Abstract
Context: Folium Eriobotryae (FE), the dry leaf of Eriobotrya japonica (Thunb.) Lindl. (Rosaceae), has been widely used to treat respiratory disorders.
Objective: To examine the bronchodilatory activity of FE and the potential mechanisms involved.
Materials and methods: The effects of ethyl acetate fraction of FE (EFE) (0.05–0.3 mg/mL) on the isolated tracheal strips, and ursolic acid (UA) (5–30 μg/mL) that was the main constituent of EFE, were tested in vitro. Meanwhile, acetylcholine (Ach) and histamine (His)-induced bronchospasm were conducted in vivo in guinea pig. Furthermore, mechanisms of relaxant effects of EFE and UA were evaluated in the absence and presence of specific inhibitors.
Results: With in vitro studies, the contractile response evoked by Ach or His (EC50 = 0.21 and 0.16 mg/mL) was decreased by EFE, and UA caused a concentration-dependent relaxation precontracted by His (EC50 = 23.2 μg/mL). With in vivo studies, EFE strongly prolonged preconvulsive time similar to isoprenalin. The bronchodilator effects of EFE could be blocked by propranolol (1 μM), NG-nitro-l-arginine methyl ester (l-NAME) (100 μM) and 1H-[1,2,4]oxadiazolo[4,3-a]quinoxaline-1-one (ODQ) (1 μM). EFE also inhibited the contraction in Ca2+-free medium and produced rightward parallel displacement of CaCl2 curves. In addition, the relaxant effects of UA could only be blocked by l-NAME and ODQ.
Discussion and conclusion: These results suggest that bronchodilator activities of EFE were related to activation of β-adrenoceptor and NO/cGMP pathway. Blockage of Ca2+ channels and inhibition of IP3R-mediated internal Ca2+ release were also involved. Additionally, UA produced relaxant effects by the NO/cGMP pathway.
Introduction
Asthma is a chronic airway inflammatory disease characterized by airway obstruction, hyperresponsiveness and remodeling (Bousquet et al. Citation2000). It is considered as a serious health problem and represents a major stake of public health in both developed and developing countries. According to the World Health Organization, there are approximately 300 million annual cases of asthma worldwide. Incidence of asthma has climbed dramatically, despite an increased use of medications that suppress airway inflammation and repress contraction of smooth muscle that encircles the airways. Asthmatic patients exhibit episodes of airway inflammation that potentiates reversible airway smooth muscle spasm (Khan et al. Citation2015). Current pharmacological management for controlling asthma is mainly based on corticoids as antiinflammatory agents in combination with β2-adrenergic agonists as bronchodilators (Chung et al. Citation2009). However, there is an increasing demand for finding new medicines in asthma therapy, as these drugs cause serious side effects. Natural products offer a remarkable pharmacological perspective for drug development with regard to their widespread acceptability and broad spectrum activities. So, it is valuable to search for effective medicines for treating asthma using plant resources.
Folium Eriobotryae (FE), the dry leaf of Eriobotrya japonica (Thunb.) Lindl. (Rosaceae), commonly known as Pipaye in China, has been recorded in the Chinese Pharmacopoeia (Committee of National Pharmacopoeia Citation2010). As a traditional Chinese medicine (TCM), FE was mainly used as an effective herb to treat respiratory diseases, and several other biological activities such as antioxidation (Bae et al. Citation2010), antidiabetes (Li et al. Citation2007), neuroprotective (Kim et al. Citation2011) and anticancer effects (Ito et al. Citation2002) have been reported. In the previous studies, FE was demonstrated to exert an obvious effect on ovalbumin-induced asthma (Jung et al. Citation2005) and relieve the symptoms of chronic bronchitis rats (Huang et al. Citation2006). The phytochemical studies on FE revealed the presence of various triterpenes, flavonoids, sesquiterpenes and tannins (Ferreres et al. Citation2009; Tan et al. Citation2015). Ursolic acid (UA), one of its main active ingredients, is popularly used in the treatment of cancer (Liu et al. Citation2012), hepatitis (Jin et al. Citation2012) and bacterial infections (Huang et al. Citation2009). It also plays an important role in respiratory diseases, which could inhibit ovalbumin-induced airway inflammation and attenuate lipopolysaccharide-induced acute lung injury in a mouse model (Chen et al. Citation2013; Kim et al. Citation2013). Moreover, it has been reported that UA possesses a relaxant effect in the vascular smooth muscle that depends on NO release (Aguirre-Crespo et al. Citation2006).
Nevertheless, despite the many studies carried out on the biological effects of FE and UA, there are no scientific data about its bronchodilatory effects. Therefore, the present study aimed to investigate the pharmacological action and possible mechanisms of the ethyl acetate fraction (EFE) and UA on isolated tracheal strips.
Materials and methods
Plant material and extraction
FE was provided by Tianjin Zhongxin Pharmaceutical Factory (Tianjin, China) and identified by Professor Wenyuan Gao (Tianjin University, China). The voucher specimens (voucher no. PPY150165) are available in the herbarium of Research Center of Tianjin Zhongxin Pharmaceuticals, Tianjin, China. FE (500 g) was extracted with 60% ethanol at 80 °C for 2 h, two times using the reflux extraction method and then extracted with 90% ethanol at the same conditions. The combined solution was filtered and concentrated under reduced pressure at a temperature below 50 °C. The extraction yield was 28.15%. Then, the ethanol extract was suspended with water and successively extracted with petroleum ether, ethyl acetate and n-butanol. The yields were 0.96, 3.20, 6.03 and 8.83% for petroleum ether fraction (PFE), ethyl acetate fraction (EFE), n-butanol fraction (NFE) and residuary water fraction (RFE), respectively. The fractions were dissolved in vehicle (normal saline +2% DMSO). Vehicles used had no effect on control experiments. In in vitro screening experiments, EFE showed the most active relaxing effect. In addition, UA accounted for 10% of EFE and was identified by HPLC.
Reagents
Verapamil (Ver), theophylline, glibenclamide (Gli), propranolol hydrochloride, indomethacin (INDO) and UA were purchased from National Institute for Control of Pharmaceutical and Biological Products (Beijing, China). Acetylcholine chloride (Ach), NG-nitro-l-arginine methyl ester (l-NAME), 1H-[1,2,4]oxadiazolo[4,3-a]quinoxaline-1-one (ODQ), tetraethylammonium chloride (TEA) and 4-aminopyridine (4-AP) were obtained from Sigma-Aldrich Co. (St. Louis, MO). Histamine dihydrochloride (His) was purchased from Tianjin Shilan Technology Co., Ltd. (Tianjin, China). Isoprenaline hydrochloride was obtained from Shanghai Harvest Pharmaceutical Co. (Shanghai, China). All chemicals used were of analytical grade.
Animals
Male guinea pigs (350–450 g) were purchased from Experimental Animal Center, Chinese Academy of Medical Sciences, Peking, SCXK-2011-0006. The animal study proposal was approved by the Institutional Animal Care and Use Committee (IACUC) of Institute of Radiation Medicine Chinese Academy of Medical Sciences (Tianjin, China) with the permit number IACUC2014-010. All animals were housed at the Animal Breeding Laboratory of Tianjin University of Traditional Chinese Medicine (Tianjin, China). All experimental protocols were approved by the Animal Ethics Committees of the Faculty of Medicine, Tianjin University, Tianjin, China, and carried out in accordance with “Principles of Laboratory Animal Care and Use in Research” (State Council of China 1988).
Analysis of chemical compounds of EFE by UPLC–Q–TOF/MS
EFE was accurately weighed (50 mg) and dissolved in 10 mL of methanol with the aid of ultrasound. An Agilent 1290 liquid chromatography system (Agilent Technologies, Santa Clara, CA), connected with the Agilent 6520 Q-TOF mass spectrometer (Agilent Technologies, Seattle, WA) via an ESI interface was used for the qualitative analysis. The separation was carried out on an ACQUITY UHPLC BEH C18 column (2.1 mm ×100 mm, 1.7 μm), with a column temperature maintained at 35 °C. The mobile phases A and B were acetonitrile and water, respectively. The flow rate was 0.5 mL/min, the injection volume was 5 μL and the gradient duration program was the following: 0–7 min, 10–20% A; 7–37 min, 20–50% A; 37–52 min, 50–60% A; 52–54 min, 60–65% A; 54–64 min, 65–71% A; 64–94 min, 71–100% A. The MS detection parameters were optimized as follows: drying gas temperature, 350 °C; drying gas (N2) flow rate, 10.0 sL/min; nebulizer gas pressure, 40 psig; fragmentor voltage, 150 V; capillary voltage, 4500 V; skimmer voltage, 65 V; octopole radio frequency voltage, 750 V. To gain more information on the structural identification, the sample was analyzed in negative ion mode. The collision energy was set at 15–40 V and the mass range was recorded from m/z 100 to 1000. Data acquisition and processing were controlled by Mass Hunter software (Agilent Technologies).
In vitro experiments
Preparation of isolated tracheal strips
Guinea pigs were euthanized by pentobarbital sodium (70 mg/kg, ip) and exsanguinated. The tracheas were then quickly removed, dissected and cut into 3–4 mm long strips. Tracheal strips were placed in an organ bath of 10 mL capacity containing the Krebs solution with the following composition (mM): NaCl 118.1, KCl 4.7, CaCl2 2.5, MgSO4 1.2, KH2PO4 1.2, NaHCO3 25.0 and glucose 11.1, bubbled with 95% O2 and 5% CO2 at 37 °C. Tissue was suspended under an isotonic tension of 1 g and allowed to equilibrate for at least 1 h, while it was washed with the Krebs solution for every 15 min. After equilibration, it was stimulated with 2 μM His to test their contractile responses and isometric contractions were measured by a force transducer connected to a computerized data acquisition system (BL-420F analyzer, Chengdu Instrument Corporation, Chengdu, China) (Khan et al. Citation2015).
Effects of EFE on the resting tracheal strips
The bronchodilator effects of different concentrations of EFE (0.075, 0.15 and 0.3 mg/mL), isoprenaline (0.01 μg/mL, as positive control), and DMSO (as control vehicle) were observed on the resting tracheal strips. Relaxant effects were defined as (Tb – Ta)/Tb ×100%. Tb was the resting force (in the absence of agonists) or steady-state contraction force (in the presence of agonists), and Ta was the force after adding the extracts for 20 min (Ge et al. Citation2013).
Effects of EFE on Ach- and His-induced tracheal contraction
The relaxant effects of EFE were evaluated in different concentrations (0.075, 0.15 and 0.3 mg/mL) under pre-contracted by Ach (2 μM) or His (2 μM). Furthermore, accumulative concentration–response curves (CRCs) of EFE for Ach (0.02–20 μM) or His (0.02–20 μM) were measured in the absence and presence of EFE (0.3 mg/mL).
Effects of EFE on basal tone of isolated tracheal strips
To determine the mediators involved in EFE-induced bronchodilators, the relaxant effect of EFE was evaluated (0.05–0.3 mg/mL, in the accumulative concentrations) on the pre-contracted tracheal strips by His (2 μM). Additionally, β-adrenoceptor inhibitor propranolol (1 μM), PDE enzyme inhibitor theophylline (100 μM), nonselective nitric oxide synthase (NOS) inhibitor l-NAME (100 μM), soluble guanylate cyclase (sGC) inhibitor ODQ (1 μM), and cyclooxygenase inhibitor INDO (10 μM) were used to pre-incubate the tracheal strip for 20 min before His-induced contraction. The non-incubation with inhibitors was considered as control.
Effects of EFE on extracellular Ca2+-induced contraction
To assess the possible roles of extracellular Ca2+ in the EFE-induced tracheal relaxation, the preparation was allowed to stabilize in the normal Krebs solution, which was then replaced with Ca2+-free solution containing EDTA (0.1 mM) for 30 min in order to remove Ca2+ from the tissues. The tracheal strip was then rinsed in the Ca2+-free and high-K+ (60 mM) Krebs solution (without EDTA). The CRCs for CaCl2 (0.03–30 mM) were obtained in the absence of EFE (control group) or after a 20 min incubation with EFE (0.075, 0.15 and 0.3 mg/mL). Finally, the contractile effect induced by CaCl2 was compared in the absence (control group) and presence of EFE. The non-incubation with EFE was considered as control. The incubation with the L-voltage calcium channel inhibitor Ver was considered as positive control (Guo et al. Citation2014).
Effects of EFE on the sarcoplasmic reticulum calcium release
To determine whether the relaxation induced by EFE was related to inhibition of intracellular Ca2+ release, the experiments were carried out in the Ca2+-free Krebs solution, and tracheal strips were allowed to stabilize in the Ca2+-free Krebs solution for 30 min. Then, EFE (0.05, 0.13, 0.22, 0.3 mg/mL) was used to pre-incubate the tracheal strips for 20 min before His (2 μM) was added to stimulate the release of intracellular Ca2+. The maximal tension induced by His in the control group (without EFE) was considered as 100% (Qu et al. Citation2014).
Role of K+ channel in EFE-induced tracheal relaxation
To test the involvement of K+ channel in EFE-induced tracheal relaxation, the effects of various K+ channel blockers were evaluated. The isolated trachea was pre-incubated with the K+ channel blockers, TEA (1 mM), Gli (0.01 mM) and 4-AP (0.1 mM) for 20 min before His (2 μM) was added. Once the plateau had attained, EFE (0.05, 0.11, 0.18, 0.24 and 0.3 mg/mL) was added, respectively (Qu et al. Citation2014). The non-incubation with inhibitors was considered as control.
Effects and mechanisms of UA on isolated tracheal strips
To assess the effects and mechanisms of UA (the main constituent of EFE, it accounted for 10% in EFE) on isolated guinea pig tracheal strips, same in vitro experiments were performed, as described previously. According to the content of UA in EFE, the concentrations of UA in experiments were ranging from 5 to 30 μg/mL (equal to EFE from 0.05 to 0.3 mg/mL).
In vivo experiments
To evaluate the bronchodilator activity of EFE in vivo, bronchoconstrictive challenge induced by Ach and His in guinea pigs was considered (Xu et al. Citation1991). Briefly, guinea pigs were numbered and individually placed into a 3000 mL special glass chamber that was placed upside down, and sprayed with a mixture of 2% Ach and 0.1% His (1:1, v/v) for 15 s using an electronic nebulizer. The time from the spraying of solution to the onset of tumble was recorded (preconvulsive time (PCT)). The guinea pigs with PCT between 30 and 120 s were considered as eligible. After 24 h of recovery, these selected guinea pigs were divided into 6 groups of 10 each randomly and treated orally for 7 days as follows: Group I was administrated with 0.5% CMC-Na, Group II (positive group) received isoprenaline (0.05 mg/kg, sc), Groups III–VI were treated with 200 (crude material mass) mg/kg of PFE, EFE, NFE and RFE, respectively. One hour after the last administration, guinea pigs were subjected to bronchoconstrictive challenge as preformed previously. Then, the above experiment was repeated with different concentrations of EFE [50, 125, 200 (crude material mass) mg/kg].
Statistical analysis
All experimental data were expressed as means ± standard error (SEM.) or percentage and analyzed for statistical significance using one-way analysis of variance (ANOVA) followed by Dunnett’s test. Tests were performed using SPSS 17.0 system (Chicago, IL); p value less than or equal to 0.05 was considered to be statistically significant.
Results
UPLC–Q–TOF/MS analysis of EFE
To determine the chemical composition of EFE, UPLC–Q–TOF/MS was used to separate and identify the constituents. The obtained total ion current (TIC) chromatogram in negative mode of EFE is illustrated in . Based on the retention time, accurate mass and characteristic MS2 fragments, a total of 35 compounds, including 18 triterpene acids, 10 flavonoids, 5 phenolic acids and 2 fatty acids, were identified or assigned tentatively by comparing with known compounds or consulting the respective literatures (). Moreover, the relative peak area was calculated as following: area of each peak/total area of peaks on TIC chromatogram ×100%. As shown in , the identified triterpene acids, flavonoids, phenolic acids, and fatty acids in EFE accounted for about 60.07, 18.79, 8.32 and 1.42%, respectively, and totalled to 88.60% of the total ion peak area.
Table 1. Compounds identified by UPLC–Q–TOF/MS in EFE and their relative content.
In vitro experiments
Effects of different fractions of FE on isolated tracheal strips
As shown in , all fractions of FE (200 (crude material mass) mg/mL) induced a relaxation in guinea pig tracheal strips. The EFE showed a stronger relaxant effect (22.75 ± 4.18%) than PFE (3.01 ± 1.35%), NFE (3.42 ± 2.68%), and RFE (1.25 ± 4.37%).
Figure 2. Influences of control (1) positive isoprenaline 0.01 μg/mL (2), the petroleum ether, ethyl acetate, n-butanol, and residuary water fraction of FE [200 mg (crude material mass)/mL] (3, 4, 5, 6, respectively) on the resting tension in guinea pig tracheal strips. Symbols and vertical bars represent means and SEM ANOVA followed by Dunnett’s multiple comparison test. Compared with control *p < 0.05, **p < 0.01 (n = 6).
![Figure 2. Influences of control (1) positive isoprenaline 0.01 μg/mL (2), the petroleum ether, ethyl acetate, n-butanol, and residuary water fraction of FE [200 mg (crude material mass)/mL] (3, 4, 5, 6, respectively) on the resting tension in guinea pig tracheal strips. Symbols and vertical bars represent means and SEM ANOVA followed by Dunnett’s multiple comparison test. Compared with control *p < 0.05, **p < 0.01 (n = 6).](/cms/asset/c8e4b578-a87d-4932-be7c-cde9bf675634/iphb_a_1183134_f0002_b.jpg)
Effects of EFE on isolated tracheal contraction
To further study the relaxant effects, the dose-effects of EFE were measured (). The percentage of relaxation at the lower (0.075 mg/mL), medium (0.15 mg/mL) or high concentration (0.3 mg/mL) was 10.31 ± 0.65, 25.66 ± 0.86 and 31.21 ± 1.50%, respectively. The high concentration-induced relaxation was similar to that of positive control (isoprenaline 29.72 ± 1.33%). In addition, pre-contraction in the tracheal strips induced by Ach and His were dramatically reduced by 6.02 ± 2.92, 34.24 ± 1.75, 88.71 ± 3.67% and 8.20 ± 1.71, 45.33 ± 4.53, 98.72 ± 3.16% at doses of 0.075, 0.15 and 0.3 mg/mL of EFE (EC50 = 0.21 and 0.16 mg/mL), respectively. The positive drug, isoprenaline, also induced a relaxation in the pre-contracted strip (). On the other hand, pretreatment with EFE (0.3 mg/mL) before adding Ach and His, the CRCs of Ach () and His () showed an unparallel rightward shift with reduced maximum contractile response of Ach and His to 36.40 ± 7.64 and 23.31 ± 6.52%, respectively.
Figure 3. Influences of control (a) positive isoprenaline 0.01 μg/mL (b) the ethyl acetate fraction at 0.075, 0.15 and 0.3 mg/mL (3, 4 and 5, respectively) on the non-precontracted (a) and pre-contracted strip by Ach and His (b). Influences of EFE (0.3 mg/mL) on cumulative Ach (c, 0.02–20 μM) or His (d, 0.02–20 μM)-induced contraction in guinea pig tracheal strips. Representative tracings of the contraction of trachea treated with Ach in response to (e) control and (f) EFE 0.3 mg/mL. Symbols and vertical bars represent means and SEM. ANOVA followed by Dunnett’s Multiple Comparison Test. *p < 0.05, **p < 0.01 (n = 6).
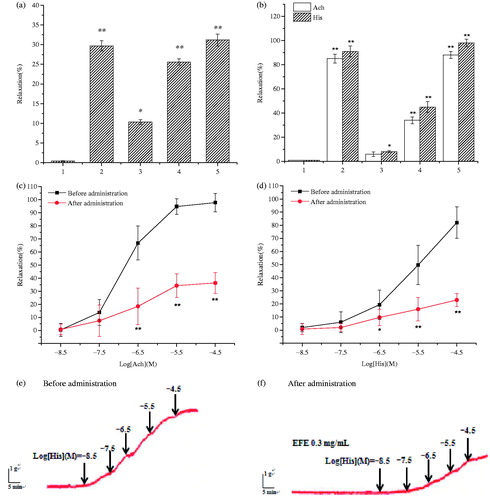
Mechanisms of the relaxant effects of EFE on tracheal strips
To evaluate the involvement of relaxing factors in the EFE-induced bronchodilator activity, the effects of propranolol (1 μM), a β-adrenoceptor inhibitor, theophylline (0.1 mM), a PDE inhibitor, l-NAME (0.1 mM), an NOS inhibitor, INDO (0.01 mM), an inhibitor of COX and ODQ (1 μM), and an sGC inhibitor were examined. As illustrated in , the tracheal relaxant effects of EFE were unaffected by theophylline and INDO, whereas pre-incubation with propranolol, l-NAME, and ODQ had significantly reduced relaxation with the maximum value of 60.23 ± 6.34, 57.18 ± 4.12, and 52.15 ± 4.51% () at a concentration of 0.3 mg/mL, respectively.
Figure 4. Effects of different inhibitors on EFE-induced bronchodilator in guinea pig tracheal strips. (a) Effect of propranolol 1 μM on EFE-induced relaxation. (b) Effect of theophylline 0.1 mM on EFE-induced relaxation. (e) Effect of l-NAME 0.1 mM on EFE-induced relaxation. (f) Effect of INDO 0.01 mM on EFE-induced relaxation. (g) Effect of ODQ 1 μM on EFE-induced relaxation. Representative force traces which show relaxation induced by EFE in tracheal strips pre-incubated with the (c) propranolol and (d) theophylline. Symbols and vertical bars represent means and SEM. ANOVA followed by Dunnett’s multiple comparison test. Compared with control *p < 0.05, **p < 0.01 (n = 6).
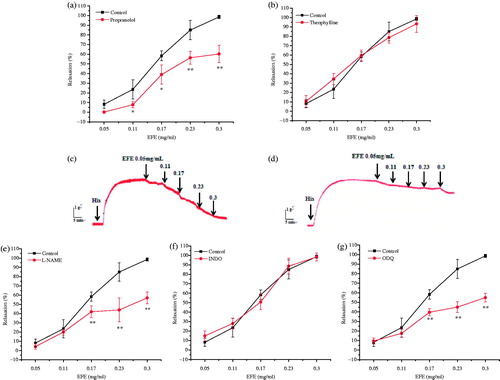
In order to determine whether the bronchodilator effects of EFE is mediated through the blockade of Ca2+ influx, a high concentration of K+ (60 mM) was used to depolarize the tissue. EFE (0.075, 0.15, 0.3 mg/mL) produced rightward parallel displacement of CaCl2 curves and decreased the maximum contraction induced by 3 × 10 − 2.5 M CaCl2 by 42.25 ± 2.57, 33.32 ± 5.41 and 26.71 ± 4.05%, respectively. Pre-incubation with Ver (0.01 mM) also dramatically reduced the maximum contraction to 23.31 ± 2.54% ().
Figure 5. (a) Dose-effect curves of CaCl2 on guinea pig tracheal strips in the absence and in the presence of EFE. (b) Bronchodilator effects of EFE on His pre-contracted tracheal strips in Ca2+-free solution. Symbols and vertical bars represent means and SEM. ANOVA followed by Dunnett’s multiple comparison test. Compared with control *p < 0.05, **p < 0.01 (n = 6).
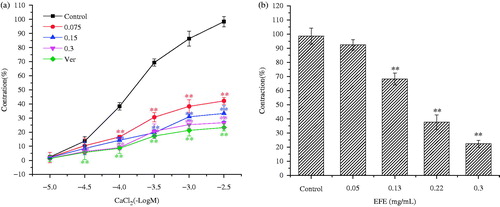
Then, the relaxant effects of EFE were assessed on the sarcoplasmic reticulum calcium release. In the Ca2+-free solution, His (2 μM) induced a transient contraction due to the release of intracellular Ca2+. Pre-incubation with EFE (0.05, 0.13, 0.22 and 0.3 mg/mL) for 20 min significantly attenuated His (2 μM)-induced contraction to 92.38 ± 3.60, 68.27 ± 4.33, 37.62 ± 5.27 and 22.40 ± 2.51%, respectively ().
To further evaluate the bronchodilator effects of EFE on tracheal strips, His-induced pre-contraction was performed in the presence of different pharmacological K+ channel blockers: nonselective K+ channel inhibitor TEA (1 mM), the KV channel inhibitor 4-AP (0.1 mM) and the KATP channel inhibitor Gli (0.01 mM). None of them had an effect on the EFE-induced response ().
Figure 6. Effects of the pretreatment with different K+ channels blockers on the EFE-induced relaxation in guinea pig tracheal strips. (a) Effect of TEA 1 mM on the EFE-induced relaxation. (b) Effect of 4-AP 0.1 mM on the EFE-induced relaxation. (c) Effect of Gli 0.01 mM on the EFE-induced relaxation. Symbols and vertical bars represent means and SEM. ANOVA followed by Dunnett’s multiple comparison test. Compared with control *p < 0.05, **p < 0.01 (n = 6).
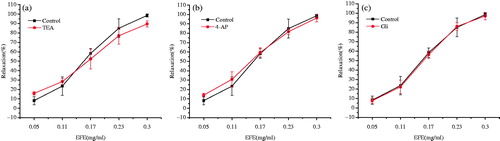
Effects and mechanisms of UA on isolated tracheal strips
UA caused a concentration-dependent relaxation of tracheal strips with an EC50 value of 23.2 μg/mL (). Compared with the relaxant effects of EFE at doses ranging from 0.05 to 0.3 mg/mL (containing UA 5–30 μg/mL), UA showed a lower relaxant effect than EFE at any doses (). In mechanism studies, only pre-incubation with l-NAME and ODQ have markedly reduced relaxation with the maximum value of 42.13 ± 2.4 and 37.23 ± 2.8% () at a concentration of 30 μg/mL, respectively. Other inhibitors had no effects on UA-induced bronchodilators (data not shown).
Figure 7. (a) Influences of UA from 5 to 30 μg/mL (equal to EFE from 0.05 to 0.3 mg/mL) and EFE from 0.05 to 0.3 mg/mL on the pre-contracted strip by His (2 μM). (b) Effect of l-NAME 0.1 mM on UA-induced relaxation. (c) Effect of ODQ 1 μM on UA-induced relaxation. Symbols and vertical bars represent means and SEM. ANOVA followed by Dunnett’s multiple comparison test. Compared with control *p < 0.05, **p < 0.01 (n = 6).
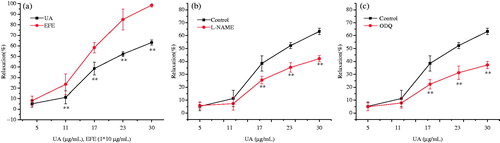
In vivo experiments
The bronchodilator effects of different fractions from FE in guinea pigs are shown in . Pretreatment of guinea pigs with isoprenaline had dramatically prolonged the PCT to 104.59%. PFE, EFE, NFE and RFE extended the PCT to 2.49, 90.54, 10.72 and 3.48%, respectively, compared with the data before administration, and the EFE had dramatically prolonged the PCT similar to isoprenalin. Besides, EFE showed dose-dependent bronchodilator effects in which the PCT was prolonged to 20.21 ± 1.41, 55.36 ± 0.80 and 90.12 ± 0.72% at doses of 50, 125 and 200 (crude material mass) mg/kg (EC50 = 114.3 mg/kg), respectively ().
Figure 8. Influences of control (1), positive isoprenaline 0.05 mg/kg (2), the EFE at 50, 125 and 200 (crude material mass) mg/mL (3, 4 and 5, respectively) on Ach and His-induced bronchospasm in guinea pigs. Symbols and vertical bars represent means and SEM. ANOVA followed by Dunnett’s multiple comparison test. Compared with control *p < 0.05, **p < 0.01 (n = 10).
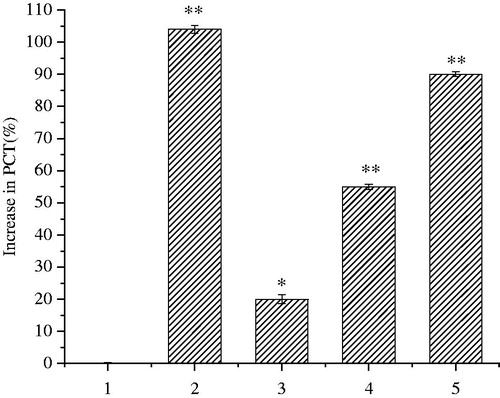
Table 2. Effects of different fractions of FE on Ach- and His-induced bronchospasm in guinea pigs.
Discussion
To the best of our knowledge, this is the first study on the bronchodilator effects induced by Folium Eriobotryae and UA. In the present research, EFE induced the highest relaxation on tracheal strips among the four fractions, which indicated that EFE was the most effective part. Therefore, we mainly focused on investigating the EFE-induced relaxation and the underlying mechanisms.
The UPLC–Q–TOF/MS analysis showed that EFE was mainly composed of 18 triterpene acids, including UA, UA derivatives, oleanolic acid, and oleanolic acid derivatives (UOAs, 60.07%, in relative peak area), 10 flavonoids (18.79%), 5 quinic acids and chlorogenic acids (QCAs, 8.32%), or 2 fatty acids (1.42%), which were probably more or less linked with the bronchodilator effects of EFE. Among them, the content of UOAs was the highest. UOAs have extensive biological activities, such as antispasmodic, antiinflammatory, antioxidant, and anticancer effects (Huang et al. Citation2009; Bae et al. Citation2010). The UOAs in FE have been used to treat respiratory disorders, which could relieve the symptoms of chronic bronchitis (Huang et al. Citation2006; Yang et al. Citation2012) and suppress lipopolysaccharide-induced cytokines or inducible enzyme production in lung epithelial cells (Huang et al. Citation2009). The vasorelaxant effects of UOAs, which from Phoradendron reichenbachianum Oliv. (Viscaceae) were mainly by NO production (Rios et al. Citation2012). UA, with the highest content in UOAs and EFE (, ), was reported to inhibit ovalbumin-induced airway inflammation (Kim et al. Citation2013) and exert relaxant effects in rat aortic rings via NO release (Aguirre-Crespo et al. Citation2006). The NO pathway was also involved in the vasodilatation effects of oleanolic acid (Rodríguez-Rodríguez et al. Citation2004). Another triterpene acid in EFE, pomolic acid, displayed the relaxation action in rat aortic rings by the NO/cGMP signaling pathway (Estrada et al. Citation2011). Taken together, the NO/cGMP pathway, one of the mechanisms of EFE for bronchodilator effects, may be contributed by UOAs. In addition, it has been demonstrated that several flavonoids possess antispasmodic effects (Ko et al. Citation2003). For examples, rutin, a natural flavonoid present in many herb families, exhibited dose-dependent relaxation effects in rat duodenum (Chen et al. Citation2001). Epicatechin might be one candidate for the airway relaxant activity, as catechin, its stereoisomer, has been shown to induce the bronchodilator activity on guinea pig tracheas (Ghayur et al. Citation2007). Therefore, we believe that the relaxation effects of EFE were related to the complexity in chemical composition and the UOAs may be the main contributor.
To assess bronchodilator effects of EFE and UA, there were studies on isolated tracheal strips. The present data showed that EFE significantly relaxed the resting or pre-contracted tracheal strips and produced an unparallel rightward shift of the CRCs of Ach and His, implying that the relaxing effects on the contraction induced by Ach and His in an uncompetitive manner. When bronchoconstriction is provoked by His, EFE is more active than Ach-induced contraction, so His was chosen as a stimulant in the following studies. The bronchodilator effects of UA were also researched and the UA displayed a lower relaxation effect than EFE at doses ranging from 5 to 30 μg/mL (equal to EFE from 0.05 to 0.3 mg/mL) (). Additionally, we evaluated the bronchodilator effects of EFE in vivo, the result implied that EFE was the most effective part, which dramatically prolonged the PCT in guinea pigs. But, the relaxation effects of UA in vivo were not tested, because it has been reported that in the similar experiment, UA could reach the same effect with a high dose of 120 mg/mL (Ju et al. Citation2003). In present studies, the content of UA in FE is low and there is no sense in comparing UA with EFE at irrelevant doses. In view of the above results, EFE is more potent than UA for the bronchodilatory effect both in vitro and in vivo. The reason why there was such a phenomenon might be related to the complexity in the chemical composition of EFE. We hypothesize that one or more elements in EFE may play a contraction or relaxant role on tracheas, which may be the synergistic or antagonistic effect of some chemical constituents, and therefore make the antiasthma effect of EFE more potent than that of UA.
To elucidate the possible mechanisms, the effects of bronchodilation were studied in the presence of the β-adrenoceptor, PDE, COX, NOS and sGC inhibitors. Propranolol, l-NAME and ODQ could inhibit the relaxant activity induced by EFE, whereas theophylline and INDO did not modify the relaxation, suggesting that bronchodilator effects were not related to the inhibition of PDE and prostaglandins. It is widely known that stimulation of β-adrenoceptor by agonists leads to an increase of adenylyl cyclase activity, which resulted in an increase of intracellular cyclic 3,5-adenosine monophosphate (cAMP) levels. The mechanism for cAMP action is via the stimulation of protein kinase A to phosphorylate a variety of target proteins to induce airway smooth muscle cell relaxation (Raeburn & Karlsson Citation1993). In this research, propranolol, a β-adrenoceptor inhibitor, inhibited the activity of EFE on the tracheal strip (), which indicated that β-adrenoceptor would take part in the bronchodilator effects induced by EFE. Besides, it is reported that endogenous NO stimulates sGC, which in turn generates intracellular cGMP and causes airway smooth muscle relaxation (Liu et al. Citation2008). Based on this observation, a possible modulatory action of NO on His-induced contraction was evaluated in the present study. When pre-incubated with l-NAME, an NOS inhibitor, the relaxant effects of EFE and UA were significantly reduced in tracheal strips, which were suggestive of the involvement of NO system (). Furthermore, there is a clear evidence that NO produces the relaxant response in airway smooth muscle through cGMP-dependent mechanism (Liu et al. Citation2008). So, we further investigated the possible requirement of cGMP pathway in the relaxant action of EFE and UA. The inhibitor of soluble sGC, ODQ blocked the bronchodilator response evoked by EFE and UA (). Therefore, we confirmed that the tracheal relaxation induced by EFE and UA were mediated by the NOS–NO–cGMP signaling pathway.
In many investigations, various spasmolytic constituents presented in plants usually mediate their relaxant effects through calcium antagonisms (Guo et al. Citation2014). In smooth muscle cells, there are two kinds of Ca2+ channels: voltage-dependent Ca2+ channels (VDCCs) and receptor-operated Ca2+ channels (ROCCs). As for VDCCs, high KCl-induced contraction is due to membrane depolarization, leading to increased Ca2+ influx through VDCCs (Qu et al. Citation2014). As for ROCs, the interaction of the contractile agonist phenylephrine and norepinephrine with their receptors induces the generation of inositol triphosphate (IP3) and diacylglycerol that activate protein kinase C (Karaki et al. Citation1997). IP3 binds to its receptor in the sarcoplasmic reticulum. The calcium released from the sarcoplasmic reticulum induces an initial transient contraction and subsequently opens the receptor linked-calcium channels, which may be activated by IP3 (Spedding & Cavero Citation1984). EFE could inhibit the contraction triggered by His in tracheal strips in Ca2+-free medium, suggesting that it also possibly interferes with the binding of agonists to receptors and inhibits Ca2+ mobilization from intracellular stores by a possible IP3 signaling blockade. EFE was capable of inhibiting contractility induced by Ach or His in airway smooth muscle (), and Ach or His induced contractile effects in tracheal smooth muscle by increasing intracellular Ca2+ (Ge et al. Citation2013). We also found that EFE remarkably blocked CaCl2-induced contraction, which was similar to the L-voltage calcium channel inhibitor Ver, in Ca2+-free medium containing 60 mM KCl, supporting the idea that EFE possesses a Ca2+ entry blocking activity. These results indicate that EFE might obstruct both voltage-dependent and receptor-operated Ca2+ channels. In summary, EFE inhibited Ca2+ released from intracellular stores and blocked extracellular Ca2+ entry, which suggests that EFE would be acting as calcium antagonist (Qu et al. Citation2014). K+ channels are important in regulating airway smooth muscle excitability or force generation, and a diverse range of K+ channels have been described in the plasmalemma of airway smooth muscle cells (Ge et al. Citation2013). They are Ca2+-activated K+ channels (KCa), voltage-dependent K+ channels (KV), ATP-sensitive K+ channels (KATP), and inward rectifier K+ channels (Kir) (Nelson & Quayle Citation1995). The opening of K+ channels in airway smooth muscle cells induces hyperpolarization of the membrane potential, an important mechanism to bronchodilator effects. So, we further investigated whether K+ channels mediated the relaxant action. EFE and UA-induced bronchodilation was not significantly attenuated by TEA, a nonselective KCa channel blocker, Gli, a KATP channel blocker or 4-AP, a voltage-gated K+ channel blocker, suggesting that EFE and UA-induced relaxation is unrelated with the activation of K+ channels. In summary, the mechanisms and pathways responsible for EFE and UA-induced bronchodilator effects are depicted in .
Figure 9. Schematic mechanisms of the bronchodilator effect of EFE and UA on isolated tracheal strips. cAMP: cyclic adenosine monophosphate; ATP: adenosine triphosphate; PKA, protein kinase A; sGC: soluble guanylyl cyclase; cGMP: cyclic guanosine monophosphate; GTP: guanosine triphosphate; VDCCs: voltage-dependent Ca2+ channels; ROCCs: receptor-operated Ca2+ channels.
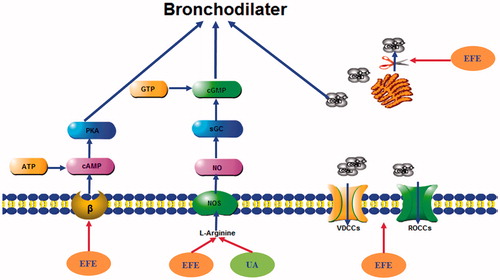
The β-adrenoceptor agonists alone are considered very effective bronchodilators, but have limited therapeutic uses due to cardiac stimulation (tachycardia), as a side effect (Raeburn & Karlsson Citation1993). On the other hand, calcium channel blockers are known to cause relaxation of smooth muscle as well as cardiac depression. The co-existence of calcium channel blockers constituents with β-adrenoceptor agonists is likely to offset the cardiac stimulation associated with β-adrenoceptor agonists when used alone. Additionally, it is widely known that both inflammation and bronchospasm play an essential role in asthma diseases (Bousquet et al. Citation2000). Thus, it will be of great significance if the plant has bronchodilator effects as well as anti-inflammatory function. Many studies have proved that FE and UA have an anti-inflammatory effect, which will contribute to the therapeutic effect on asthma (Huang et al. Citation2006, Citation2009).
Conclusions
In conclusion, the results suggest that EFE induces relaxation in isolated tracheal strips through the activation of β-adrenoceptor and NO/cGMP pathways. Blockage of Ca2+ channels and inhibition of IP3R-mediated internal Ca2+ release from intracellular stores were also involved. In addition, activation of NO/cGMP pathway is the bronchodilator mechanism of UA.
Disclosure statement
The authors have no conflict of interest in this research.
References
- Aguirre-Crespo F, Vergara-Galicia J, Villalobos-Molina R, López-Guerrero JJ, Navarrete-Vázquez G, Estrada-Soto S. 2006. Ursolic acid mediates the vasorelaxant activity of Lepechinia caulescens via NO release in isolated rat thoracic aorta. Life Sci. 79:1062–1068.
- Bae D, You Y, Yoon HG, Kim K, Lee YH, Kim Y, Baek H, Kim S, Lee J, Jun W. 2010. Protective effects of loquat (Eriobotrya japonica) leaves against ethanol-induced toxicity in HepG2 cells transfected with CYP2E1. Food Sci Biotechnol. 19:1093–1096.
- Bousquet J, Jeffery PK, Busse WW, Johnson M, Vignola AM. 2000. Asthma. From bronchoconstriction to airways inflammation and remodeling. Am J Respir Crit Care Med. 161:1720–1745.
- Chen XJ, Wan Y, Zhou TY, Li J, Wei Y. 2013. Ursolic acid attenuates lipopolysaccharide-induced acute lung injury in a mouse model. Immunotherapy. 5:39–47.
- Chen YC, Shen SC, Lee WR, Hou WC, Yang LL, Lee TJ. 2001. Inhibition of nitric oxide synthase inhibitors and lipopolysaccharide induced inducible NOS and cyclooxygenase-2 gene expressions by rutin, quercetin, and quercetin pentaacetate in RAW 264.7 macrophages. J Cell Biochem. 82:537–548.
- Chung KF, Caramori G, Adcock IM. 2009. Inhaled corticosteroids as combination therapy with beta-adrenergic agonists in airways disease: present and future. Eur. J. Clin. Pharmacol. 65:853–871.
- Committee of National Pharmacopoeia. 2010. Pharmacopoeia of People’s Republic of China (I). Vol. 57. Beijing (China): Chemical Industry Press.
- Estrada O, González-Guzmán JM, Salazar-Bookaman M, Fernández AZ, Cardozo A, Alvarado-Castillo C. 2011. Pomolic acid of Licania pittieri elicits endothelium-dependent relaxation in rat aortic rings. Phytomedicine. 18:464–469.
- Ferreres F, Gomes D, Valentão P, Gonçalves R, Pio R, Chagas EA, Seabra RM, Andrade PB. 2009. Improved loquat (Eriobotrya japonica Lindl.) cultivars: variation of phenolics and antioxidative potential. Food Chem. 114:1019–1027.
- Ge Y, Dai Q, Wan DR, Liu QH, Mei ZN. 2013. Relaxant effect of 1-butanol fraction from Elaeagnus pungens leaf through inhibiting L-type Ca2+ channel on guinea pig tracheal smooth muscle. J Ethnopharmacol. 150:196–201.
- Ghayur MN, Khan H, Gilani AH. 2007. Antispasmodic, bronchodilator and vasodilator activities of (+)-catechin, a naturally occurring flavonoid. Arch Pharm Res. 30:970–975.
- Guo HM, Zhang JZ, Gao WY, Qu Z, Liu CX. 2014. Gastrointestinal effect of methanol extract of Radix Aucklandiae and selected active substances on the transit activity of rat isolated intestinal strips. Pharm Biol. 52:1141–1149.
- Huang Y, Li J, Cao Q, Yu SC, Lv XW, Jin Y, Zhang L, Zou YH, Ge JF. 2006. Anti-oxidative effect of triterpene acids of Eriobotrya japonica (Thunb.) Lindl. leaf in chronic bronchitis rats. Life Sci. 78:2749–2757.
- Huang Y, Li J, Meng XM, Jiang GL, Li H, Cao Q, Yu SC, Lv XW, Cheng WM. 2009. Effect of triterpene acids of Eriobotrya japonica (Thunb.) Lindl. leaf and MAPK signal transduction pathway on inducible nitric oxide synthase expression in alveolar macrophage of chronic bronchitis rats. Am J Chinese Me. 37:1099–1111.
- Huang Y, Nikolic D, Pendland S, Doyle BJ, Locklear TD, Mahady GB. 2009. Effects of cranberry extracts and ursolic acid derivatives on P-fimbriated Escherichia coli, COX-2 activity, pro-inflammatory cytokine release and the NF-kappabeta transcriptional response in vitro . Pharm Biol. 47:18–25.
- Ito H, Kobayashi E, Li SH, Hatano T, Sugita D, Kubo N, Shimura S, Itoh Y, Tokuda H, Nishino H, Yoshida T. 2002. Antitumor activity of compounds isolated from leaves of Eriobotrya japonica. J Agric Food Chem. 50:2400–2403.
- Jin YR, Jin J, Li CH, Piao XX, Jin NG. 2012. Ursolic acid enhances mouse liver regeneration after partial hepatectomy. Pharm Biol. 50:523–528.
- Ju JH, Zhou L, Lin G, Liu D, Wang L, Yang J. 2003. Studies on constituents of triterpene acids from Eriobotrya japonica and their anti-inflammatory and antitussive effects. Chin Pharm J. 38:752–756.
- Jung JY, Yim YK, Lee H. 2005. The effects of Eriobotryae Folium herbal-acupuncture at ST36 on ovalbumin-induced asthma in C57BL mouse. Korean J Acupunct. 22:91–107.
- Karaki H, Ozaki H, Hori M. 1997. Calcium movements, distribution, and functions in smooth muscle. Pharmacol Rev. 49:157–230.
- Khan M, Shah AJ, Gilani AH. 2015. Insight into the bronchodilator activity of Vitex negundo. Pharm Biol. 53:340–344.
- Kim MJ, Lee J, Seong AR, Lee YH, Kim YJ, Baek HY, Kim YJ, Jun WJ, Yoon HG. 2011. Neuroprotective effects of Eriobotrya japonica against β-amyloid-induced oxidative stress and memory impairment. Food Chem Toxicol. 49:780.
- Kim SH, Hong JH, Lee YC. 2013. Ursolic acid, a potential PPARγ agonist, suppresses ovalbumin-induced airway inflammation and Penh by down-regulating IL-5, IL-13, and IL-17 in a mouse model of allergic asthma. Eur J Pharmacol. 701:131–143.
- Ko WC, Liu PY, Chen JL, Leu IJ, Shih CM. 2003. Relaxant effect of flavonoids in isolated guinea pig trachea and their structure-activity relationships. Planta Med. 69:1086–1090.
- Li WL, Wu JL, Ren BR, Chen J, Lu CG. 2007. Pharmacological studies on anti-hyperglycemic effect of Folium Eriobotryae. Am J Chin Med. 35:705–711.
- Liu B, Yang J, Wen QS, Li Y. 2008. Isoliquiritigenin, a flavonoid from licorice, relaxes guinea-pig tracheal smooth muscle in vitro and in vivo: role of cGMP/PKG pathway. Eur J Pharmacol. 587:257–266.
- Liu WB, Tan XB, Shu L, Sun H, Song J, Jin P, Yu SM, Sun M, Jia X. 2012. Ursolic acid inhibits cigarette smoke extract-induced human bronchial epithelial cell injury and prevents development of lung cancer. Molecules. 17:9104–9115.
- Nelson MT, Quayle JM. 1995. Physiological roles and properties of potassium channels in arterial smooth muscle. Am J Physiol. 268:799–822.
- Qu Z, Zhang JZ, Gao WY, Chen H, Guo H, Wang T, Li HF, Liu C. 2014. Vasorelaxant effects of Cerebralcare Granule are mediated by NO/cGMP pathway, potassium channel opening and calcium channel blockade in isolated rat thoracic aorta. J Ethnopharmacol. 155:572–579.
- Raeburn D, Karlsson JA. 1993. Effects of isoenzyme-selective inhibitors of cyclic nucleotide phosphodiesterase on microvascular leak in guinea pig airways in vivo. J Pharmacol Exp Ther. 267:1147–1152.
- Rios MY, López-Martínez S, López-Vallejo F, Medina-Franco JL, Villalobos-Molina R, Ibarra-Barajas M, Navarrete-Vazquez G, Hidalgo-Figueroa S, Hernández-Abreu O, Estrada-Soto S. 2012. Vasorelaxant activity of some structurally related triterpenic acids from Phoradendron reichenbachianum (Viscaceae) mainly by NO production: Ex vivo and in silico studies. Fitoterapia. 83:1023–1029.
- Rodríguez-Rodríguez R, Herrera MD, Perona JS, Ruiz-Gutiérrez V. 2004. Potential vasorelaxant effects of oleanolic acid and erythrodiol, two triterpenoids contained in ‘orujo' olive oil, on rat aorta. Br J Nutr. 92:635–642.
- Spedding M, Cavero I. 1984. ‘Calcium antagonists’: a class of drugs with a bright future. Part II. determination of basic pharmacological properties. Life Sci. 35:575–587.
- Tan H, Ashour A, Katakura Y, Shimizu K. 2015. A structure–activity relationship study on antiosteoclastogenesis effect of triterpenoids from the leaves of loquat (Eriobotrya japonica). Phytomedicine. 22:498–503.
- Xu SY, Bian RL, Chen X. 1991. Pharmacological experiment methodology people's. Beijing (China): Medical Publishing House.
- Yang Y, Huang Y, Huang C, Lv X, Liu L, Wang Y, Li J. 2012. Antifibrosis effects of triterpene acids of Eriobotrya japonica (Thunb.) Lindl. leaf in a rat model of bleomycin-induced pulmonary fibrosis. J Pharm Pharmacol. 64:1751–1760.