Abstract
Antioxidant enzymes superoxide dismutase (SOD) and catalase were cross-linked with each other to form an antioxidant complex (polySOD-catalase). SOD activity was unaffected after polymerization reaction initiated by glutaraldehyde, and catalase activity was modestly decreased. In an oxidative stress model, where a xanthine/xanthine oxidase system was employed to generate superoxide radicals, catalase located at sufficient proximity to SOD after polymerization reaction provided effective protection for SOD from oxidative damage. In vivo evaluation indicated the antioxidant complex of polySOD-catalase had an extended circulation time suitable for therapeutic utility. As measured in anesthetized rate, polySOD-catalase can remain circulating with a half-life of as much as 6 h. Such a polymerization formulation could be a candidate therapeutic agent for age-related diseases associated with reactive oxygen species.
Introduction
Reactive oxygen species (ROS) are widely believed to contribute to the development of several age-related diseases, and perhaps, even to the aging process itself by causing “oxidative stress” and “oxidative injury” (CitationSohal et al., 2002). Other diseases in which oxidative injury have been implicated include cancer, diabetes, atherosclerosis, and other neurodegenerative diseases (CitationChowienczyk et al., 2000; CitationHalliwell, 2002; CitationParthasarathy et al., 2000). Therefore, actions that decrease ROS should be therapeutically beneficial, and successful antioxidant treatment should prevent or delay the onset of that disease, if the oxidative stress is involved in the origin of a disease. External antioxidant enzymes such as catalase or superoxide dismutase (SOD) in an organism could remove the increasing ROS along with the aging process. Superoxide radical (O2−) is dismuted by superoxide dismutase into oxygen and hydrogen peroxide. The latter is in turn transformed by catalase into water and oxygen () (CitationHalliwell, 2001).
Scheme 1. Relationship between superoxide dismutase (SOD), catalase (CAT), superoxide radical (O2−), and hydrogen peroxide (H2O2).
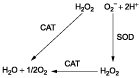
However, the experimental and therapeutic potentials of superoxide dismutase and catalase are limited by one vital factor: both proteins are rapidly cleared by the kidney, leading to circulatory half-lives of only 6-10 min following intravenous injection (CitationBullock et al., 1997; CitationD’agnillo & Chang, 1998). Thus, antioxidant enzymes cannot achieve effective pharmacological value.
In this study, glutaraldehyde was employed as bifunctional agent to cross-link superoxide dismutase and catalase intermolecularly. Their molecular weight was increased through covalent attachment to each other to hinder the renal clearance. Furthermore, the spatial proximity of superoxide dismutase and catalase after polymerization would favor efficient scavenging of ROS in sequence ().
Compared with other protein modification reagents, glutaraldehyde is much safer and more effective. A glutaraldehyde cross-linked bovine polyhemoglobin has been developed and is now well into Phase III clinical trials (CitationSprung et al., 2002). This bovine polyhemoglobin has been approved for routine clinical use in patients in South Africa (CitationLok, 2001). In North America, it has been approved for compassionate use in patients.
Materials and methods
Bovine erythrocyte superoxide dismutase (3300 U/mg), bovine milk xanthine oxidase (29.4 U/mL), xanthine (> 99%), lysine (monohydrochloride, SigmaUltra > 99%), cytochrome c (type III), glutaraldehyde (grade II, 25%), and pentobarbital sodium were purchased from Sigma-Aldrich. Bovine liver catalase (47413 U/mL) was obtained from ICN Inc. All other chemicals and reagents used were of analytical grade.
Experimental animals
Sprague-Dawley rats (250–275 g) were supplied by the Experimental Animal Center of Shandong University. The rats were fasted but allowed free access to water 12 h prior to experiment. The environment was maintained at 22°C with a 12 h light and dark cycle. The animal care and use were in accordance with guidelines of Shandong University.
Preparation of polySOD-catalase and polySOD
An aliquot of 2. 5 mg of SOD (3300 U/mg) was dissolved in 3.5 mL of 0.1 M potassium phosphate buffer, pH 7.4 and mixed with 1.5 mL of catalase (47413 U/mL). In polySOD mixtures, an equivalent volume of buffer replaced catalase addition. The polymerization reaction was started with slow addition of glutaraldehyde (0–0.5%, w/v) at 4°C with rotating (CitationD’agnillo & Chang, 1998). The reacting mixtures were kept rotating (150 rpm) for 6 h at 4°C. The polymerization reaction was terminated by the addition of 50 mg of lysine. Then solutions were centrifuged at 15,000 g for 1 h. Supernatants were collected and dialyzed at 4°C against 1 L Ringer’s lactate solution overnight.
Determination of superoxide dismutase and catalase activity
Superoxide dismutase activity was measured by the ferricytochrome c reduction method of CitationMcCord and Fridovich (1969). Catalase activity was determined by the method of CitationAebi (1974) by measuring the rate of decomposition of hydrogen peroxide at 240 nm.
Production of oxidative stress in vitro
The reaction of xanthine with xanthine oxidase was utilized as a source of continuous production of superoxide radicals. Reaction mixtures contained 50 mM potassium phosphate, pH 7.4, 100 μM xanthine, and 0.01 U/mL of xanthine oxidase (CitationKuppusamy & Zweier, 1989).
Animal manipulation
Male Sprague-Dawley rats were first anesthetized with an intraperitoneal injection of sodium pentobarbital (65 mg/kg). Polyethylene cannulae were inserted and secured distal to the superficial epigastric branches in the femoral arteries and veins. Both the venous and arterial catheters were filled with heparin saline (50 IU/mL) to avoid blood clotting. And then 4.5 mL of sample was injected into the vein catheter using a syringe with injection flow rate at about 0.5 mL/min. After injection of antioxidant samples, the blood samples were taken as desired and centrifuged at 1,000 g for 10 min. Supernatants were collected for SOD and catalase activity measurements. When monitoring procedures were completed, catheters were removed, the vessels were ligated, and local skin was sutured (CitationYu & Chang, 2004).
Results and discussion
Effect of glutaraldehyde on the catalytic activity of polySOD-catalase
It can be seen from , catalase activity was modestly decreased and SOD activity was almost unaffected after the glutaraldehyde polymerization reaction. There remains 74.8% of catalase activity after 6 h of cross-linking reaction with 0.1% glutaraldehyde, whereas more than 50% (52%) of catalase activity was lost with 0.5% glutaraldehyde, indicating that catalase activity decreased along with the increase in initial polymerization reagent concentration. However, SOD was still almost 100% of the original activity after 6 h of cross-linking reaction with as high as 0.5% of initial concentration of glutaraldehyde.
SOD stability of polySOD-catalase complex under in vitro oxidative stress
SOD could scavenge superoxide free radicals through the dismutation reaction it catalyzed (). However, hydrogen peroxide would accumulate along with increasing oxidative stress if SOD alone is employed as antioxidant enzyme. Excess hydrogen peroxide would damage SOD enzyme molecules and inhibit SOD activity. This explains why SOD is hypersensitive to oxidative damages. Actually, SOD is an efficient marker of the extent of oxidative stress (CitationTarpey et al., 2004).
The human and animal body produces reactive oxygen species by a variety of normal metabolic processes. The action of xanthine oxidase on xanthine results in the similar process of single-electron reduction of oxygen and produces both superoxide and hydroxyl free radicals (CitationKuppusamy & Zweier, 1989). So xanthine/xanthine oxidase system was employed as a source of continuous reactive oxygen species production in order to simulate oxidative stress circumstance in vivo.
As shown in , after cross-linking with catalase, SOD was well protected under oxidative stress because the resultant H2O2 from the dismutation reaction was quickly removed by the immediate proximity of the catalase molecules. SOD could still retain 88.3% of original enzyme activity even after exposure to xanthine/xanthine oxidase system for 30 min, whereas polymerized SOD without catalase could only be maintained at 32.8% of the initial activity in 30 min. And the activity of the free-form SOD had been almost totally inhibited under those circumstances. There was only 12.2% of the original enzyme activity left after a period of 30 min of continuous oxidative stress in vitro.
Plasma circulation time of antioxidant enzymes in vivo
demonstrates the increased circulation time of the polySOD-catalase in rats. Free-form SOD was rapidly cleared from the rat circulation with a half-life (t1/2) of 13 min, whereas in the polymerized form the circulatory half-life of SOD was improved to more than 6 h (364 min). Free-form catalase showed a greater retention of activity in plasma compared with free-form SOD. However, its circulatory half-life (51.8 min) still did not exceed one hour. After intermolecular polymerization with itself and SOD, the circulatory half-life of catalase was increased to 374 min.
Figure 3. Plasma circulation time of SOD and catalase in rats. Antioxidant enzyme samples were injected intravenously into anesthetized Sprague-Dawley rats. The activities of SOD (U) and catalase (U) remaining in the plasma were measured as a function of time. Rat plasma before injection was used as the blank for plasma enzyme activity determination. Initial glutaraldehyde concentration for polymerization reaction was 0.25%..
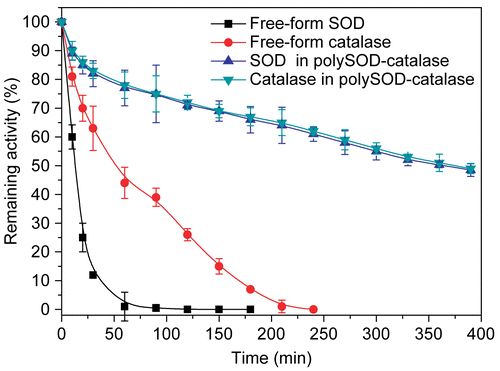
SOD is an interesting enzyme since while it dismutates superoxide radicals it also produces another highly reactive oxidizing agent, hydrogen peroxide, which in the presence of iron results in the production of the very toxic hydroxyl radicals via the Fenton reaction (CitationMcCord, 2002). Thus, although SOD is likely an important scavenger of superoxide radicals, its use as an exogenously administered antioxidant must be carefully examined.
From our results, after intermolecular cross-linking with catalase, the potential of SOD for therapeutic agent was greatly increased on both the stability under continuous oxidative stress in vitro and the restrictions associated with the circulation time in vivo. The catalase molecules cross-linked to SOD enzyme molecules ensure that hydrogen peroxide derived from SOD is removed by the immediate proximity of the catalase molecule as soon as the superoxide dismutation reaction occurs. In vitro it may not be necessary to have SOD and catalase cross-linked, since the process is unlikely to be diffusion-limited in a test tube. In vivo, however, it would be quite beneficial to have them linked before administration to preserve the efficacy of the ROS scavenging (CitationD’agnillo & Chang, 1998; CitationChang, 2006).
PolySOD-catalase as an efficient antioxidant complex would be fairly useful in clinical conditions in which damage is caused by superoxide and hydroxyl free radicals or in clinical situations in which hydrogen peroxide is produced, including where SOD is desirably employed to inactivate superoxide free radicals.
Declaration of interest: This work was supported by Science and Technology Research Fund no. B03-01 from the University of Jinan. The authors alone are responsible for the content and writing of the paper.
References
- Aebi H (1974): Catalase. In: Bergmeyer HU, eds., Methods of Enzymatic Analysis. New York, Academic Press, pp. 673–677.
- Bullock J, Chowdhury S, Severdia A (1997): Comparison of results of various methods used to determine the extent of modification of methoxy polyethylene glycol 5000 modified bovine cuprizinc superoxide dismutase. Anal Biochem 254: 254–262.
- Chang TMS (2006): Blood substitutes based on nanobiotechnology. Trends Biotechnol 24: 372–377.
- Chowienczyk PJ, Brett SE, Gopaul NK, Meeking D, Marchetti M, Russell-Jones DL, Anggard EE, Ritter JM (2000): Oral treatment with an antioxidant (raxofelast) reduces oxidative stress and improves endothelial function in men with type II diabetes. Diabetologia 43: 974–977.
- D’agnillo F, Chang TMS (1998): Polyhemoglobin-superoxide dismutasecatalase as a blood substitute with antioxidant properties. Nat Biotechnol 16: 667–671.
- Halliwell B (2001): Role of free radicals in the neurodegenerative diseases: Therapeutic implications for antioxidant treatment. Drugs Aging 18: 685–716.
- Halliwell B (2002): Effect of diet on cancer development: Is oxidative DNA damage a biomarker? Free Radic Biol Med 32: 968–974.
- Kuppusamy P, Zweier JL (1989): Characterization of free radical generation by xanthine oxidase. Evidence for hydroxyl radical generation. J Biol Chem 264: 9880–9884.
- Lok C (2001): Blood product from cattle wins approval for use in humans. Nature 410: 855–855.
- McCord JM (2002):Superoxide dismutase in aging and disease: An overview.Method Enzymol 349: 331–341.
- McCord, JM, Fridovich, I (1969): Superoxide dismutase: An enzymic function for. erythrocuprein (hemocuprein). J Biol Chem 244: 6049–6055.
- Parthasarathy S, Santanam N, Ramachandran S, Meilhac O (2000): Potential role of oxidized lipids and lipoproteins in antioxidant defense. Free Radic Res 33: 197–215.
- Sohal RS, Mockett RJ, Orr WC (2002): Mechanisms of aging: An appraisal of the oxidative stress hypothesis. Free Radic Biol Med 33: 575–586.
- Sprung J, James D, Kindscher JD, Wahr JA, Levy JH, Monk TG, Moritz MW, O’Hara PJ (2002): The use of bovine hemoglobin glutamer-250 (Hemopure) in surgical patients: Results of a multicenter, randomized, single-blinded trial. Anesth Analg 94: 799–808.
- Tarpey MM, Wink DA, Grisham MB (2004): Methods for detection of reactive metabolites of oxygen and nitrogen: In vitro and in vivo considerations. Am J Physiol Regul Integr Comp Physiol 286: 431–444.
- Yu BL, Chang TMS (2004): In vitro and in vivo enzyme studies of polyhemoglobintyrosinase. Biotechnol Bioeng 86: 835–841.