14) was obtained from ATCC, and was propagated and assayed, by plaque formation, in monolayers of H-1 cells, as described previously (CitationSharma et al., 2006).
Infection and treatment of BEAS-2B cells
BEAS-2 cells were grown to 90%-100% confluency in 75 cm2 flasks. Medium was removed and replaced with 2.0 mL of fresh medium without serum, containing the appropriate amount of stock RV (moi = 0.4 pfu/cell), or medium only. The flasks were incubated on a shaker platform at 34°C for 1 h. Unadsorbed virus and media were removed and the cells washed once with 5 mL medium without serum. Fresh medium was then added, 10 mL per flask, containing where appropriate 100 μg/mL of E1 or 50 μg/mL of E2. These concentrations were non-cytotoxic (CitationSharma et al., 2006).
DNA microarray analysis
Complete details are described elsewhere (CitationAltamirano-Dimas et al., 2007). Briefly, total
RNA was extracted from cells 18 h after infection, by the Trizol® technique, and purified from uninfected and RV14-infected BEAS-2B cells with or without extract E1 or E2. The mRNAs were reverse transcribed into Cy5 (cyanin dye #5) labeled cDNAs, and hybridized, together with Cy3 (cyanin dye #3) labeled UHR cDNA (derived from Universal Human Reference RNA), with DNA microarrays (Prostate Research Centre, Vancouver Hospital) containing a total of 13,816 human gene-specific oligomers, plus 536 controls, in duplicate.
Each experimental sample was compared directly with the reference sample on the same array. The arrays were scanned after hybridization, and the data normalized and statistically analyzed as described below.
Statistical analysis of arrays
The arrays were scanned after hybridization with the ScanArray analizer laser scanner (Perkin Elmer). Independent grayscale images, 16-bit TIFF images, were generated for each pair of samples to be compared. These images were analyzed to identify the arrayed spots and to measure the relative fluorescence intensities for each element. Signal median intensities and background correction were quantitated using the computer program Imagene 5.6. Signal intensities of each one of the 28,704 spots in the microarray were plotted. Only experiments in which 80% of the spots were present and the ratio of signal to noise was higher than 3 in at least 40% of the total number of spots were selected.
Gene expression was evaluated with the aid of GeneSpring 7.3.1 program. The intensity of every spot in the microarray was compared with the intensity of the Universal Human Reference RNA (two color experiments) in order to obtain a signal ratio. Normalization was performed for each spot. Normalized data were subjected to statistical analysis of variance (ANOVA).
The procedure one way ANOVA was used to filter out genes that did not vary significantly across different groups with multiple samples. This allowed us to find those genes that exhibited important changes between various conditions of the experiment. This comparison was performed for each gene, and the genes with sufficiently small p-values were returned. Comparisons were performed using parametric methods, variances not assumed equal (Welch ANOVA) and p-value cut-off at 0.05.
Clustering algorithms were used to divide genes into groups that have similar expression patterns. K-means clustering was used to divide genes into groups based on their expression patterns. The goal was to produce groups of genes with a high degree of similarity within each group and a low degree of similarity between groups. K-means clusters were constructed so that the average behavior in each group was distinct from any of the other groups.
Principal component analysis (PCA) was used as a decomposition technique that produced a set of expression patterns known as principal components. Linear combinations of these patterns were assembled to represent the behavior of all of the genes in a given data set.
The phylogenetic tree or dendrogram was constructed in order to view the results of hierarchical clustering. In such a tree, genes having similar expression patterns were clustered together.
The pathway views were used to display and place genes on an imported .gif or .jpeg image. We used the biochemical pathways imported from the database Kyoto Encyclopedia of Genes and Genomes (KEGG) and gene maps from GenMapp2 Pathways to see the expression values in relationship with other proteins in a pathway.
The normalized values obtained from GeneSpring analysis were used to generate the normalized ratios of E1/C, E2/C, RV/C, RVE1/RV, RVE2/RV and loaded in to the program Ingenuity Pathway analysis (IPA 4.0) from Ingenuity Systems Inc. (Redwood City, CA). The genes were analyzed by function and those related to Immune Response were selected. Those genes with a ratio between 1.5 and -1.25 were excluded.
Cytokine antibody arrays
Panomics TransSignal human antibody arrays 3.0, MA 6160 (Panomics, Inc. Redwood City, CA) were used. Cell-free supernatants were taken 48 h after infection and measured without further treatment, according to the Manufacturer’s instructions. Complete details were provided in CitationSharma et al. (2006). Following a thorough wash of the array membranes, the signals were imaged with enhanced chemiluminescence (ECL) in a Bio-Rad Fluor-S Max Multi-imager (Bio-Rad, Hercules, CA), and signal intensities (average of two identical spots) were quantified by means of the Bio-Rad “Quantity One’ software. Results were reproducible on duplicate membranes.
In several cases, Raybiotech Quantibody arrays were used to confirm the effects of virus infection on the secretion of specific cytokines.
Results
CXC chemokines
The chemokines can be conveniently divided into several sub-groups, depending on their chromosomal location (many are clustered on chromosome 17, while another cluster is found on chromosome 4), their detailed structures and relative sequence homology (most of them have either the CC or CXC motif), and functional aspects (chemo-attraction of predominantly neutrophils, eosinophils, or T-lymphocytes) (CitationColobran et al., 2007; Balestrieri et al., 2008; Vandercappelen et al., 2008).
shows the transcription ratios for four CXC chemokines located close together on chromosome 4q, CXCL 9 (Mig), CXCL 10 (IP-10), CXCL11 (I-TAC), and CXCL13 (BCA; BLC/BCA-1). This group tends to chemo-attract T-cells, but they are not angiogenic, i.e., do not promote angiogenesis (CitationBalestrieri et al., 2008), and they do not possess the amino acid sequence motif ELR. However, it is evident from that this apparent similarity is not reflected in their gene responses to the various treatments. The virus/control ratios (V/C) varied from > 20 for CXCL11 (I-TAC), indicating substantial up-regulation of that gene, to slight down-regulation of CXCL13 (BCA). However, E1 and E2, expressed as VE1/V and VE2/V, respectively, both reversed the viral stimulation, indicating anti-inflammatory activities of the two Echinacea preparations. The effects of E1 and E2 on control, uninfected cells (CE1/C and CE2/C in ) were quite variable, indicating the variation in individual gene responses and the differences between the two extracts. For comparison, CXCL12 (SDF-1α/β), which is not part of this cluster of genes, is also shown in
Figure 1. Relative gene transcription of CXCL chemokines (ELR-) 9-13. BEAS- 2B cells, in 75 cm 2 flasks, uninfected or infected with rhinovirus type 14, were treated with Echinacea extract E1 or E2, or medium only, for 18 h. Total RNA was extracted, converted into cDNA and hybridized to DNA arrays containing 13,816 genes in duplicate. Data analysis was carried out as described in Materials and Methods and the normalized values from three separate experiments were converted into ratios: V/C = virus/control; VE1/V = virus + E1/virus; VE2/V = virus + E2/virus; CE1/C = control + E1/control; CE2/C = control + E2/control.
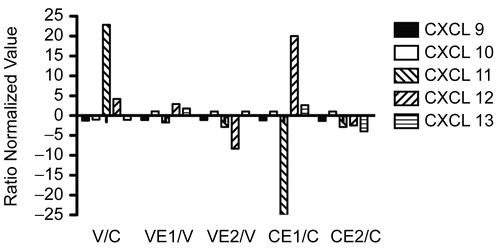
Corresponding protein data were only available for CXCL10, and these are shown in . In spite of the lack of changes in transcription of this chemokine, there was a small but significant up-regulation of protein secretion, which was reversed by both E1 and E2.
Table 1. CXCL chemokines.
shows the transcription ratios for 5 ELR+ CXC chemokines, most of which are angiogenic, CXCL1 (GROα), CXCL2 (GROβ), CXCL3 (GROγ), CXCL5 (ENA78), and CXCL8 (also referred to as IL8, interleukin 8). There was relatively little effect of the virus itself, but in general there was a marked stimulation by both E1 and E2 in uninfected cells, and this was also reflected in the virus + extract treated cells. However, the responses of each gene to E1 and E2 were quite different.
Figure 2. CXCL chemokines 1, 2, 3, 5 and 8 (ELR+), relative gene transcription. BEAS- 2B cells, in 75 cm2 flasks, uninfected or infected with rhinovirus type 14, were treated with Echinacea extract E1 or E2, or medium only, for 18 h. Total RNA was extracted, converted into cDNA and hybridized to DNA arrays containing 13,816 genes in duplicate. Data analysis was carried out as described in Materials and Methods and the normalized values from three separate experiments were converted into ratios: V/C = virus/control; VE1/V = virus + E1/virus; VE2/V = virus + E2/virus; CE1/C = control + E1/control; CE2/C = control + E2/control.
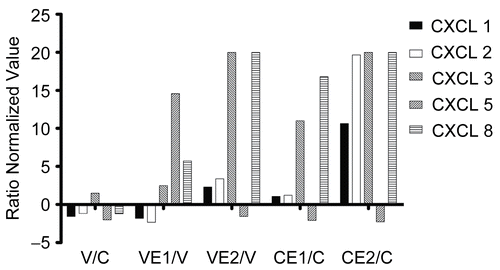
Protein data were available for only CXCL1 and 8, and these are shown in . For CXCL8 (IL-8), the virus showed substantial stimulation in protein production, and this was reversed by the Echinacea extracts. The CXCL1 protein was not affected by the virus, although down-regulation by both E1 and E2 occurred. The effects on uninfected cells were small and did not reflect the gene transcription changes. Thus, in general, there was a lack of correlation between gene transcription and the final products.
CC chemokines
Most of the CC chemokines are clustered on chromosome 17, and many of them chemo-attract neutrophils and/or eosinophils, in addition to various other functions. shows the gene array results for CCL2 (MCP-1), CCL8 (MCP-2), CCL3 (MIP-1α), CCL4 (MIP-1β), CCL5 (RANTES), CCL11 (eotaxin), CCL17 (TARC) and CCL18 (PARC, MIP-4), expressed as ratios as before. In all cases, except CCL11, the virus stimulated transcription, especially CCL 17 and 18, and in most cases this was reversed by E1 and/or E2. The effects on uninfected cells, however, were quite variable. shows the corresponding data for the protein products, when these were available. In general, the protein ratios resembled the corresponding gene changes, although the magnitude of the protein changes was much less pronounced. Thus, for many of the CC chemokines transcriptional changes were reflected in corresponding, but muted, protein changes.
Figure 3. CCL chemokines 2-5, 11, 17 and 18. BEAS- 2B cells, in 75 cm2 flasks, uninfected or infected with rhinovirus type 14, were treated with Echinacea extract E1 or E2, or medium only, for 18 h. Total RNA was extracted, converted into cDNA and hybridized to DNA arrays containing 13,816 genes in duplicate. Data analysis was carried out as described in Materials and Methods and the normalized values from three separate experiments were converted into ratios: V/C = virus/control; VE1/V = virus + E1/virus; VE2/V = virus + E2/virus; CE1/C = control + E1/control; CE2/C = control + E2/control.
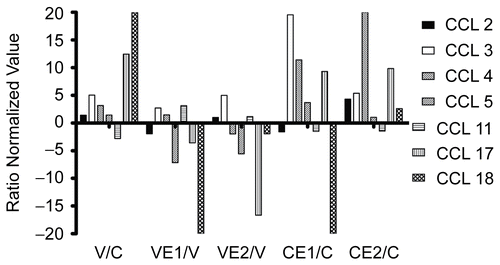
Table 2. CCL chemokines, chromosome 17.
Interleukin clusters
Interleukin 1 α and β have long been recognized as pro-inflammatory cytokines. The genes are part of a large cluster of related cytokines on chromosome 2, now classified as IL1 family members 1-11, IL1F1 – IL1F11 (CitationBarksby et al., 2007). Some of these, together with a few related proteins such as IL1 receptor 1 and caspase 1 (IL1β convertase), were available on the gene arrays. The data are shown in . Protein data were also available for IL1 α and β, and their receptor antagonist IL1ra. The virus down regulated the genes for IL1 α and β, while IL1 RA was induced. In contrast, both IL1 β and IL1ra proteins were induced. Both E1 and E2 down-regulated IL1 α and β genes in uninfected cells, but these changes were not reflected in corresponding protein changes.
Table 3. Interleukin-1 family.
The other IL1 family members, as well as caspase 1, showed variable but small changes in response to virus infection, and generally a down-regulation in uninfected cells exposed to Echinacea.
Chromosome 5q31 contains a cluster of cytokines, IL4, IL5, IL13 and GM-CSF. Their functions, however, appear to be unrelated, and this is reflected in their gene array and protein responses, shown in . IL4 and 5 showed significant up-regulation in virus-infected cells, which was generally inhibited by both E1 and E2, although the corresponding protein changes were smaller. In contrast, IL13 and GM-CSF were down- regulated by the virus, and somewhat up-regulated by E1 and E2. Thus, there was little relationship between the members of this cluster at the transcript level, but at the protein level they were similar.
Table 4. Cytokine cluster: chromosome 5q31.
Other inflammation-related cytokines
A number of other interleukins, and unrelated proteins, have been implicated in various inflammatory conditions, either as pro-inflammatory mediators or as anti-inflammatory regulators (Janeway et al., 2006). Gene array data and corresponding protein data are shown in . The IL6 protein was substantially induced by virus, in confirmation of several previous reports (CitationMessage & Johnston., 2004; CitationSharma et al., 2006; CitationEdwards et al., 2007), although gene transcription was not significantly affected (, row 1). Both E1 and E2 inhibited the protein secretion, but transcription was little affected. IL10 (an anti-inflammatory cytokine) and IL12 showed relatively small responses in terms of gene transcription and protein production.
Table 5. Cytokines involved in inflammatory conditions.
IL16, which has been implicated in asthma and allergic disorders (CitationArima et al., 1999; CitationDeng & Shi, 2006), responded to the virus with marked stimulation at the gene level (), which was inhibited strongly by both E1 and E2. We did not have protein data for this cytokine. IL17 also showed a significant virus-induction at the protein level, but not at the gene level ().
TNFα, which has been implicated as a co-inducer of rhinovirus-induced chemokine expression and airway inflammation (CitationNewcomb et al., 2007), showed a marked induction in transcription by the virus itself, but this was not reflected by protein production (), in agreement with the report by CitationGertsch et al. (2004). Echinacea showed a down-regulation of TNFα. In general the protein changes were considerably less pronounced than transcription changes, as we observed for some of the chemokines mentioned above. FAS ligand, a member of the TNF family, showed smaller effects ().
The anti-inflammatory TGFβ and the other cytokines showed relatively small changes in transcription and protein production, both in response to the virus and in response to the Echinacea extracts ().
A number of other interleukins were also represented on the gene arrays. These were IL- 2, 3, 7, 9, 11, 13, 14, 15, 19 and 20. Their virus/control ratios varied from 0.15 (IL-19) to 2.13 (IL-3), with relatively small changes in the other ratios, and protein levels, when available, also showed minor changes.
shows a summary of those cytokines/chemokines induced by the virus, at either the gene or protein level, and their modulation by E1 and E2.
Table 6. Summary of cytokines showing modulation.
Receptors
A total of eight chemokine receptors and a variety of interleukin receptors were represented on the gene arrays. In general, most of the changes in response to virus or Echinacea were relatively small, and furthermore there were no apparent correlations between receptors and the corresponding ligands.
Discussion
The objectives of this study were:
to determine how widespread were the effects of rhinovirus infection on host genes, especially those genes involved in inflammatory responses (cytokines and chemokines);
to examine the effects of the two distinct Echinacea extracts on these virus-induced changes, reversal of virus induction indicating an anti-inflammatory effect;
to examine the effects of the Echinacea extracts on uninfected cells;
to determine to what extent the effects shown by virus and extracts were reflected in changes in the production of the corresponding proteins, an absence of correlations indicating the likelihood of post- transcriptional controls;
to consider how these results can be used to explain the benefits of Echinacea consumption. The cell culture-virus model was chosen in order to reflect the main purpose of Echinacea consumption, which is to control or alleviate the symptoms of common colds caused by rhinovirus. Consequently, the principal target cells are epithelial cells in the tracheo-bronchial and nasal tissues.
We and others have reported previously that RV can bring about substantial changes in gene expression in human airway epithelial cells (CitationMessage & Johnston, 2004; CitationHudson & Altamirano-Dimas, 2006; CitationKatz et al., 2006; CitationChen et al., 2006; CitationAltamirano-Dimas et al., 2007), and it is clear from those results and the present data that many of the gene changes include genes involved in innate immune responses, particularly chemokines and other cytokines. Among the collection of cytokines for which we have presented data here, 14 of them were significantly induced by the virus, some of them by more than 5-fold (summarized in ). However, not all of these resulted in similar changes in the corresponding protein; a total of 13 showed substantial protein increases, including a few proteins that were induced in the absence of additional transcription. The latter situation was particularly evident for the well-known pro-inflammatory cytokines IL-6 and IL-8 (CXCL8), which we and others have reported to be substantially induced in cells of tracheo-bronchial origin (CitationMessage & Johnston, 2004; CitationSharma et al., 2006; CitationEdwards et al., 2007). In both of these cases, transcription was barely affected by virus or by Echinacea, all of the effects shown being manifest at the level of protein secretion. However, this should not be surprising in view of the known complex regulatory pathways operating post-transcriptionally and post-translationally (CitationFan et al., 2005; CitationProost et al., 2006; CitationGomez-Martin et al., 2008). In fact, IL-8 (CXCL8) mRNA stability and protein production has been shown to be subject to a variety of such controls (CitationFan et al., 2005). Consequently, in these instances the gene array results could give rise to misleading interpretations.
For this reason it is difficult to draw conclusions about the cytokine IL-16, which has been associated with asthma and allergic conditions (CitationDeng & Shi, 2006). If the gene array results presented here reflect protein changes, then this would suggest that not only does RV14 induce this cytokine, but that the Echinacea preparations could prove useful in the treatment or amelioration of such conditions. This will have to be confirmed by means of ELISA type tests in the future, since IL-16 was not present on our cytokine antibody arrays. However, the chemokine CCL18 (PARC) showed the highest response of all the cytokines to RV infection, and this level was inhibited strongly by both E1 and E2, yet the corresponding changes in protein levels were relatively small (). Consequently, we have to conclude that the gene array data are not necessarily good predictors of protein production, even though for some cytokine clusters, such as the group containing CCL-2, 3, 4, 5 (), there seemed to be reasonable agreement.
In regard to the question of Echinacea as a modulator of immune responses induced by the virus, the gene array data were not necessarily informative; the protein data were again more convincing. For example, CXCL8 transcription was substantially elevated by both E1 and E2 in virus-infected and uninfected cells, but the protein data indicate strong inhibition of the virus-induced stimulation ().
The IL-1 family of cytokines, which includes IL-18, generally showed unremarkable responses to virus and to Echinacea (), both at the transcription and protein levels. The other cytokine cluster, containing IL-4, 5, 13 and GM-CSF, also gave relatively small changes overall, although the patterns of transcription response differed widely. The other non-interleukin inflammatory genes also showed few significant responses (), except for the anomalous results for TNFα, for which the high stimulations in transcription seen in virus and Echinacea treated cells were not reflected in corresponding protein changes, in agreement with CitationGertsch et al. (2004).
Chemokines utilize at least 15 receptors on their target cells, and there is considerable redundancy in their usage, such that a specific chemokine could use several different receptors and a specific receptor could bind several different chemokines. Our gene arrays contained seven CC receptors (CCR 1 – 5, 8, 9) and CXCR 1. Their V/C ratios ranged from 0.09 (CCR3) to 4.82 (CCR9), but, in any case, a relationship might not be significant because these values would reflect transcription and expression of receptors on the source epithelial cells, rather than on the target cells (mostly leukocytes).
Data were also available for a number of interleukin receptors, but again, for the same reasons, most of the changes were small and of dubious significance to the overall control of inflammatory conditions.
summarizes the genes for which virus infection resulted in significant induction of either transcription or protein production, and their modulation by the Echinacea extracts. The two Echinacea preparations did not show the same pattern of effects in this virus-cell system; but this is not surprising in view of the known significant differences in chemical composition between these two types of preparation, E1 being an aqueous extract of the above ground parts of the plant, while E2 was an ethanol extract of the dried roots (CitationBinns et al., 2002).
Since we were interested primarily in inflammatory responses to the virus, then we have not discussed cases of viral down-regulation of gene expression, although some examples of this were observed. However, this aspect is relevant to Echinacea consumers, based upon the presumption that certain components of Echinacea extracts are capable of neutralizing the virus-induced elevation in secreted chemokines and other cytokines, the latter being responsible for the myriad symptoms that accompany common colds. These results also suggest that Echinacea extracts could be beneficial in treating virus-induced asthmatic and allergic conditions.
Declaration of interest: The authors report no conflicts of interest. The authors alone are responsible for the content and writing of the paper.
References
- Altamirano-Dimas M, Hudson JB, Cochrane D, Nelson C, Arnason JT (2007): Modulation of immune response gene expression by Echinacea extracts: Results of a gene array analysis. Can J Physiol Pharmacol 85:1091–1098.
- Arima M, Plitt J, Stellato C, Bickel C, Motojima S, Makino S, Fukuda T, Schleimer RP (1999): Expression of interleukin-16 by human epithelial cells. Am J Respir Cell Mol Biol 21: 684–692.
- Balestrieri ML, Balestrieri A, Mancini FP, Napoli C (2008): Understanding the immunoangiostatic CXC chemokine network. Cardiovasc Res 78: 250–256.
- Barksby HE, Lea SR, Preshaw PM, Taylor JJ (2007): The expanding family of interleukin-1 cytokines and their role in destructive inflammatory disorders. Clin Exper Immunol 149: 217–225.
- Barnes J, Anderson LA, Gibbons S, Phillipson JD (2005): Echinacea species (Echinacea angustifolia (DC.) Hell. Echinacea pallida (Nutt.) Nutt., Echinacea purpurea (L.) Moench: A review of their chemistry, pharmacology and clinical properties. J Pharm Pharmacol 57: 929–954.
- Bauer R (1998): Echinacea: Biological effects and active principals. In: Lawson LD, Bauer R, eds., Phytomedicines of Europe: Chemistry and Biological Activity. ACS symposium series 691. Washington, D.C., American Chemical Society, pp. 140–157.
- Binns SE, Livesey JF, Arnason JT, Baum BR (2002): Phytochemical variation in Echinacea from roots and flowerheads of wild and cultivated populations. J Agric Food Chem 50: 3673–3687.
- Chen Y, Hamati E, Lee P-K, Lee W-M, Wachi S, Schnurr D, Yagi S, Dolganov V, Boushey GH, Avila P, Wu R (2006): Rhinovirus induces airway epithelial gene expression through double stranded RNA and IFN-dependent pathways. Am J Respir Cell Mol Biol 34: 192–203.
- Colobran R, Pujol-Borrell R, Armengol MP, Juan M (2007): The chemokine network. 1. How the genomic organization of chemokines contains clues for deciphering their functional complexity. Clin Exp Immunol 148: 208–217.
- Deng JM, Shi HZ (2006): Medical progress: Interleukin-16 in asthma. Chin Med J 119: 1017–1025.
- Edwards MR, Hewson CA, Laza-Stanca V, Lau HTH, Mukaida N, Hershenson MB, Johnston SL (2007): Protein kinase R, IkB kinase b, and NFkB are required for human rhinovirus induced pro-inflammatory cytokine production in bronchial epithelial cells. Molecular Immunology 44: 1587–1597.
- Fan J, Heller NM, Gorospe M, Atasoy U, Stellato C (2005): The role of post-transcriptional regulation in chemokine gene expression in inflammation and allergy. Eur Respir J 26: 933–947.
- Gertsch J, Schoop R, Kuenzle U, Suter A (2004): Echinacea alkylamides modulate TNFα gene expression via cannabinoid receptor CB2 and multiple signal transduction pathways. FEBS Journal Letters 577: 563–569.
- Gomez-Martin D, Diaz-Zamudio M, Alcocer-Varela J (2008): Ubiquitination system and autoimmunity: The bridge towards the modulation of the immune response. Autoimmunity Rev 7: 284–290.
- Gwaltney JM (2002): Clinical significance and pathogenesis of viral respiratory infections. Am J Med 112: 13S–18S.
- Hansbro NG, Horvat JC, Wark PA, Hansbro PM (2008): Understanding the mechanisms of viral induced asthma: New therapeutic directions. Pharm Thera 117: 313–353.
- Hudson J, Altamirano M (2006): The application of DNA micro-arrays (gene arrays) to the study of herbal medicines. J Ethnopharmacol 108: 2–15.
- Johnston SL (2005): Overview of virus-induced airway disease. Proc Am Thorac Soc 2: 150–156.
- Katz S, Harris R, Lau J, T-Y, Chau A (2006): The use of gene expression analysis and proteomic databases in the development of a screening system to determine the value of natural medicinal products. eCAM 3: 65–70.
- Message SD, Johnston SL (2004): Host defense function of the airway epithelium in health and disease clinical background. J Leukoc Biol 75: 1–13.
- Mosser AG, Vrtis R, Burchell L, Lee WM, Dick CR, Weisshaar E, Bock D, Swenson CA, Cornwell RD, Meyer KC, Jarjour NN, Busse WW, Gern JE (2005): Quantitative and qualitative analysis of rhinovirus infection in bronchial tissues. Amer J Resp Critical Care Medicine171:645–651.
- Newcomb DC, Sajjan US, Nagarkar DR, Goldsmith AM, Bentley JK, Hershenson MB (2007): Cooperative effects of rhinovirus and TNFα on airway epithelial cell chemokine expression. Am J Phsiol. Lung Cell Mol Physiol 293: L1021–L1028.
- Proost P, Struyf S, Van Damme J (2006): Natural post-translational modifications of chemokines. Bioch Soc Trans 34: 997–1001.
- Schaller M, Hogaboam CM, Lukacs N, Kunkel SL (2006): Respiratory viral infections drive chemokine expression and exacerbate the asthmatic response. J Allergy Clin Immunol 118: 295–302.
- Schoop R, Klein P, Suter A, Johnston SL (2006): Echinacea in the prevention of induced rhinovirus colds: A meta-analysis. Clin Therapeutics 28: 174–183.
- Shah SA, Sander S, White M, Rinaldi M, Coleman C (2007): Evaluation of Echinacea for the prevention and treatment of the common cold: A meta-analysis. Lancet Infect Dis 7: 473–480.
- Sharma M, Arnason JT, Burt A, Hudson JB (2006): Echinacea extracts modulate the pattern of chemokine and cytokine secretion in rhinovirus-infected and uninfected epithelial cells. Phytotherapy Res 20: 147–152.
- Vandercappelen J, Van Damme J, Struyf S (2008): The role of CXC chemokines and their receptors in cancer. Cancer lett 267: 226–244.
- Vimalanathan S, Kang L, Treyvaud Amiguet V, Livesey J, Arnason JT, Hudson J (2005): Echinacea purpurea aerial parts contain multiple antiviral compounds. Pharm Biol 43:740–745.
- Wark PAB, Bucchieri F, Johnston SL, Gibson PG, Hamilton L, Mimica J, Zummo G, Holgate SG, Attia J, Thakkinstian A, Davies DE (2007): IFNγ-induced protein 10 is a novel biomarker of rhinovirus induced asthma exacerbations. J Allergy Clin Immunol 120: 586–593.
- Xatzipsalti M, Psarros F, Konstantinou G, Gaga M, Gourgiotis D, Saxoni-Papageorgiou P, Papadopoulos NG (2007): Modulation of the epithelial inflammatory response to rhinovirus in an atopic environment. Clin Exper Allergy 38: 466–472.