Abstract
Trypanosomiasis is one of the most important parasitic diseases worldwide. The undesirable side effects and low efficacy of classical trypanocidal drugs underline the necessity of the development of new drugs from natural products. Although marine algae have been recognized as attractive sources of known and novel bioactive compounds, very little research has been focused on antiprotozoal activity. Aqueous and organic extracts of 29 species of marine algae (14 species of Rhodophyta, seven species of Phaeophyta, and eight species of Chlorophyta) collected from the Gulf of Mexico and Caribbean coast of the Yucatan Peninsula (Mexico) were evaluated for their antiprotozoal activity in vitro against Trypanosoma cruzi trypomastigotes. The toxicity of these extracts was evaluated using brine shrimp (Artemia salina). The cytotoxicity on mammalian cells was also assessed by the MTT viability assay. The organic extracts from Dictyota caribea Horning & Schnetter, Lobophora variegata (J.V. Lamouroux) Womersley, Turbinaria turbinata Linnaeus, and Laurencia microcladia Kützing possess promising in vitro activity against T. cruzi trypomastigotes. The toxicity displayed by Laurencia microcladia against Artemia salina and the high cytotoxicity exhibited by T. turbinata must be taken into account in further studies.
Introduction
American trypanosomiasis, also known as Chagas’ disease, is one the most important parasitic infections worldwide caused by Trypanosoma cruzi, a protozoan parasite transmitted by hematophagous vectors from the Triatominae subfamily. The parasite plays a fundamental role in the development of organ lesions by sequentially inducing an inflammatory response, cellular lesions, and fibrosis. A report from the CitationWorld Health Organization (2002) estimates that a population of well over 16 million people may currently be infected, with some 120 million people at risk of contracting the infection, killing around 50,000 people annually due to chronic chagasic cardiomyopathy. Chagas’ disease is primarily a concern for people of Central and South American countries. However, due to recent population migrations from endemic countries toward developed countries, the threat of the disease is expanding to Europe and various parts of the USA; this is especially relevant in the context of transmission through blood transfusion (CitationRamsey et al., 2003).
The main drug available for the treatment of Chagas’ disease is benznidazole, whose action eliminates T. cruzi parasites. According to CitationTropical Disease Research (2005), this compound has several limitations such as a degree of high toxicity and low efficacy for parasitologic cure, mainly in the chronic phase of the disease, besides being poorly tolerated and causing severe side effects. The undersirable side effects associated with this classical trypanocidal drug, as well as the development of resistance, are encouraging research into alternative synthetic or natural compounds effective for the treatment of Chagas’ disease and for the chemoprophylactic treatment of banked blood. In this regard, most studies have been focused on trypanocidal activity of natural substances derived from higher plants, mainly due to their accessibility and use in traditional medicine (CitationChan-Bacab & Peña-Rodríguez, 2001; CitationPaveto et al., 2004).
The metabolic and physiological capabilities of marine organisms that allow them to survive in a complex habitat provide a tremendous potential for the production of unique metabolites, which are not found in terrestrial environments. Thus, marine organisms, and particularly sessile invertebrates, have been recognized as attractive sources of antiprotozoal compounds (CitationMayer & Hamann, 2005; CitationMorales-Landa et al., 2007). Marine algae are among the richest sources of known and novel bioactive compounds, and the biological activity of compounds derived from this group has been recently reviewed (CitationFaulkner, 2002; CitationSmith, 2004; CitationBlunt et al., 2006). Although very little research has been focused on their potential as a source of antiprotozoal compounds, recent studies have shown promising antimalarial activity in Laurencia sp. (CitationWright et al., 1996; CitationTopcu et al., 2003) as well as trypanocidal and leishmanicidal activity in Fucus evanescens C. Agardh, Pelvetia babingtonii (Harvey) De Toni (CitationNara et al., 2004), Ulva lactuca Linnaeus, and Sargassum natans (Linnaeus) Gaillon (CitationOrhan et al., 2006).
In the present study various tropical marine algae extracts (aqueous and organic) were evaluated for their potential parasitocidal effect at 24 h, 48 h, and 7 days on Trypanosoma cruzi trypomastigotes. A procedure for general toxicity screening that does not require much specialization is essential as a preliminary stage in the study of bioactive compounds. Thus, the toxicity of algal extracts was evaluated using a brine shrimp (Artemia salina) model, since this lethality assay has been used successfully to screen medicinal plants for their biological activity (CitationMeyer et al., 1982; CitationKrishnaraju et al., 2006). The cytotoxicity on mammalian cells was also assessed by the MTT viability assay, which detects mitochondrial activity in living cells (CitationMosmann, 1983). Although the brine shrimp lethality assay is considered a useful tool for preliminary assessment of toxicity, it has been demonstrated that the simultaneous use of both bioassays is appropriate to test natural marine products for biological and pharmacological activity (CitationCarballo et al., 2002).
Materials and methods
Collection and preparation of algal extracts
Marine algae were collected between January 2005 and May 2006 from different localities in the Gulf of Mexico (Telchac, 21°17′ N–88°58′ W; Dzilam de Bravo, 21°22′ N–88°57′ W; and Rio Lagartos, 21°24′ N–88°15′ W) and the Caribbean (Playa del Carmen, 20°46′ N–88°05′ W).
Once harvested, seaweeds were stored in plastic bags and chilled on ice for transport to the laboratory. Voucher specimens for all species were identified by L. León-Deniz according to CitationLittler & Littler (2000). Collected samples were washed thoroughly with fresh water to remove salts, sand, and epiphytes, and stored at −20°C. Entire plants of each macroalgae were lyophilized and milled into powder before extraction. Lyophilized samples (15 g) were extracted with 100 mL of dichloromethane:methanol (7:3) during 24 h. These extracts, hereafter named organic, were filtered and concentrated to dryness in vacuo at 40°C and stored at −20°C until required. To prepare aqueous extracts, 10 g of freeze-dried material was macerated for 12 h in constant agitation with 100 mL of distilled water. These extracts were kept as mentioned above.
Antitrypanosomal bioassay
Trypanosoma cruzi strain H1 was isolated from a human patient in the Yucatan Peninsula, Mexico. Parasites were cultured at 28°C in liver infusion tryptose (LIT) broth medium supplemented with 10% of fetal bovine serum (FBS), hemine (50 mg mL−1), penicillin (100 IU mL−1), and streptomycin (100 μg mL−1). The trypomastigotes were harvested at day 7 in the “log” phase (), counted in a Neubauer’s chamber, and adjusted to a concentration of 1 × 105 trypomastigotes/100 μL with fresh medium. For the bioassay, 100 μL of inoculum (1 × 105 trypomastigotes) was dispensed in 96-well plates containing 100 μL of serial dilutions of algal extracts (aqueous/organic) at concentrations of 5, 10, 50, 100, and 500 μg mL−1 and incubated at 28°C. The extracts were dissolved in 1% dimethylsulfoxide (DMSO) and diluted with broth medium. Live mobile trypomastigotes were counted with a Neubauer’s chamber. The growth inhibition percentage was determined after 24 h, 48 h, and 7 days of incubation, by comparison with controls. Violet crystal was used as a positive control, whereas untreated T. cruzi trypomastigotes were used as negative controls. All assays were performed in triplicate. IC50, defined as the concentration of extract which caused a 50% reduction in survival or viability in comparison to that in an identical culture without the extract, was determined by nonlinear regression analysis, by plotting the number of viable trypomastigotes versus log EC (effective concentration) values by use of GraphPad Prism 4 software. Comparisons of IC50 values were carried out by Student’s t-test.
Brine shrimp (Artemia salina) lethality assay
Brine-shrimp eggs (Microfeast®) were hatched at 27°C under continuous illumination in seawater prepared with 38 g L−1 sea salt (Sigma®) supplemented with 6 mg L−1 dried yeast, and with air bubbling. The algal extracts were dissolved in DMSO (final concentration 1%) and diluted with seawater to concentrations of 10, 100, and 1000 μg mL−1. After 48 h, 10 nauplii were placed in each test tube containing test concentrations and controls. The lethal concentration of 50% (LC50) was determined by counting the dead nauplii after a 24 h incubation period. Data were analyzed with the Finney computer program, as described previously (CitationMeyer et al., 1982; CitationSolis et al., 1993). All assays were done in triplicate.
Cytotoxicity test on mammalian cells
Dog kidney cells (MDCK) were grown in DMEM (Dulbecco’s modified Eagle’s medium; Gibco) supplemented with 10% (v/v) FBS (Gibco) with 100 U mL−1 penicillin and 100 mg mL−1 streptomycin, and maintained at 37ºC in a 5% CO2 atmosphere with 95% humidity.
The cytotoxicity assay was performed according to CitationRahman et al. (2001), where 1.5 × 104 viable cells from the cell line were seeded in a 96-well plate (Costar) and incubated for 24–48 h. When cells reached >80% confluence, the medium was replaced and the cells were treated with the crude extracts at 6.25, 12.5, 25, and 50 μg mL−1 dissolved in DMSO at a maximum concentration of 0.05%. After 72 h of incubation, 10 μL of a 0.005% 3-(4,5-dimethylthiazol-2-yl)-2,5-diphenyl tetrazolium bromide (MTT; Sigma) solution (5 mg mL−1) was added to each well and incubated at 37ºC for 4 h. The medium was removed and the formazan, a product generated by the activity of dehydrogenases in cells, was dissolved in acidified isopropanol (0.4 N HCl). The amount of MTT-formazan is directly proportional to the number of living cells, and was determined by measuring the optical density (OD) at 540 nm using a bio-assay reader (BioRad, USA). Violet crystal was used as a positive control, whereas untreated cells were used as negative controls. The concentration of crude extract that killed 50% of the cells (CC50) was calculated by GraphPad Prism 4 software. All determinations were performed in triplicate.
Results
Taxonomic information and the origin of the 29 species of macroalgae tested, as well as the yield of organic and aqueous extracts (% of dry weight), are shown in .
Table 1. Yield of organic and aqueous extracts of the studied algae.
The in vitro activity of the algal extracts tested against Trypanosoma cruzi trypomastigotes is summarized in . The criteria for crude extract antitrypanosomal activity, as established by CitationLeite et al. (2006), were considered as follows: active (100% reduction in the number of parasites), partially active (50–90% reduction), and inactive (<50%).
Table 2. In vitro activity of organic and aqueous extracts from the algal species tested against Trypanosoma cruzi trypomastigotes at three different times.
In general, aqueous extracts were inactive against T. cruzi trypomastigotes. Thus, the results for the activity of the organic extracts revealed that Dictyota caribaea, Lobophora variegata (Phaeophyta), Penicillus dumetosus, Rhipocephalus phoenix f. brevifolius, Udotea conglutinata, and Udotea flabellum (Chlorophyta) caused total inhibition of parasites at 24 h, 48 h, and even after 7 days of growth (). In addition, the organic extracts of Gracilaria caudate, Gracilaria cervicornis, Laurencia microcladia (Rhodophyta), and Turbinaria turbinata (Phaeophyta) inhibited the totality of the parasites at 48 h of growth and this effect continued up to 7 days. It is interesting to note that although a total inhibition of parasites was observed at 24 h for P. dumetosus, U. conglutinata, and U. flabellum, these extracts had an oily consistency at the highest concentrations (500 and 250 μg mL−1). This fact may be related to micelles formation and the death of the parasites by suffocation, and therefore the above species were not taken into account in this study as a potential antitrypanosoma source.
Based on the above mentioned results, the in vitro antitrypanosomal activity of species that caused 100% reduction in the number of Trypanosoma cruzi trypomastigotes was expressed in the form of dose–response curves. The data derived from antitrypanosomal activity were transformed into IC50 values for statistical comparison and were classified according to CitationCalderon et al. (2006) as follows: high activity (IC50 <10 μg mL−1), average (IC50 between 10 and 20 μg mL−1), and low activity (IC50 >20 μg mL−1). The IC50 of the standard violet crystal at 24 h was 0.02 μg mL−1
The inhibitory effect of the Rhodophyta Gracilaria caudata, G. cervicornis, and Laurencia microcladia on T. cruzi trypomastigotes and their IC50 values at 24 h, 48 h, and 7 days are summarized in . All extracts displayed a dose-dependent inhibitory effect, showing 100% reduction at the highest concentrations. The strongest and fastest inhibition capacity was observed for L. microcladia extract, which showed highest activity at 48 h (IC50 9.47 ± 1.41 μg mL−1) with a slight increase in activity after 7 days (IC50 8.67 ± 1.63 μg mL−1).
Figure 2. Antiprotozoal activity of Rhodophyta species that caused 100% reduction in Trypanosoma cruzi trypomastigotes at 24 h (dotted curve), 48 h (dashed curve), and 7 days (solid curve). (a) Gracilaria caudata (48 h, IC50 = 403.4 μg mL−1; 7 days, IC50= 7.72 μg mL−1). (b) Gracilaria cervicornis (48 h, IC50 = 133.9 μg mL−1; 7 days, IC50 = 166 μg mL−1). (c) Laurencia microcladia (48 h, IC50 = 9.47 μg mL−1; 7 days, IC50 = 8.67 μg mL−1). All IC50 values are significantly different at p ≤ 0.05.
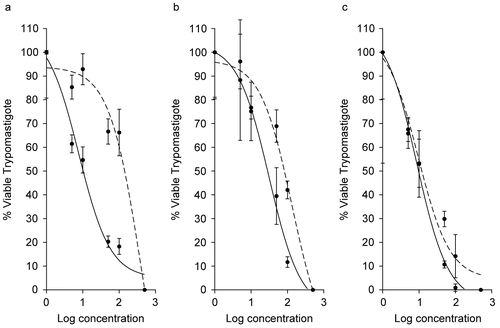
Other extracts from members of the Phaeophyta extracts also showed 100% reduction at the highest concentrations (). The strongest and fastest inhibition capacity was shown by Turbinaria turbinata (IC50 7.55 ± 1.52 μg mL−1), Dictyota caribaea (IC50 7.73 ± 1.41 μg mL−1), and L. variegata (IC50 9.72 μg mL−1), with high activity at 48 h. All the above species maintained this high activity after 7 days with the exception of T. turbinata, which had reduced activity (IC50 14.24 μg mL−1).
Figure 3. Antiprotozoal activity of Phaeophyta species that caused 100% reduction in Trypanosoma cruzi trypomastigotes at 24 h (dotted curve), 48 h (dashed curve), and 7 days (solid curve). (a) Dictyota caribaea (24 h, IC50 = 48.05 μg mL−1; 48 h, IC50 = 7.73 μg mL−1; 7 days, IC50 = 9.28 μg mL−1). (b) Lobophora variegata (24 h, IC50 = 84.37 μg mL−1; 48 h, IC50 = 9.72 μg mL−1; 7 days, IC50 = 6.48 μg mL−1). (c) Turbinaria turbinata (48 h, IC50 = 7.55 μg mL−1; 7 days, IC50 = 14.24 μg mL−1). All IC50 values are significantly different at p ≤ 0.05.
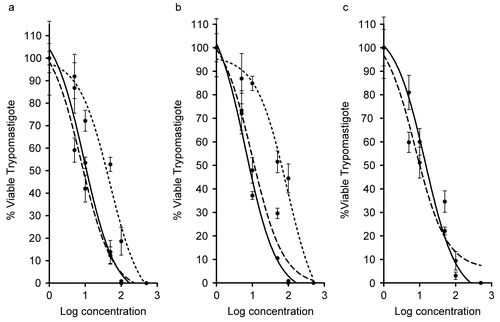
For Chlorophyta Halimeda incrassata and Rhipocephalus phoenix a 100% reduction was also observed at the highest concentrations (). A high and an average activity was found for H. incrassata (IC5010.26 ± 1.53 μg mL−1) and R. phoenix (IC50 16.32 ± 2.17 μg mL−1), respectively, at 7 days. It is interesting to note that extracts of R. phoenix as well as the red alga Gracilaria caudata dramatically increased their activities after 7 days ( and ).
Figure 4. Antiprotozoal activity of Chlorophyta species that caused 100% reduction in Trypanosoma cruzi trypomastigotes at 24 h (dotted curve), 48 h (dashed curve), and 7 days (solid curve). (a) Halimeda incrassata (7 days, IC50 = 10.26 μg mL−1). (b) Rhipocephalus phoenix f. brevifolius (24 h, IC50 = 229.6 μg mL−1; 48 h, IC50 = 112.1 μg mL−1; 7 days, IC50 = 16.32 μg mL−1). All IC50 values are significantly different at (p ≤ 0.05).
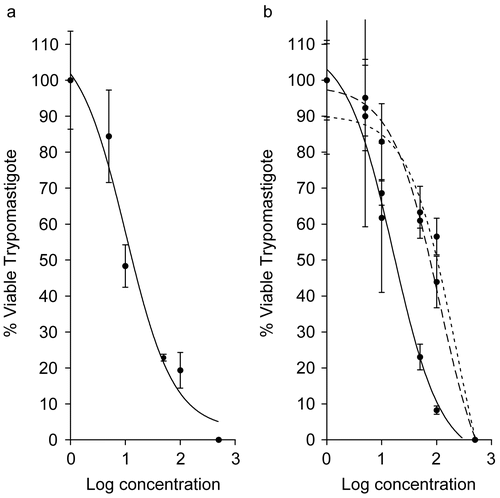
summarizes the toxicity of the algal extracts with antitrypanosomal activity (IC50 ≤10 μg mL−1). None of the algal extracts tested showed toxicity against Artemia salina (LC50 values >1000 μg mL−1) with the exception of L. microcladia (LC50 119.8 ± 2.09 μg mL−1). On the other hand, only the extract of T. turbinata exhibited a high cytotoxicity against MDCK cells (CC50 23.33 μg mL−1), and this effect was lower than for the drug standard violet crystal (CC50 0.11 ± 0.02 μg mL−1). Additionally, also shows the selectivity index (SI) of extracts, which is defined as the ratio of cytotoxicity to biological activity. Even if this in vitro selectivity index does not allow extrapolation to the in vivo situation, the index is valuable for determining compounds with selective activity against protozoa, although not host cells, at the early phase of a screening process for further evaluation in animal models.
Table 3. Algae organic extract toxicity against Artemia salina (LC50 μg mL−1 ± SD) and cytotoxic activity (CC50 μg mL−1 ± SD) on mammalian cells.
Discussion
Trypanosoma cruzi is a protozoan parasite whose life cycle involves invertebrate and vertebrate hosts. In mammals the parasite exists in two forms: the extracellular trypomastigote that is a non-dividing invasive hemolymphatic form, and the amastigote that divides in the cytoplasm of the cells. The trypomastigote form is easily cultured and sensitive to drug action, and therefore it is an excellent model for preliminary in vitro screening. The present results revealed high antitrypanosomal activity (IC50 ≤10 μg mL−1) at 48 h from brown algae belonging to the orders Dictyotales (Lobophora variegata, Dictyota caribaea) and Fucales (Turbinaria turbinata) and from red algae belonging to the order Ceramiales (Laurencia microcladia). It is noteworthy that Phaeophyta was the algal division with more species having antitrypanosomal activity. In support of our results, several reports on marine algae have demonstrated inhibitory effects against T. cruzi only for brown algae species (CitationTakeaki et al., 2003; CitationNara et al., 2004; CitationOrhan et al., 2006).
Although the above species showed a promising trypanocidal effect, the toxicity displayed by Laurencia microcladia against Artemia salina and the high cytotoxicity exhibited by Turbinaria turbinata () must be taken into account for further studies. It is generally considered that biological efficacy is not due to in vitro cytotoxicity when SI ≥ 10 (CitationVonthron-Sénécheau et al., 2003). In this study, three out of four seaweeds with high antitrypanosomal activity showed SI ≥ 10, indicating a higher selectivity of the extract to the parasite than to the mammalian cells. Only the extract from Turbinaria turbinata showed low selectivity (SI 3.09). A similar observation was evident for the standard drug. The crude extract complex could contribute to the cytotoxic effect of T. turbinata. In this way, several cytotoxic and antitumoral compounds have been isolated from the genus Turbinaria such as turbinaric acid (CitationAsari et al., 1989), sterols (CitationSheu et al., 1997), and oxygenated fucosterols (CitationSheu et al., 1999) that could be responsible for the cytocidal effect.
In this study it is not possible to infer whether one molecule, several molecules, or the interaction between molecules contributes to the antitrypanosomal activity of the analyzed extracts. The presence of terpenes and other compounds such as long-chain fatty acids, organosulfur compounds, acetogenins, and polyphenols may be responsible for the activity displayed, since these types of metabolites isolated from terrestrial sources have been demonstrated to have antitrypanosomal activity (CitationSepulveda-Boza & Cassels, 1996). In this stage of our investigation we did not include structure elucidation, but literature search revealed that macroalgal species showing antitrypanosomal activity in this study have also been reported to contain terpenes, acetogenins, and polyphenols (CitationFaulkner, 2002).
It is difficult to speculate the mechanism by which these compounds act as trypanocidal agents. In this regard, CitationSepulveda-Boza and Cassels (1996) suggest that many natural products exhibit their trypanocidal activity by virtue of their interference with the redox balance of the parasites, acting either on the respiratory chain or on the cellular defenses against oxidative stress. It is also known that some compounds act by binding with the DNA of the parasite. For example, dihydroorotate dehydrogenase (DHOD), the fourth enzyme in the de novo pyrimidine biosynthetic pathway, is essential to Trypanosoma cruzi, including the electron acceptor capacity and cellular localization (CitationNara et al., 2000). In this way, it has been recently demonstrated that the methanol extracts of brown algae Ishige okamurae Yendo, Fucus evanescens, and Pelvetia babingtonii contain potent noncompetitive inhibitors against T. cruzi DHOD (CitationTakeaki et al., 2003; CitationNara et al., 2004).
The seaweed extracts of Dictyota caribaea and Lobophora variegata that showed both high antitrypanosomal activity and high selectivity at this stage have been selected for bio-guided fractionation. Phytochemical studies of these algae are being performed in order to identify the most effective fraction or compound, bearing in mind that the optimal product may not always correspond to a single compound.
Acknowledgements
We are grateful to C. Chávez Quintal and M. J. Chan-Bacab for their technical assistance during laboratory analysis.
Declaration of interest: This study was supported by the project SEP-CONACYT 53687.
References
- Asari F, Kusumi T, Kakisawa H (1989): Turbinaric acid, a cytotoxic secosqualene carboxylic acid from the brown alga Turbinaria ornata. J Nat Prod 52: 1167–1169.
- Blunt JW, Copp BR, Munro MHG, Northcote PT, Prinsep MR (2006): Marine natural products. Nat Prod Rep 23: 26–78.
- Calderon AI, Romero LI, Ortega-Barría E, Brun R, Correa MDA, Gupta MP (2006): Evaluation of larvicidal and in vitro antiparasitic activitites of plants in a biodiversity plot in the Altos de Campana National Park Panama. Pharm Biol 44: 487–498.
- Carballo JL, Hernández-Inda ZL, Pérez P, García-Grávalos MD (2002): A comparison between two brine shrimp assays to detect in vitro cytotoxicity in marine natural products. BMC Biotechnol 2: 17–22.
- Chan-Bacab MJ, Peña-Rodríguez LM (2001): Plant natural products with leishmanicidal activity. Nat Prod Rep 18: 674–688.
- Faulkner DJ (2002): Marine natural products. Nat Prod Rep 19: 1–48.
- Krishnaraju AV, Rao TVN, Sundararaju D, Vanisree M, Tsay H, Subbaraju GV (2006): Biological screening of medicinal plants collected from Eastern Ghats of India using Artemia salina (brine shrimp test). Int J Appl Sci Eng 4: 115–125.
- Leite JPV, Oliveira AB, Lombardi JA, Filho JDS, Chiari E (2006): Trypanocidal activity of triterpenes from Arrabidaea triplinervia and derivatives. Biol Pharm Bull 29: 2307–2309.
- Littler DS, Littler MM (2000): Caribbean Reef Plants, 2nd ed. Washington, DC, Offshore Graphics, Inc., p. 300.
- Mayer AMS, Hamann MT (2005): Marine pharmacology in 2001-2: marine compounds with antibacterial, anticoagulant, antifungal, anti-inflammatory, antimalarial, antiplatelet, antiprotozoal, antituberculosis and antiviral activities affecting the cardiovascular, immune and nervous systems and other miscellaneous mechanisms of action. Comp Biochem Phys C 140(3–4): 265–286.
- Meyer BN, Ferrigni NR, Putnam JE, Jacobsen LB, Nichols DE, McLaughlin JL (1982): Brine shrimp: A convenient general bioassay for active plant constituents. Planta Med 45: 31–34.
- Morales-Landa JL, Zapata-Pérez O, Cedillo-Rivera R, Segura-Puertas L, Simá-Alvarez R, Sánchez-Rodríguez J (2007): Antimicrobial, antiprotozoal and toxic activities of Cnidarian extracts from the Mexican Caribbean Sea. Pharm Biol 45: 37–43.
- Mosmann T (1983): Rapid colorimetric assay for cellular growth and survival: application to proliferation and cytotoxicity assays. J Immunol Methods 16: 55–63.
- Nara T, Hashimoto T, Aoki T (2000): Evolutionary implications of the mosaic pyrimidine-biosynthetic pathway in eukaryotes. Genes 257: 209–222.
- Nara T, Kamei Y, Tsubouchi A, Annoura T, Hirota K, Lizumi K, Dohmoto Y, Ono T, Aoki T (2004): Inhibitory action of marine algae extracts on the Trypanosoma cruzi dihydroorotate dehydrogenase activity and on the protozoan growth in mammalian cells. Parasitol Int 54: 59–64.
- Orhan I, Sener B, Atici T, Brun R, Perozzo R, Tasdemir D (2006): Turkish freshwater and marine macrophyte extracts show in vitro antiprotozoal activity and inhibit FabI, a key enzyme of Plasmodium falciparum fatty acid biosynthesis. Phytomedicine 13: 388–393.
- Paveto C, Güida MC, Esteva MI, Martino V, Coussio J, Flawiá MM, Torres HN (2004): Anti-Trypanosoma cruzi activity of green tea (Camellia sinensis) catechins. Antimicrob Agents Chemother 48: 69–74.
- Rahman A, Choudhary MI, Thomsen WJ (2001): Bioassay techniques for drug development. In: Atta-ur-Rahman MIC, Thomsen W, eds., Manual of Bioassay Techniques for Natural Products Research. Amsterdam, Harwood Academic Publishers, pp. 34–35.
- Ramsey JM, Tello LA, Pohls JS (2003): Iniciativa para la vigilancia y el control de la enfermedad de chagas en la Republica Mexicana. Cuernavaca, Mexico, Instituto Nacional de Salud Publica, pp. 24.
- Sepulveda-Boza S, Cassels BK (1996): Plant metabolites active against Trypanosoma cruzi. Planta Med 62: 98–105.
- Sheu JH, Wang GH, Sung PJ, Chiu YH, Duh CY (1997): Cytotoxic sterols from the Formosan brown alga Turbinaria ornata. Planta Med 63: 571–572.
- Sheu JH, Wang GH, Sung PJ, Duh CY (1999): New cytotoxic oxygenated fucosterols from the brown alga Turbinaria conoides. J Nat Prod 62: 224–227.
- Smith AJ (2004): Medicinal and pharmaceutical uses of seaweed natural products: a review. J Appl Phycol 16: 245–262.
- Solis PN, Wright CW, Anderson MM, Gupta MP, Phillipson JD (1993): A microwell cytotoxicity assay using Artemia salina (brine shrimp). Planta Med 59: 250–252.
- Takeaki O, Ryuta T, Takeshi N,Takashi A,Yuto K (2003): Screening for anti-Trypanosoma cruzi dihydroorotate dehygrogenase from marine algae collected from Japanese coasts. Coast Bioenviron 2: 67–76.
- Topcu G, Anydoqmus Z, Imre S, Goren AC, Pezzuto JM, Clement JA, Kingston DG (2003): Brominated sesquiterpenes from the red alga Laurencia obtusa. J Nat Prod 66: 1505–1508.
- Tropical Disease Research (2005): Progress 2003-2004. 17th Programme Report of the UNICEF/UNDP/World Bank/WHO Special Programme for Research and Training in Tropical Diseases. Geneva, TDR, pp. 31–34.
- Vonthron-Sénécheau C, Bernard Weniger B, Ouattara M, Tra Bi F, Kamenan A, Lobstein A, Brun R, Anton R (2003): In vitro antiplasmodial activity and cytotoxicity of ethnobotanically selected Ivorian plants. J Ethnopharmacol 87: 221–225.
- Wright AD, Koning GM, Angerhofer CK, Greenidge P, Linden A, Desqueyroux-Faundez R (1996): Antimarial activity: the search for marine-derived natural products with selective antimalarial activity. J Nat Prod 59: 710–716.
- World Health Organization (2002): Control of Chagas Disease. WHO Technical Report Series 905. Geneva, World Health Organization.