Abstract
The high risk of human exposure to ionizing radiations during radiation therapy and also during space travel underscores the need to develop novel radioprotectors with improved efficacy. Increased oxidative stress and antioxidant deficit have been suggested to play a major role in radiation induced toxicity, and hence maintaining an antioxidant balance through dietary supplementation might provide beneficial effects during radiation exposure. The present study was designed to evaluate the effect of hesperidin, a flavanone glycoside found in citrus plants, on the antioxidant defense system and lipid peroxidation against γ-radiation induced damage in rats. Exposure of rats to γ-radiation (5 Gy) resulted in tissue damage characterized by significantly elevated levels of serum marker enzymes (AST, ALT, ALP, LDH, and CPK) and a decrease in their activities in the heart tissue. γ-Radiation induced oxidative stress was observed by elevated levels of lipid peroxidation and a decrease in the activities of endogenous antioxidants (SOD, CAT, GPx, and GSH) in the heart and kidney of rats. Post-treatment with hesperidin (50 and 100 mg/kg bw/day, orally) for 7 days following exposure to γ-radiation significantly attenuated these changes when compared to the radiation exposed groups. Histopathological examination of the heart tissue of rats exposed to γ-radiation and treated with hesperidin also showed minimal damage when compared to those exposed to γ-radiation alone. These findings indicate the protective effect of hesperidin on lipid peroxidation and the antioxidant tissue defense system during γ-radiation induced tissue damage in rats.
Introduction
Ionizing radiation has become an integral part of modern medicine and is widely employed in the treatment of several disorders such as cataracts and tumors. Although radiation is used in treating a variety of disorders, it has been reported to produce harmful effects in patients undergoing radiotherapy, as normal cells are also unnecessarily exposed to radiation. Further, human exploration into deep space also results in exposure to radiation as men travel beyond the earth’s protective magnetic field. The degree of these harmful effects depends on dose, frequency, duration of radiation exposure, and amount of exposed area (CitationHannig et al., 2000). The major problem when using radiation for therapy is that it is known to generate reactive oxygen species (ROS) in the irradiated tissue (CitationMansour, 2006). As the human body comprises more than 80% water, the major radiation damage is due to the aqueous free radicals generated by the radiolysis of water. These free radicals react with cellular macromolecules in their vicinity, such as DNA, RNA, proteins, membrane, etc., and cause cell dysfunction and mortality (CitationTominaga et al., 2004). The main cause for concern is that, during radiotherapy, not only the tumor cells but the surrounding normal healthy cells are also exposed to radiation, resulting in oxidative stress and subsequent tissue damage (CitationNair et al., 2001). Under normal conditions, the body’s endogenous enzymic and non-enzymic antioxidant systems can control the flux of generated reactive oxygen species (ROS). The overproduction of ROS in both intra- and extracellular spaces upon exposure of cells or tissues to radiation results in an imbalance between pro-oxidant and antioxidant statuses, resulting in oxidative stress (CitationYilmaz & Yilmaz, 2006).
Hesperidin (hesperetin-7-rhamnoglucoside) is a flavanone glycoside abundantly found in sweet oranges and lemons, and is an inexpensive by-product of citrus cultivation. Traditionally, hesperidin is commonly used as a combination product, namely Daflon (Servier, Switzerland), which contains hesperidin and diosmin (CitationGarg et al., 2001). Hesperidin has been reported to possess antihypercholesterolemic activity (CitationSon et al., 1991), anti-inflammatory and analgesic activity (CitationGalati et al., 1994), antifungal activity (CitationKrolicki & Lamer-Zarawaska, 1984), and anticarcinogenic activity (CitationYang et al., 1997). Its deficiency has been linked to abnormal capillary leakiness as well as pain in the extremities, causing aches, weakness, and night leg-cramps. Supplementation of hesperidin also helps in reducing edema or excess swelling in the legs due to fluid accumulation (CitationGarg et al., 2001). Hesperidin was found to reduce superoxide ions in an electron transfer plus concerted proton transfer reaction in vitro (CitationJovanovic et al., 1994). The antioxidant activity and radical scavenging properties of hesperidin have been reported by several investigators, using a variety of assay systems (CitationDeng et al., 1997; CitationMiller & Rice-Evans, 1997; CitationSuarez et al., 1998; CitationMalterud & Rydland, 2000). Further, hesperidin was found to be effective in protecting liposomes from ultraviolet (UV)-irradiation induced peroxidation, probably by scavenging the oxygen free radicals generated by UV-irradiation (CitationBonina et al., 1996).
The present study was designed to investigate the protective effect of hesperidin on γ-radiation induced oxidative stress and resultant dysfunction of the rat heart and kidney in male Sprague-Dawley rats.
Materials and methods
Chemicals
All the chemicals, including hesperidin, thiobarbituric acid, nicotinamide adenine dinucleotide (NAD), lithium lactate, 5,5′-dithiobis-(2-nitrobenzoic acid), and glutathione, were purchased from Sigma Chemical Co., St. Louis, USA.
Animals
Inbred strains of Sprague-Dawley rats purchased from Central Lab Animal Inc., Seoul, Korea were used for the experiments. Rats weighing 150 ± 5 g were acclimatized to animal house conditions and maintained under controlled conditions of temperature (23 ± 2°C), humidity (55 ± 5%), and light (12 h light/dark cycle) at the Central Animal Research Laboratory, Advanced Radition Technology Institute (ARTI), Korea Atomic Energy Research Institute (KAERI). The animals were provided with a sterile pellet diet and water ad libitum. Three animals were housed together in polypropylene cages containing sterile husk bedding, throughout the experiment. The study was approved by the Institutional Animal Ethical Committee and performed in strict compliance with the guidelines prescribed by the committee.
Irradiation of animals
Irradiation of animals was carried out using the Gammacell 40 Exactor (MDS Nordion, Canada) at ARTI, KAERI. Unanesthetized animals were placed in a well ventilated acrylic restrainer and exposed to whole body γ-radiation at a dose of 5 Gy. Immediately after irradiation the animals were sorted into individual polypropylene cages and monitored daily for the development of symptoms of radiation sickness and mortality.
Drug preparation and dosing
Hesperidin (Sigma Chemical Co.) was dissolved in saline and was administered orally to rats using a ball tipped needle exactly 1 h after their exposure to γ-radiation. The drug was administered at the same time on all 7 days of the experiment and was freshly prepared on each day. Two doses (50 and 100 mg/kg) of hesperidin were selected for this study based on the reports of CitationHosseinimehr and Nemati (2006), where they have shown the protective effect of various doses of hesperidin against γ-radiation induced damage to mouse bone marrow cells.
Experimental design
Animals were divided into five groups with six animals in each group. The experimental design and treatment protocol was as follows:
Group 1: Rats served as controls and received saline for 7 days.
Group 2: Rats were exposed to 5 Gy of γ-radiation and left for 7 days.
Group 3: Rats were exposed to 5 Gy of γ-radiation and treated with hesperidin (50 mg/kg bw, orally) for 7 days.
Group 4: Rats were exposed to 5 Gy of γ-radiation and treated with hesperidin (100 mg/kg bw, orally) for 7 days.
Group 5: Rats were treated with hesperidin (100 mg/kg bw, orally) for 7 days.
Twenty-four hours after the last dose of hesperidin, animals (after overnight fasting) were anesthetized with ether, and blood was collected from the retro-orbital plexus using a sterile capillary tube. The blood was allowed to clot, then it was centrifuged (1000g for 20 min), and serum was separated and stored at 4°C. The animals were then killed by decapitation and the heart and kidney were excised, washed in ice cold saline, blotted dry, and weighed. The tissue was homogenized in phosphate buffer (0.1 M, pH 7.4) using a Wisestir™ HS 30E homogenizer fitted with Teflon pestle, to yield a 10% homogenate. The crude homogenate was centrifuged (1000g for 10 min at 4°C) and the clear supernatant was used for biochemical analysis. A portion of the heart tissue was fixed in 10% buffered formalin, sectioned, stained with hematoxylin and eosin, and viewed under light microscopy for histological changes.
Biochemical analysis
Aspartate and alanine transaminases (AST and ALT) were assayed in the serum and heart tissue homogenate according to the method of CitationBergmeyer et al. (1978). Alkaline phosphatase (ALP) was assayed in the serum and tissue homogenate according to CitationKing (1965a), using disodium phenyl phosphate as substrate. Lactate dehydrogenase (LDH) was assayed by the method of CitationKing (1965b), using lithium lactate as substrate. Protein was estimated by the method described by CitationBradford (1976), using bovine serum albumin as standard. Creatine phosphokinase (CPK) in the serum and heart tissue was estimated by the method of CitationOkinaka et al. (1961). Lipid peroxidation (LPO) was determined in the tissues as described by CitationOhkawa et al. (1979). Superoxide dismutase (SOD) and catalase (CAT) in the tissues were assayed using commercial assay kits (Calbiochem, Germany) as per the manufacturer’s instructions. The activity of glutathione peroxidase (GPx) in the tissues were estimated by the method of CitationRotruck et al. (1973). Total glutathione (GSH) content in the tissues were estimated by the method of CitationBeutler et al. (1963). The protein carbonyl content of the tissues were assayed according to the method of CitationEvans et al. (1999). Non-protein sulfhydryl (NPSH) contents in the heart and kidney were quantified using the DTNB [5,5′-dithiobis-(2-nitrobenzoic acid)] method (CitationEllman, 1959).
Statistical analysis
Values are expressed as mean ± SD for six samples in each group. Statistical analysis was done using one-way analysis of variance (ANOVA), and inter-group comparisons were made using Tukey’s multiple comparison test. A p value < 0.05 was considered as significant.
Results
Effect of hesperidin on body weight and organ weight of control and experimental animals
shows the effect of hesperidin on the body weight, heart weight, and kidney weight of control and test animals. Exposure to γ-radiation resulted in a significant decrease in the body weight and heart and kidney weights of rats when compared to the control rats (Group 1). Rats exposed to γ-radiation (Group 2) also showed decreased physical activity, characterized by a decreased uptake of food and water during the study period. Hesperidin treatments for 7 days caused a significant improvement in the body and organ weights, comparable with those of control rats.
Table 1. Effect of hesperidin treatment on body weight and organ weight of control and experimental animals.
Effect of hesperidin treatment on status of cardiac marker enzymes in serum of control and experimental animals
Exposure to γ-radiation caused a marked rise in serum levels of AST, ALT, ALP, LDH, and CPK () and a subsequent fall in their levels in the heart tissue () when compared to control rats, demonstrating marked myocardial damage. Treatment with hesperidin decreased the elevated levels of these cardiac marker enzymes in the serum. Both doses of hesperidin also attenuated the γ-radiation induced alterations in the levels of marker enzymes in the heart.
Table 2. Effect of hesperidin treatment on the status of AST, ALT, ALP, LDH, and CPK in the serum of rats exposed to various doses of γ-radiation.
Table 3. Effect of hesperidin treatment on the status of AST, ALT, ALP, LDH, and CPK in the heart tissue of rats exposed to γ-radiation.
Effect of hesperidin treatment on status of cardiac and renal lipid peroxidation levels in control and experimental animals
γ-Radiation challenge caused a marked elevation in lipid peroxidation in both the heart and the kidney when compared to unexposed control rats. Administration of hesperidin for 7 days decreased the level of lipid peroxidation in the heart and kidney, with maximum attenuation observed with the 100 mg/kg dose (). Further, oral administration of hesperidin alone (100 mg/kg) did not result in a significant alteration of either cardiac or renal lipid peroxidation levels.
Figure 1. Effect of hesperidin treatment on the status of lipid peroxidation (LPO) in the heart and kidney of rats exposed to γ-radiation. Values are expressed as mean ± SD for six rats in each group. Comparisons are made as: a, compared with Group 1; b, compared with Group 2. ***, statistical significance at p < 0.001. See Appendix 1 for key.
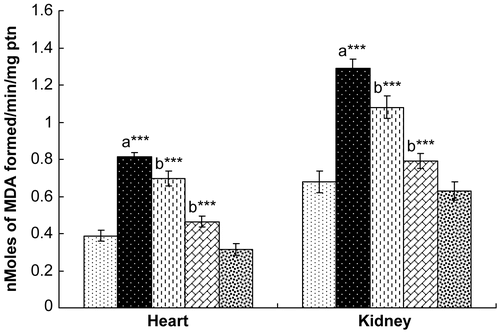
Effect of hesperidin treatment on antioxidant enzymes in heart and kidney of control and experimental animals
Rats exposed to γ-radiation showed significantly decreased levels of SOD, CAT, and GPx in both the heart and the kidney (). Treatment with hesperidin for 7 days resulted in a recovery in the levels of these endogenous antioxidant enzymes to normal levels in both the heart and kidney, comparable with those of control rats. Rats administered hesperidin alone (100 mg/kg) did not show any marked alterations in the levels of these antioxidant enzymes when compared with control rats.
Figure 2. Effect of hesperidin treatment on the activity of superoxide dismutase (SOD) in the heart and kidney of rats exposed to γ-radiation. Values are expressed as mean ± SD for six rats in each group. Comparisons are made as: a, compared with Group 1; b, compared with Group 2. ***, statistical significance at p < 0.001.
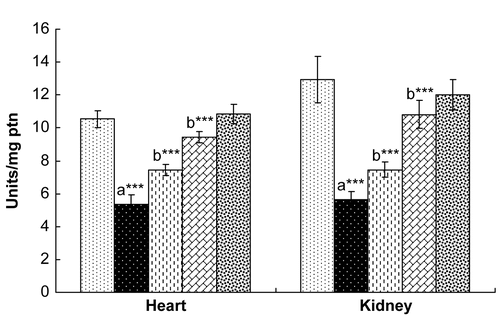
Figure 3. Effect of hesperidin treatment on the activity of catalase (CAT) in the heart and kidney of rats exposed to γ-radiation. Values are expressed as mean ± SD for six rats in each group. Comparisons are made as: a, compared with Group 1; b, compared with Group 2. ***, statistical significance at p < 0.001.
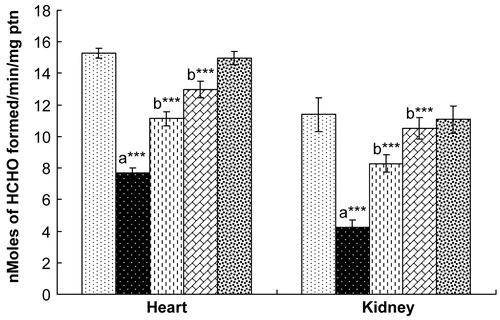
Figure 4. Effect of hesperidin treatment on the activity of glutathione peroxidase (GPx) in the heart and kidney of rats exposed to γ-radiation. Values are expressed as mean ± SD for six rats in each group. Comparisons are made as: a, compared with Group 1; b, compared with Group 2. ***, statistical significance at p < 0.001.
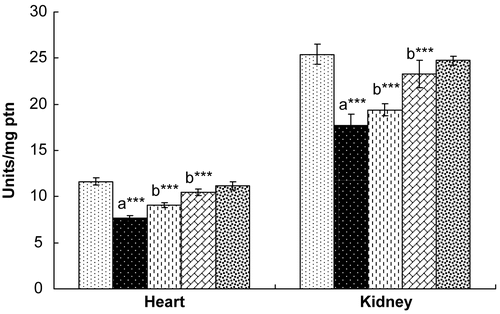
Effect of hesperidin treatment on glutathione, protein carbonyl content, and non-protein sulfhydryl (NPSH) content in control and experimental animals
Exposure to γ-radiation markedly decreased the levels of glutathione in both the heart and kidney, demonstrating oxidative stress (). A significant increase in protein carbonyl content () and decrease in the levels of non-protein thiols () in the heart and kidney of rats exposed to γ-radiation was also observed. Hesperidin treatment for 7 days significantly ameliorated the γ-radiation induced depletion of GSH in both heart and kidney and also stabilized the levels of protein carbonyls and non-protein thiols in the heart and kidney.
Figure 5. Effect of hesperidin treatment on the levels of glutathione (GSH) in the heart and kidney of rats exposed to γ-radiation. Values are expressed as mean ± SD for six rats in each group. Comparisons are made as: a, compared with Group 1; b, compared with Group 2. ***, statistical significance at p < 0.001.
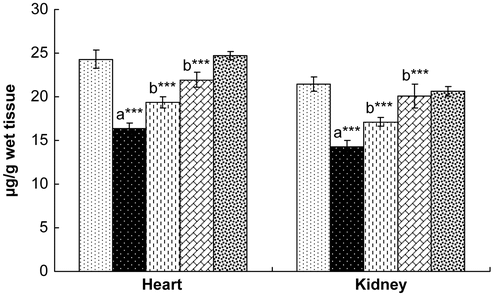
Figure 6. Effect of hesperidin treatment on the levels of protein carbonyl in the heart and kidney of rats exposed to γ-radiation. Values are expressed as mean ± SD for six rats in each group. Comparisons are made as: a, compared with Group 1; b, compared with Group 2. ***, statistical significance at p < 0.001.
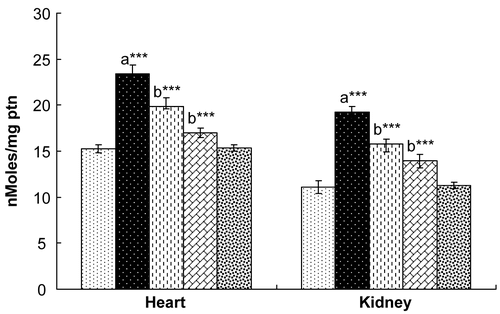
Figure 7. Effect of hesperidin treatment on the levels of non-protein thiols in the heart and kidney of rats exposed to γ-radiation. Values are expressed as mean ± SD for six rats in each group. Comparisons are made as: a, compared with Group 1; b, compared with Group 2. ***, statistical significance at p < 0.001.
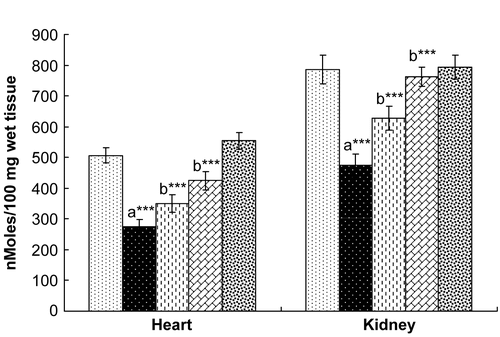
Effect of hesperidin treatment on histopathology of heart tissue of control and experimental animals
Light microscopy observation of rat heart tissues stained with hematoxylin and eosin revealed γ-radiation induced tissue damage characterized by cardiac muscles showing some debranching mononuclear cells and a damaged intercalated network (). Severe destruction to cardiac muscles was prevented upon treatment with hesperidin for 7 days. Likewise, the severity of damage in the intercalated network was also restricted in hesperidin administered rats. Rats treated with hesperidin alone for 7 days did not show any marked alteration in the cardiac architecture, which was comparable with that of control rats.
Discussion
In spite of being widely used in therapy, ionizing radiation is considered to be toxic to organisms because it induces deleterious structural changes in essential macromolecules of the cells (CitationNavarro et al., 1997). Exposure to γ-radiation is reported to cause cancer due to the resulting oxidative damage, and the molecule most often reported to be damaged by this physical agent is the DNA (CitationDaly et al., 1999). About 60–70% of cellular DNA damage produced by ionizing radiation is caused by hydroxyl (•OH), formed from the radiolysis of water. Besides DNA, lipids and proteins are also attacked by free radicals induced by ionizing radiation (CitationReiter, 2000). Hence, supplementation of exogenous dietary antioxidants might prove beneficial to patients undergoing radiotherapy and also to astronauts traveling in deep space.
In the present study, exposure of rats to γ-radiation (5 Gy) induced a significant increase in the activities of ALT and AST in serum and a fall in their levels in heart tissue. A similar increase in serum AST and ALT was observed by CitationMansour (2006) and CitationRamadan et al. (2002), and our observation is in agreement with the above reports. The increase in serum aminotransferase activities with γ-radiation may be due to the damage of cellular membranes of the heart tissue, which in turn leads to an increase in the permeability of cell membranes and facilitates the passage of cytoplasmic enzymes outside the cells, leading to an increase in aminotransferase activities in the systemic circulation (CitationKhamis & Roushdy, 1991). A decrease in serum ALP, LDH, and CPK in rats exposed to γ-radiation was also observed, which is an indication of myocardial damage. These changes in the sera of rats exposed to γ-radiation may be due to the leakage of enzymes from the heart as a result of necrosis induced by γ-radiation. Post-treatment with hesperidin for 7 days preserved the structural and functional integrity of the myocardial membrane, as evident from the significant lowering of these serum marker enzymes when compared with γ-radiation exposure, which establishes the cardioprotective effect of hesperidin.
Lipid peroxidation (LPO) is an important pathogenic event in radiation exposure. SOD, CAT, and GPx are anti-peroxidative enzymes that protect the cellular constituents against oxidative damage (CitationManda et al., 2007). A significant reduction in the activities of SOD, CAT, and GPx with a concomitant increase in LPO observed in rats exposed to γ-radiation is in agreement with the results of CitationPathak et al. (2007) and CitationMansour (2006). The above observed effect might be due to excessive generation of free radicals following radiation exposure, resulting in peroxidative membrane damage. Post-treatment with hesperidin significantly restored the levels of SOD, CAT, and GPx to near normal, and concomitantly decreased LPO levels, when compared to radiation exposed animals, which shows the membrane stabilizing activity of hesperidin.
Exposure to γ-radiation also caused a significant increase in the protein carbonyl content in the heart and kidney of rats. This might be due to oxidation of cellular proteins caused by ROS generated during radiation exposure. Hesperidin treatment caused a fall in the levels of protein carbonyls in rats exposed to γ-radiation, which is indicative of the inhibitory role of hesperidin against radiation induced protein oxidation. The radiation induced decrease in the levels of cellular GSH and NPSH was also ameliorated by hesperidin treatment, which further validates the hypothesis that hesperidin may scavenge the free radicals formed during oxidative stress. Studies have shown that the depletion of glutathione results in enhanced lipid peroxidation (CitationManda & Bhatia, 2003). Excessive lipid peroxidation can cause increased glutathione consumption (CitationComporti, 1987), as indicated by the NPSH values in the present study. The ability of hesperidin to restore the levels of cellular thiols indicates that it might play a role in GSH metabolism.
The biochemical findings are also supported by the histopathological observations of the heart tissue. Rats exposed to γ-radiation showed severe cardiac tissue damage, characterized by cardiac muscles showing debranching mononuclear cells and a damaged intercalated network. Damage to cardiac muscles was prevented in a dose dependent manner upon treatment with hesperidin for 7 days. Rats treated with hesperidin showed minimal damage to cardiac tissue and an improvement in the cardiac tissue architecture.
Several investigators have reported that phytochemicals with strong antioxidant activity such as silymarin, α-lipoic acid, carnitine, melatonin, ferulic acid, vanillin, etc. offer protection against radiation induced damage. The results of the present investigation have confirmed the usefulness of hesperidin as an effective antioxidant during exposure to γ-radiation. Further studies are under way to evaluate the protective effect of hesperidin on other cellular macromolecules such as DNA, protein, and lipids, and also on other tissues.
Appendix 1. Key to figures
Group 1: Control rats administered with saline alone
Group 2: Rats exposed to 5 Gy of γ-radiation
Group 3: Rats exposed to 5 Gy of γ-radiation + hesperidin (50 mg/kg bw, orally for 7 days)
Group 4: Rats exposed to 5 Gy of γ-radiation + hesperidin (100 mg/kg bw, orally for 7 days)
Group 5: Rats administered with hesperidin (100 mg/kg bw, orally for 7 days)
Acknowledgement
This work was supported by the nuclear research development project of the Korea Ministry of Science and Technology.
Declaration of interest: The authors report no conflicts of interest.
References
- Bergmeyer HU, Scheibe P, Wahlefeld AW (1978): Optimization of methods for aspartate aminotransferase and alanine aminotransferase. Clin Chem 24: 58–61.
- Beutler E, Duron O, Kelly BM (1963): Improved method for the determination of blood glutathione. J Lab Clin Med 61: 882–888.
- Bonina F, Lanza M, Montenegro L (1996): Flavonoids as potential protective agents against photo-oxidative skin damage. Int J Pharm 145: 87–94.
- Bradford MM (1976): A rapid and sensitive method for the quantitation of microgram quantities of protein utilizing the principle of protein-dye binding. Anal Biochem 72: 248–254.
- Comporti M (1987): Glutathione depleting agents and lipid peroxidation. Chem Phys Lipids 45: 143–169
- Daly JM, Bertagnoli M, De Cosse JJ, Morton DL (1999): Oncology. In: Schwartz SI, ed., Principles of Surgery, Vol. 1. New York, McGraw-Hill, pp. 335–347.
- Deng W, Fang WI, Wu J (1997): Flavonoids function as antioxidants: by scavenging reactive oxygen species or by chelating iron? Radiat Phys Chem 50: 271–276.
- Ellman GL (1959): Tissue sulfhydryl groups. Arch Biochem Biophys 82: 70–77.
- Evans P, Lyras L, Halliwell B (1999): Measurement of protein carbonyls in human brain tissues. Methods Enzymol 300: 145–157.
- Galati EM, Monforte MT, Kirjavainen S, Forertieri AM, Tripodo MM (1994): Biological effects of hesperidin – a citrus flavonoid. Part 1. Anti-inflammatory and analgesic activity. Farmaco 49: 709–712.
- Garg A, Garg S, Zaneveld LJD, Singla AK (2001): Chemistry and pharmacology of the citrus flavonoid hesperidin. Phytother Res 15: 655–669.
- Hannig J, Zhang, D, Canaday DJ, Beckett MA, Astumian RD, Weichselbaum RD, Lee RC (2000): Surfactant sealing of membranes permeabilized by ionising radiation. Radiat Res 154: 171–177.
- Hosseinimehr SJ, Nemati A (2006): Radioprotective effects of hesperidin against gamma irradiation in mouse bone marrow cells. Br J Radiol 79: 415–418.
- Jovanovic SV, Steenken S, Tosic M, Marjonovic B, Simic MG (1994): Flavonoids as antioxidants. J Am Chem Soc 116: 4846–4851.
- Khamis F, Roushdy HM (1991): Synergistic radio-protective action of imidazole and serotonin on serum and liver enzymes in rats. Arab J Sic Ape 24: 19–36.
- King J (1965a): The phosphohydrolases-acid and alkaline phosphatases. In: Practical Clinical Enzymology. London, Van D (Ed), Nostrand Co. Ltd., pp. 191–208.
- King J (1965b): The dehydrogenase (or) oxidoreductase – lactate dehydrogenase. In: Practical Clinical Enzymology. London, Van D (Ed), Nostrand Co. Ltd., pp. 83–93.
- Krolicki Z, Lamer-Zarawaska E (1984): Investigation of antifungal effect of flavonoids. Part 1. Herb Pol 30: 53–57.
- Malterud KE, Rydland KM (2000): Inhibitors of 15-lipoxygenase from orange peel. J Agric Food Chem 48: 5576–5580.
- Manda K, Bhatia AL (2003): Role of β-carotene against acetaminophen induced hepatotoxicity in mice. Nutr Res 23: 1097–1103.
- Manda K, Ueno M, Moritake T, Anzai K (2007): α-Lipoic acid attenuates x-irradiation-induced oxidative stress in mice Cell Biol Toxicol 23: 129–137.
- Mansour HH (2006): Protective role of carnitine ester against radiation-induced oxidative stress in rats. Pharmacol Res 54: 165–171.
- Miller NJ, Rice-Evans CA (1997): The relative contributions of ascorbic acid and phenolic antioxidants to the total antioxidant activity of orange and apple fruit juices and blackcurrant drink. Food Chem 60: 331–337.
- Nair CKK, Parida DK, Nomura T (2001): Radioprotectors in radiotherapy. J Radiat Res 42: 21–37.
- Navarro J, Obrador E, Pellicer JA., Asensi M, Vina J, Estrela JM (1997): Blood glutathione as an index of radiation-induced oxidative stress in mice and humans. Free Radic Bio Med 22: 1203–1209.
- Ohkawa H, Ohishi N, Yagi K (1979): Assay for lipid peroxides in animal tissues by thiobarbituric acid reaction. Anal Biochem 95: 351–358.
- Okinaka S, Kumogai H, Ebashi S, Sugitha H, Momoi H, Toyokura Y, Fujie Y (1961): Serum creatine phosphokinase activity in progressive muscular dystrophy and neuromuscular diseases. Arch Neurol 4: 520–525.
- Pathak CM, Avti PK, Kumar S, Khanduja KL, Sharma SC. (2007): Whole body exposure to low-dose gamma radiation promotes kidney antioxidant status in Balb/c mice. J Radiat Res 48: 113–120.
- Ramadan LA, Roushdy HM, Abu Senna GM, Amin NE, El-Deshw OA (2002): Radioprotective effect of silymarin against radiation induced hepatotoxicity. Pharmacol Res 45: 447–454.
- Reiter RJ (2000): Melatonin: Lowering the high price of free radicals. News Physiol Sci 15: 246–250.
- Rotruck JT, Pope AL, Ganther HE, Swanson AB, Hafeman DG, Hoekstra WG (1973): Selenium: Biochemical role as a component of glutathione peroxidase. Science 179: 588–590.
- Son HS, Kim HS, Ju JS (1991): Effects of rutin and hesperidin on total cholesterol concentration, transaminase and alkaline phosphatase activity in carbon tetrachloride treated rats. Hanguk Nonghwa Hakhoe Chi 34: 318–326.
- Suarez J, Herrera MD, Marhuenda E (1998): In vitro scavenger and antioxidant properties of hesperidin and neohesperidin dihydrochalcone. Phytomedicine 5: 469–473.
- Tominaga H, Kodama S, Matsuda N, Suzuki K, Watanabe M (2004): Involvement of reactive oxygen species (ROS) in the induction of genetic instability by radiation. J Radiat Res 45: 181–188.
- Yang M, Tanaka T, Hirose Y, Deguchi T, Mori H, Kawada Y (1997): Chemopreventive effects of diosmin and hesperidin on N-butyl-N-(4-hydroxybutyl)nitrosamine induced urinary bladder carcinogenesis in male ICR mice. Int J Cancer 7: 719–724.
- Yilmaz S, Yilmaz E (2006): Effects of melatonin and Vitamin E on oxidative–antioxidative status in rats exposed to irradiation. Toxicology 222: 1–7