Abstract
In this study we attempt to investigate the new role of docosahexaenoic acid (DHA) supplementation on cognitive ability in aged mice. Kunming-line mice were treated with DHA (200, 400 mg/kg body weight/day) for 30 successive days. The cognitive ability of mice was assessed by learning and memory behavioral test; the levels of DHA were assessed by capillary gas chromatography; the levels of dopamine (DA), norepinephrine (NE), and 5-hydroxytryptamine (5-HT) were assessed by high-performance liquid chromatography; the levels of brain-derived neurotrophic factor (BDNF) and nerve growth factor (NGF) proteins were assessed by the enzyme-linked immunosorbent assay method. The results showed the cognitive ability of mice were significantly different between the DHA-treated groups and the aged group, and orally administered DHA can increase the levels of DA, NE and 5-HT, the content of BDNF and NGF proteins in hippocampus, frontal cortex and striatum tissues. In addition, aged-related changes in phospholipid DHA content were seen. Decreases in DHA were mostly corrected by DHA supplementation. These novel data suggest that DHA supplementation can improve the cognitive dysfunction due to aging by increasing the levels of BDNF and NGF protein and the levels of DA, NE, 5-HT to some extent. We speculate that the mechanism of DHA on cognitive ability may have a beneficial effect on signaling networks through modulation of monoamine neurotransmitters and neurotrophic factors in brain aging.
Introduction
Docosahexaenoic acid (DHA) is the most important omega-3 fatty acid in the brain (CitationHashimoto et al., 2002). It has been demonstrated that an increased intake of DHA in the diet influenced brain functions, such as improving cognitive dysfunction (CitationCatalan et al., 2002), impaired attention (Lim & Suzuki, 1997) and poor flexibility behavior (Yamada et al., 2002). Moreover, DHA in the brain is known to show an age-dependent decrease (CitationMcGahon et al., 1999). Therefore, DHA supplementation is very important to old people.
Previous research has shown that metabolism of monoamines neurotransmitters significantly changes with aging, such as reduction of dopamine (DA) and norepinephrine (NE) in cerebrum and brain stem of aged rats, etc. (CitationBarili et al., 1998; CitationLee et al., 2001). It has indicated that the levels of DA, NE, and 5-hydroxytryptamine (5-HT) decrease throughout the brain: young rats (3–6 months old), as compared to aging rats (15 months or older), display higher monoamine levels in striatum, entorhinal cortex, hippocampus, frontal cortex (CitationLuine et al., 1990; CitationTanila et al., 1994; CitationMiguez et al., 1999). Although recent findings suggest that some of the neurochemical alterations resulting from low brain DHA levels during development can be reversed by increasing brain DHA content (CitationKodas et al., 2002), the effects of DHA on monoamine neurotransmitters still remained unclear.
Trophic factors are a class of molecules that foster the growth, maintenance, and survival of cells. It has been suggested that chronic neurological diseases as well as acute brain injuries could be treated with trophic factors such as nerve growth factor (NGF) and brain-derived neurotrophic factor (BDNF). However, poor penetration of the blood2brain barrier and the occurrence of side effects limit the use of exogenous applications of these factors. Therefore, stimulating the local production of trophic factors seems to be a more attractive approach (CitationCiccarelli et al., 2001). Previous research has shown that hippocampus neurons synthesize NGF (CitationAcsa´dy et al., 2000) and DHA deficiency leads to a decrease NGF content in the hippocampus (CitationIkemoto et al., 2000), and it may be proposed that a reduction in neuronal DHA could decrease NGF (or other factors) in these cells. Besides, it has been reported that the anti-inflammatory role of omega-3 fatty acids may influence BDNF in depression (CitationLogan, 2003). So, it seems that DHA is a good stimulus to up-regulate trophic factors.
Considering that monoamine neurotransmitters and trophic factors play an important role in learning and memory system, accordingly, we performed this experiment to evaluate the effect of DHA on the monoamine neurotransmitters (DA, NE, 5-HT) and trophic factors (NGF, BDNF) in three brain areas of aged mice: hippocampus, frontal cortex and striatum tissues, and to establish whether dietary supplementation of DHA could rectify age-related changes in monoamine neurotransmitters and trophic factors.
Materials and methods
Animals
Thirty aged female mice (22-month Kunming-line, 54 ± 3 g, originally introduced from Swiss mice at the Hoffkine Institute, India in 1944) and 10 young female mice (3-month Kunming-line, 22 ± 2 g) were purchased from the Animal Experimental Center of Sichuan (Chengdu, China). The mice were housed in a controlled environment with temperature maintained at 23° ± 2°C and humidity at 42% ± 2% under a 12:12 h light/dark cycle, with free access to water and food. The animals were treated according to the guidelines of the National Institute of Health Guide for the Care and Use of Laboratory Animals and the Animal Ethics Committee of the University.
Animal treatment
After a week of adaptation, mice were randomly divided into four experimental groups, young group: 3-month young female mice, n = 10, aged group: 22-month aged female mice, n = 10, and two DHA-treated groups: low dose group, n = 10, 22-month aged mice treated with DHA 200 mg/kg body weight/day; high dose group, n = 10, 22-month aged mice treated with DHA 400 mg/kg body weight/day, n = 10. Administered docosahexaenoic acid was dissolved in 5% gum arabic solution, pipetted into light-protecting glass vials under a nitrogen gas flow intended to diminish their auto-oxidation, and kept at 280°C until given via oral gavages for 30 successive days. In order to eliminate the effects of daily gavages and rule out an effect of the treatment on motor or muscular capacity of the animal, young group and aged group received a similar volume of corn oil, respectively. The experiments were performed 3 times.
Behavioral experiments
The behavior experiments were carried out on the second day when DHA treatment was finished. The step-through test was performed first, followed by the water-maze test.
The step-through test (one trial passive avoidance)
The step-through test was performed in the JZZ94 multifunction passive avoidance apparatus (PA M1 O’Hara & Co., Ltd., Tokyo, Japan). The apparatus consists of two compartments separated by a black wall with a hole in the lower middle part. One of the two chambers is illuminated and the other is dark. The test was conducted for two consecutive days, including one training trial (day 1). Each mouse was placed in the illuminated compartment, facing away from the dark compartment for 5 min to be habituated to the apparatus, then it received the training trial for 1 h. The training trial is similar to the adaptation trial, except that when the mouse enters the dark compartment it experiences electric foot shock (DC, 0. 3 mA, 30 V, 1 s) through the stainless steel grid floor. In the testing trial (day 2), the same procedure was repeated. The interval time between placement in the illuminated compartment and entry into the dark compartment was regarded as the memory retention period (time). The entering events per testing trial were recorded as the error counts (number of errors). Mice were allowed to stay in the illuminated compartment for 30 s in the training trial and 5 min in the testing trial.
Passageway water maze test
The passageway water maze was provided by the Pharmacological Institute of Chinese Academy of Medical Sciences. A rectangular maze (73 × 42 × 20 cm, width/length/height) was made of grey Plexiglass and consisted of vertical panels inside (). In the maze, there are one safe area with a stairway under the water, three start areas (A, B, C) with movable guillotine doors, and four error areas (1, 2, 3, 4; corners deviated from the correct pathways) as dead ends in the maze. The maze was filled with water 10 cm deep and kept at a temperature of 23 ± 2°C. There was a light hung 2 m above the center of the maze.
Figure 1. Diagram of the passageway water maze. Mice were individually placed in the start area (A, B and C) with the head toward the wall during training. They started training from A on day 1, from B on day 2, from C on day 3–5. There are 4 error areas (1, 2, 3 and 4) which are dead ends in the maze. Stair: the underwater stairway is a safe area where the mice can climb out from the water. The maze is filled with water (23 ± 2°C) 10 cm in depth.
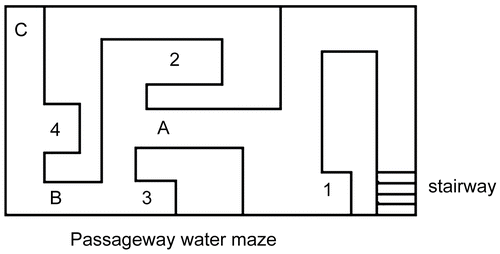
On day 1, the guillotine door of A was closed. Each mouse was individually placed into the start area A with its head toward the wall and allowed to acclimatize to the safe area with the underwater stairway three times. The maximum time for each trial was 2 min. Then each mouse was returned to its cage after the acclimation.
On day 2, the guillotine door of B was closed, while the door of A was open. Each mouse was individually placed into B with its head toward the wall. Each mouse was returned to its cage after it arrived at the safe area. The mouse was returned to its cage if it couldn’t find the safe area within 2 min.
On day 3, the guillotine doors of A and B were raised. Each mouse was placed into C with its head toward the wall. The training session ended after the mouse reached the safe area and climbed out from the water. The mouse would be returned to its cage if it couldn’t find the safe area within 2 min.
On days 4-6, each mouse was placed into C with its head toward the wall. The guillotine doors of A and B were raised. The escape latency period (in seconds) for the mouse arriving at the safe area and the number of errors (mouse head enters error areas) were recorded. In the training and test sessions, the mice were removed from the maze and returned to their cages by the experimenter if they failed to reach the safe area within 2 min. The maze was cleaned and water was refreshed every day after the experiment. It was counted as an error when the mouse’s head collided against the blind end. Learning and memory abilities were assessed by the number of errors.
Measurement of DHA levels
At the end of the behavior experiment tests, the mice were decapitated; the brains were removed and weighed, and then frozen at 280°C for subsequent biochemical analysis. The lipid of hippocampus, frontal cortex and striatum tissues was extracted by using a method modified from Bligh and CitationDyer (1959). After a centrifuged procedure at 1,500 g for 10 min, the substrata were transferred to the test tube for vacuum drying. Dried crude lipids were weighed before proceeding to the phospholipid separation. The crude lipids were then dissolved in 200 μL of chloroform and applied onto a solid phase extraction column (Amino disposable extraction column, Bakerbond speTM, LOT × 02550, Eschborn, Germany) for phospholipid separation (CitationEdwards et al., 1998; CitationJumpsen et al., 1997).
Each sample of the hippocampus, frontal cortex, and striatum was analyzed for the individual fatty acid by gas chromatography (Lipid Standards, FAMEs, Sigma Co., St. Louis, MO). The detailed step-by-step procedures were applied according to the laboratory practice manual, as briefly described below (CitationEdwards et al., 1998). Extracted phospholipids were placed into 16 × 150 mm test tubes with Teflon-lined screw caps, and dissolved with 1 mL of 14% boron trifluoride methanol (BF3-methanol, Sigma) for fatty acid methylation reaction. Fatty acid methyl esters were analyzed by capillary gas chromatography (Trace GC, Thermo Finnigan, Inc., Rodano, Italy) equipped with a 30 m (0. 32 mm inter dimention (ID)) capillary column (cross-linked polyethylene glycol-TPA phase, Sulpelco), and flame ionized detector. DHA was identified according to the retention time of appropriate standard DHA-methyl esters. Researchers who participated in the laboratory were blind to the information of coded samples.
Measurement of the levels of monoamine neurotransmitters
DA, NE and 5-HT levels in hippocampus, frontal cortex and striatum were measured as described previously (CitationNitta et al., 1992) with minor modifications using high performance liquid chromatography (HPLC) with electrochemical detection. Briefly, each frozen tissue sample was homogenized by ultrasonication in 200 μL of 0.4 M perchloric acid (solution A). The homogenate was kept on ice for 1 h and then centrifuged at 12,000 g at 4°C for 20 min, and the pellet was discarded. An aliquot of 160 μL of supernatant was added to 80 μL of solution B (containing 0.2 M potassium citrate, 0.3 M di-potassium hydrogen phosphate, and 0.2 M EDTA). The mixture was kept on ice for 1 h and then centrifuged at 12,000 g at 4°C for 20 min again. Of the resultant supernatant, 20 μL was directly injected into an ESA liquid chromatography system equipped with a reversed-phase C18 column (150 × 4. 6 mm I.D., 5 μm) and an electrochemical detector (ESA CoulArray, Chelmsford, MA). The detector potential was set at 50, 100, 200, 300, 400, 500 mV, respectively. The mobile phase consisted of 125 mM citric acid-sodium citrate (pH 4.3), 0.1 mM EDTA, 1.2 mM sodium octanesulfonate and 16% methanol; the flow rate was 1 mL/min. The tissue levels of monoamine neurotransmitter were expressed in terms of nanograms per gram of tissue.
Enzyme-linked immunosorbent assay (ELISA) method analysis of BDNF and NGF in hippocampus, frontal cortex and striatum tissues
Hippocampus, frontal cortex and striatum were homogenized with an ultrasonic cell disrupter in 2 mL and 0.5 mL ice-cold lysis buffer (HEPES 25 mmol/L, MgCl2 · 6H2O 5 mmol/L, EDTA·2Na 5 mmol/L, pH 7.4, 0.5% (v/w) Triton X-100, DTT 5 mmol/L, PMSF 2 mmol/L, Pepstation A 10 μg/mL, Leupetion 10 μg/mL), respectively. The lysates were centrifuged at 10,000 g for 10 min at 4°C and the supernatant solutions were collected. The supernatant from each sample was diluted five times with phosphate buffer saline solution. After 15 min stay at room temperature, the diluted supernatants were neutralized to pH 7.6 and the gross protein were measured by Coomassie blue protein binding method using bovine serum albumin as standard. NGF, BDNF in the cerebral cortex and hippocampus were measured by a two-site enzyme-linked immunosorbent assay (ELISA) using NGF Emax®, BDNF Emax® Immunoassay system, respectively, according to the manufacturer’s protocols. The values obtained from the absorbance reader were expressed as pg/mg wet weight of tissue.
Statistical analysis
Data are expressed as means ± standard error of the mean (SEM). The data were analyzed by one-way ANOVA using SPSS program (version 10.0). Expression levels of NGF, BDNF protein were analyzed by either the unpaired t-test or one-way ANOVA. A probability value of less than 0.05 was considered as significant (aap < 0.05 versus young group; bbp < 0.01 versus aged group).
Results
The effect of DHA on the performance of mice in step-through test
The data in demonstrated that the aged group had significant cognitive deficits when compared with the young group. In the DHA-treated groups, the r etention time was longer, and the number of errors was significantly lower when compared with aged group. The data showed that the DHA-treated groups significantly increase retention time, and increase by 28.56% and 45.98%, respectively, when compared with the aged group. The mean number of errors was reduced from 1.7 in the aged group to 0.8 and 0.7 errors in DHA-treated groups respectively. Taking all the data into consideration, DHA can improve the learning and memory ability of aged mice.
Table 1. The effect of DHA on mouse memory performance in the step-through test. The data show DHA can significantly increase latency time and decrease number of errors by mice in the step-through avoidance test. There was significant difference between the DHA-treatment groups and aged group.
The effect of DHA on performances of mice in the passageway water maze
The data in and demonstrate that the mice in the aged group had significant cognitive deficits when compared with the young group. The escape latency period in the DHA-treated groups was shorter, and the number of errors was significantly lower when compared with the aged group. It could be concluded that DHA can improve the learning and memory ability of aged mice. Although exercise appears to have improved the performance of all mice, the experimental results still showed that DHA can improve learning and memory ability in the passageway water maze test.
Table 2. The effect of DHA on mouse latency period in the passageway water maze. The data shows DHA can decrease mouse escape latency period in the passageway water maze. From day 4 to day 6, the escape latency period of mice in each group continuously decreased. Moreover, there was significant difference in the escape latency period between the DHA-treatment groups and aged group.
Table 3. The effect of DHA on mouse number of errors in the passageway water maze. DHA can decrease the mouse number of errors in the passageway water maze. For days 4 to 6, there were significant differences between the high dose group and aged group. For days 4 to 5 there were significant differences between the low dose group and aged group, but no significant differences on day 6.
The changes of DHA levels in hippocampus, frontal cortex, and striatum
shows DHA levels were markedly decreased in hippocampus, frontal cortex, and striatum tissues of the aged group when compared with the young group. The results show that levels of DHA in three brain regions of the DHA-treated groups were significantly increased when compared with the aged group. The levels of DHA in the DHA-treated groups were increased by 1.23 and 1.42 times in hippocampus tissue, by 1.49 and 1.67 times in frontal cortex tissue, and by 1.34 and 1.46 times in striatum tissue, respectively, when compared with the aged group.
Table 4. The changes in DHA levels in hippocampus, frontal cortex and striatum tissue of mice. In hippocampus, the change of DHA levels is more obvious than in frontal cortex and striatum tissue.
The effects of DHA on monoamine neurotransmitters in hippocampus, frontal cortex, and striatum
shows the effects of DHA on DA, NE and 5-HT levels in three brain regions of aged mice, respectively. The data demonstrate that the DA, NE, and 5-HT levels in three brain regions of the aged group had significantly decreased when compared with the young group. The results showed the effects of DHA on DA, NE, and 5-HT levels were significantly increased in frontal cortex, hippocampus, and striatum. It revealed that monoamine neurotransmitters levels in frontal cortex, hippocampus, and striatum of aged mice were significantly increased by orally administered DHA.
The expression of NGF and BDNF protein in hippocampus, frontal cortex, and striatum
shows that the levels of NGF and BDNF proteins in hippocampus, frontal cortex and striatum tissues of DHA-treated groups were significantly increased when compared with the aged group. Mice treated with DHA showed a significant increase in BDNF protein levels of hippocampus, frontal cortex, and striatum tissues. also shows that NGF levels decreased by 72.80% in hippocampus of aged mice when compared with young mice, whereas no significant alterations were observed in striatum tissue. It indicated that NGF levels affected by aging were different and the mechanism dietary DHA affects NGF levels remains unclear in the three brain regions. The effect of DHA on NGF and BDNF levels among brain regions may be attributable to different sensitivity of each brain region to development and aging.
Table 5. The effect of DHA on DA, NE and 5-HT levels in three brain regions in mice.
Table 6. The effect of DHA on NGF and BDNF levels in hippocampus, frontal cortex, and striatum tissue of mice.
Discussion
Docosahexaenoic acid (DHA, 22:6n-3) is a major component of membrane phospholipids in nerve cells, thus is considered to be essential for proper neuronal development and function, and for brain development and neurological function (CitationAkbar et al., 2005; CitationSalem et al., 2001; CitationMinami et al., 1997).
In the present study we examined the effect of DHA supplementation on the performance of the step-through and passageway water maze test. Experimental results showed that learning and memory ability was reduced with aging and was restored by DHA supplementation in aged mice, which agreed with previous study results. Our experimental results still showed that DHA supplementation can increase DHA levels in hippocampus, frontal cortex, and striatum tissues. It means that dietary DHA supplementation can increase brain DHA content.
Monoamine neurotransmitters are known to play an important role in the cognitive functions (CitationArnsten et al., 1994; CitationGottfries, 1990). The experimental results showed that the levels of DA, NE and 5-HT in the brain tissues of naturally aged mice were remarkably lower than young mice. These data indicated that monoamine neurotransmitters changed a lot during the aging process, and treatment with DHA at doses of 200 and 400 mg/kg body weight/day by oral administration for 30 days can increase the level of DA, NE and 5-HT changed by aging.
Neurotrophic factors are known to be critically involved in neuritis outgrowth and cell survival in the central nervous system (CitationBarde, 1989). NGF and BDNF are present at the highest level in the hippocampal formation and cerebral cortex (CitationErnfors et al., 1990, CitationKorsching et al., 1985, CitationLevi-Montalcini, 1987). Moreover, they are the most abundant neurotrophins in the central nervous system and are important survival factors for cholinergic and dopaminergic neurons (CitationConner et al., 1998). The functionality of these neurons is crucial for memory processes (CitationFuji et al., 1993). As far as BDNF is concerned, BDNF is a trophic factor for the survival and differentiation of dopaminergic neurons and can influence dopamine and serotonin neurotransmission (CitationConner et al., 1998). Moreover, BDNF protein expression is critically involved in the consolidation phase of long-term memory due to its involvement in long-term potentiation in the hippocampus (CitationPang et al., 2004; CitationZafra et al., 1991). Furthermore, all monoamines are able to significantly increase BDNF astrocyte synthesis and secretion, and suggest the existence of a positive reciprocal interaction between monoaminergic neuronal activity and astrocyte neurotrophic support in neuron–astrocyte crosstalk which has a dynamic role in mediating neuronal plasticity and trophic functions in the brain (CitationMojca-Juric et al., 2006).
Our results also show that the levels of NGF and BDNF in the hippocampus, frontal cortex, and striatum of the aged group were significantly lower when compared with the young group, and orally administered DHA can significantly increase the levels of NGF and BDNF, but cannot significantly increase the level of NGF in the striatum. The NGF level in the striatum was essentially low as described previously (CitationHashimoto et al., 1994). The results from the present study suggested that the beneficial effects of DHA on cognitive function appear to be associated with NGF and BDNF. Therefore, we may speculate that DHA has neurotrophic effects and/or neurotrophin-modifying activity. More factors contributing to these changes, particularly the relationship between NGF and BDNF in brain tissue, may lead to a greater understanding of the effect of DHA on the amelioration of certain kinds of neurodegenerative and age-related losses in brain function.
In conclusion, the effect of DHA on two neurochemical systems (monoamine neurotransmitter and neurotrophic factor) have been examined in the hippocampus, frontal cortex, and striatum tissues. The present study has demonstrated that different DHA content in brain may result in different neurochemical and behavioral alterations. These findings also suggest that DHA supplementation during the old age period may represent a potential means for minimizing certain risk factors that may contribute to the neurochemical alterations associated with neuropsychiatric disorders. Further, investigations aimed at clarifying these issues would provide insights into the significance of DHA in neuronal replacement therapy.
Declaration of interest: This research was funded by the Sichuan Province Science and Technology Platforms Project, the Cellular and Molecular Biology Experiment Platform Construction Project for Chinese Traditional Medicine, Sichuan Finance and Education Project (No. 2005172).
References
- Acsa´dy L, Pascual M, Rocamora N, Soriano E, Freund TF (2000): Nerve growth factor but not neurotrophin-3 is synthesized by hippocampal GABAergic neurons that project to the medial septum. Neuroscience 98: 23–31.
- Akbar M, Calderon F, Wen Z, Kim HY (2005): Docosahexaenoic acid: A positive modulator of Akt signaling in neuronal survival. Proc Natl Acad Sic USA 102: 10858–10863.
- Arnsten AFT, Cai JX, Murphy BL,Goldman-Rakic PS (1994): Dopamine D1 receptor mechanisms in the cognitive performance of young adult and aged monkeys. Psychopharmacology 116: 143–151.
- Barde YA (1989): Trophic factors and neuronal survival. Neuron 2: 1525–1534.
- Barili P, De Carolis G, Zacchero D, Amenta F (1998): Sensitivity to aging of the limbic dopaminergic system: a review. Mech Ageing Devel 106: 57–92.
- Bligh EG., Dyer WJ (1959): A rapid method of total lipid extraction and purification. Can J Biochem Phys 37: 911–917.
- Catalan J, Moriguchi T, Slotnick B, Murthy M, Greiner RS, Salem N Jr (2002): Cognitive deficits in docosahexaenoic acid-deficient rats. Behav Neurosci 116: 1022–1031.
- Ciccarelli R, Ballerini P, Sabatino G, Rathbone MP, D’Onofrio M, Caciagli F, Di Iorio P (2001): Involvement of astrocytes in purine-mediated reparative processes in the brain. Int J Dev Neurosci 19: 395–414.
- Conner JM, Lauterborn JC, Gall CM (1998): Anterograde transport of neurotrophin proteins in the CNS 2 A reassessment of the neurotrophic hypothesis. Rev Neurosci 9: 91–103.
- Edwards R, Peet M, Shay J, Horrobin D (1998): Omega-3 polyunsaturated fatty acid levels in the diet and in red blood cell membranes of depressed patients. J Affect Disord 48: 149–155.
- Ernfors P, Ibanez CF, Ebendal T, Olson L, Persson H (1990): Molecular cloning and neurotrophic activities of a protein with structural similarities to nerve growth factor: Developmental and topographical expression in the brain. Proc Natl Acad Sci USA 87: 5454–5458.
- Fuji K, Hiramatsu M, Kameyama T, Nabeshima T (1993): Effects of repeated administration of propentofylline on memory impairment produced by basal forebrain lesion in rats. Eur J Pharmaco 236: 411–417.
- Gottfries CG (1990): Neurochemical aspects on aging and diseases with cognitive impairment. J Neurosci Res 27: 541–547.
- Hashimoto M, Hossain S, Shimada T, Sugioka K, Yamasaki H, Fujii Y, Ishibashi Y, Oka J, Shido O (2002): Docosahexaenoic acid provides protection from impairment of learning ability in Alzheimer’s disease model rats. J Neurochem 81: 1084–1091.
- Hashimoto Y, Furukawa S, Omae F, Miyama Y, Hayashi Y (1994): Correlative regulation of nerve growth factor level and choline acetyltransferase activity by thyroxine in particular regions of infant rat brain. J Neurochem 63: 326–332.
- Ikemoto A, Nitta A, Furukawa A, Ohishi M, Nakamura A, Fujii Y, Okuyama H (2000): Dietary n-3 fatty acid deficiency decreases nerve growth factor content in rat hippocampus. Neurosci Lett 285: 99–102.
- Jumpsen J, Lien EL, Goh YK, Glandinin T (1997): Small changes of dietary (n-6) and (n-3) fatty acid content ratio alter phosphatidylethanolamine and phosphatidylcholine fatty acid composition during development of neuronal and glial cells in rats. J Nutr 127: 724–731.
- Kodas E, Vancassel S, Lejeune B, Guilloteau D, Chalon S (2002): Reversibility of n−3 fatty acid deficiency-induced changes in dopaminergic neurotransmission in rats: Critical role of developmental stage. J Lipid Res 43: 1209–1219.
- Korsching S, Auburger G, Heumann R, Scott J, Thoenen H (1985): Levels of nerve growth factor and its mRNA in the central nervous system of the rat correlate with cholinergic innervation. EMBO J 4: 1389–1393.
- Lee JI, Chang CK, Liu IM, Chi TC, Yu HJ, Cheng JT (2001): Changes in endogeneous monoamines in aged rats. Clin Exper Pharmacol Physiol 28: 285–289.
- Levi-Montalcini R (1987): The nerve growth factor 35 years later. Science 237: 1154–1162.
- Lim SY, Suzuki H (2002): Dose-response effect of docosahexaenoic acid ethyl ester on maze behavior and brain fatty acid composition in adult mice. Int J Vitam Nutr Res 72: 77–84.
- Logan AC (2003): Neurobehavioral aspects of omega-3 fatty acids: Possible mechanisms and therapeutic value in major depression. Altern Med Rev 8: 410–425.
- Luine V, Bowling D, Hearns M (1990): Spatial memory deficits in aged rats: contributions of monoaminergic systems. Brain Res 537: 271–278.
- McGahon BM, Martin DS, Horrobin DF, Lynch MA (1999): Age-related changes in synaptic function: Analysis of the effect of dietary supplementation with omega-3 fatty acids. Neuroscience 94: 305–314.
- Miguez JM, Aldegunde M, Paz-Valinas L, Recio J, Sanchez-Barcelo E (1999): Selective changes in the contents of noradrenaline, dopamine and serotonin in rat brain areas during aging. J Neural Transm 106: 1089–1098.
- Minami M, Kimura S, Endo T, Hamaue N, Hirafuji M, Togashi H, Matsumoto M, Yoshioka M, Sato H, Watanabe S, Kobayashi T, Okuyama H (1997): Dietary docosahexaenoic acid increases cerebral acetylcholine levels and improves passive avoidance performance in stroke-prone spontaneously hypertensive rats. Pharmacol Biochem Behav 58: 1123–1129.
- Mojca-Juric DM, Milklic S, Carman-Krzan M (2006): Monoaminergic neuronal activity upregulates BDNF synthesis cultured neonatal rat astrocytes. Brain Res 1108: 54–62.
- Nitta A, Furukawa Y, Hayashi K, Hiramatsu M, Kamayama T, Hasegawa T, Nabeshima T (1992): Denervation of dopaminergic neurons with 6-hydroxydopamine increases nerve growth factor content in rat brain. Neurosci Lett 44: 152–156.
- Pang PT, Teng HK, Zaitsev E, Woo NT, Sakata K, Zhen S, Teng KK, Yung WH. Hempstead BL, Lu B (2004): Cleavage of proBDNF by tPA/plasmin is essential for long-term hippocampal plasticity. Science 306: 487–491.
- Salem N Jr, Litman B, Kim HY, Gawrisch K (2001): Mechanisms of action of docosahexaenoic acid in the nervous system. Lipids 36: 945–959.
- Suzuki H, Morikawa Y, Takahashi H (2001): Effect of DHA oil supplementation on intelligence and visual acuity in the elderly. World Rev Nutr Diet 88: 68–71.
- Tanila H, Taira T, Piepponen TP, Honkanen A (1994): Effect of sex and age on brain monoamines and spatial learning in rats. Neurobiol Aging 15: 733–741.
- Yamada K., Noda Y, Nakayama S, Komori Y, Sugihara H, Hasegawa T, Nabeshima T (1995): Role of nitric oxide in learning, and memory and in monoamine metabolism in the rat brain. Br J Pharmacol 115: 852–858.
- Zafra F, Castren E, Thoenen H, Lindholm D (1991): Interplay between glutamate and gamma-aminobutyric acid transmitter systems in the physiological regulation of brain-derived neurotrophic factor and nerve growth factor synthesis in hippocampal neurons. Proc Natl Acad Sci USA 88: 10037–10041.