Abstract
Context: The genus Xylopia L. (Annonaceae) includes aromatic plants that have both nutritional and medicinal uses. Essential oils of Xylopia species have antitumour effects. However, the efficacy of the essential oil from the fruit of Xylopia langsdorffiana St. Hil & Tul. (EOX) has not been examined.
Objective: EOX was evaluated to determine its chemical composition, antitumour activity and toxicity.
Materials and methods: EOX was obtained from fresh fruits of X. langsdorffiana subjected to hydrodistillation, and gas chromatography-mass spectrometry was used to characterize the chemical composition of EOX. The toxicity of EOX was evaluated using haemolysis, acute toxicity and micronucleus assays. The in vitro antitumour activity of EOX was investigated using the sulforhodamine B assay. The sarcoma 180 murine tumour model was used to evaluate the in vivo antitumour activity and toxicity of EOX (50 and 100 mg/kg) after 7 d of treatment.
Results: The major components of EOX were α-pinene (34.57%) and limonene (31.75%). The HC50 (concentration producing 50% haemolysis) was 293.6 μg/ml. EOX showed greater selectivity for the leukaemia cell line K562, with total growth inhibition (TGI) (concentration producing TGI) of 1.8 μg/ml, and for multidrug-resistant ovarian tumour cell line NCI/ADR-RES (TGI of 45.4 μg/ml). The LD50 was approximately 351.09 mg/kg. At doses of 50 and 100 mg/kg, EOX inhibited the in vivo growth of sarcoma 180 by 38.67 and 54.32%, respectively. EOX displayed minor hepatic alterations characteristic of acute hepatitis and induced no genotoxicity.
Conclusion: EOX showed in vitro and in vivo antitumour activity and low toxicity, which warrants further pharmacological studies.
Keywords:
Introduction
Cancer is a complex genetic disease, characterized by unlimited growth, invasion, angiogenesis and metastasis. It is considered a major cause of death worldwide (Hanahan & Weinberg Citation2011).
Natural products, especially from plants, represent a fertile ground for the development of novel drugs. Among the products obtained from plants, essential oils have also been used as therapeutic agents since ancient times. For example, lavender oil, rosemary oil, eucalyptus oil and chamomile oil are used for insomnia, digestive problems, muscle aches and inflammation, respectively (Wei & Shibamoto Citation2007). Essential oils are natural, complex, multi-component systems composed mainly of terpenes in addition to some other non-terpene components. Essential oils and their constituents possess different biological activities, including antioxidant, antimicrobial, antifungal, anti-inflammatory and anticancer activities, and the last has been demonstrated in a number of human cancer cell lines (Edris Citation2007; Farag Citation2009; Tung et al. Citation2010; Mohsenzadeh et al. Citation2011; Sobral et al. Citation2014; Castro et al. Citation2015).
Essential oils may be useful in cancer prevention and treatment through various effects such as antioxidant, antimutagenic and antiproliferative, or by enhancing immune function and surveillance, inducing enzymes for enhanced detoxification and modulating multidrug resistance. A recent review of the anticancer activity of essential oils concluded that the theory of synergistic action appears to be of significance, and therefore, for biological purposes, it is more important to study the whole essential oil rather than its components separately (Bhalla et al. Citation2013).
Annonaceae is a large family known for its edible fruits and medicinal properties, including antitumour properties (Bakarnga-Via et al. Citation2014). The genus Xylopia L. includes aromatic plants that have both nutritional and medicinal uses (Moreira et al. Citation2013). The essential oils from X. aethiopica (Dunal) A. Rich. and X. parviflora (A. Rich.) Benth. have demonstrated cytotoxicity in different tumour cell lines (Bakarnga-Via et al. Citation2014; Woguem et al. Citation2014). Moreover, the essential oils of X. frutescens Aubl. (Ferraz et al. Citation2013) and X. laevigata (Mart.) R.E. Fries (Quintans et al. Citation2013) exhibit in vivo anticancer effects (sarcoma 180 model).
There are few reports in the literature on X. langsdorffiana St.-Hil. & Tul., known popularly as ‘pimenteira-da-terra’. Some diterpenes isolated from this species have shown cytotoxic effects on leukemic cells (Castello-Branco et al. Citation2009, Citation2011) and murine sarcoma 180 in vivo (Pita et al. Citation2012).
This study determined the chemical composition, antitumour activity and toxicity of the essential oil from X. langsdorffiana fruit (EOX).
Materials and methods
Drugs and reagents
RPMI 1640 culture medium, glutamine, penicillin, streptomycin, 4-(2-hydroxyethyl)-1-piperazineethanesulfonic acid (HEPES) and foetal bovine serum (FBS) were obtained from GIBCO (Carlsbad, CA). 5-Fluorouracil (5-FU), Triton X-100, Tween-80, cyclophosphamide, Trizma base and sulforhodamine (SRB) were purchased from Sigma-Aldrich (St. Louis, MO). Dimethylsulfoxide (DMSO) was purchased from Mallinckrodt Chemicals (Phillipsburg, NJ). Kits for biochemical analysis were purchased from LABTEST (Lagoa Santa, MG, Brazil). Sodium thiopental (Thiopentax®) was purchased from Cristália (Itapira, SP, Brazil) and heparin (Parinex®) from Hipolabor (Sabará, MG, Brazil). Doxorubicin (DOX) was from Tecnofarma International (Uruguay) and trichloroacetic acid (TCA) from Merck (Darnstadt, Germany).
Plant material
Xylopia langsdorffiana fruits were collected in July 2012 in Santa Rita, Paraíba State, Brazil. The species was identified by Dr. Maria de Fátima Agra. Voucher specimen number AGRA 5541 was deposited at the Herbarium Lauro Pires Xavier of the Federal University of Paraíba, Brazil.
Hydrodistillation of the volatile constituents
Fresh fruits (100 g) of X. langsdorffiana were subjected to hydrodistillation for 4 h using a Clevenger-type apparatus. The essential oil was dried with anhydrous sodium sulphate and filtered. The percentage content (v/w) was calculated on the basis of the fresh weight of plant material.
GC analysis
CG was performed with a Shimadzu GC17-A chromatograph, using a DB-5 fused sílica capillary column (30 m × 0.25 mm i.d., film thickness of 0.25 μm). Helium was used as the carrier gas, at a flow rate of 1.0 ml/min. The oven temperature was programmed at 60–240 °C at 3 °C/min. The injector and detector temperatures were 220 and 230 °C, respectively.
GC/MS analysis
Gas chromatography-mass spectrometry (CG-MS) was carried out in a Shimadzu QP-5000-Quadrupole MS system, operating with the ionizing energy of 70 eV. The DB-5 fused silica capillary column (30 m × 0.25 mm i.d., film thickness of 0.25 μm) was used. Helium was used as the carrier gas, at a flow rate of 1.0 ml/min. The oven temperature was programmed at 60–240 °C at 3 °C/min. The injector and detector temperatures were 220 and 230 °C, respectively. The substances were identified by comparison of their mass spectra with GC-MS databank system (NIST/EPA/HIH Mass Spectral Library Citation2005) and Kovats retention index (Adams Citation1995). The retention indices of the substances were obtained by coinjection of the essential oil with a standard mixture of hydrocarbons (C9-C24) and applying the formula of Van den Dool and Kratz (Citation1963). It was performed using quantitative standards: α-pinene and limonene. We obtained the average of three calibration curves.
Cell lines
The tumour cell lines utilized were: U251 – glioma, MCF-7 – breast, NCI/ADR-RES – multidrug-resistant ovarian, 786-0 – kidney, NCI-H460 – non-small cell lung cancer, PC-3 – prostate, OVCAR – ovarian, HT29 – colon and K562 – leukaemia, and HaCaT human keratinocytes served as the normal cell line. The cells lines were cultivated in RPMI-1640 supplemented with 10% FBS, 2 mm glutamine, 100 U/ml penicillin, 100 μg/ml streptomycin and 2 mm HEPES, at 37 °C with 5% CO2, in the Chemical, Biological and Agricultural Pluridisciplinary Research Center, State University of Campinas-UNICAMP, Campinas, Brazil. Sarcoma 180 tumour cells were maintained in the peritoneal cavity of Swiss mice in the Dr. Thomas George Bioterium (Research Institute in Drugs and Medicines/Federal University of Paraíba, Brazil).
Animals
For in vivo assays, adult female and male Swiss albino mice (28–32 g) were used. The animals were obtained from the Dr. Thomas George Bioterium and randomly housed in rodent cages in a controlled environment (12 h/12 h light-dark cycle, 24 ± 1 °C, 55% relative humidity) with free access to food and water. Animals were used in groups of 6. Measures were taken to minimize pain, stress and any suffering in accordance with ethical guidelines for animal use. The Animal Studies Committee of the Federal University of Paraíba had approved the experimental protocol (CEUA/UFPB number 0706/11).
Haemolysis assay
The haemolytic activity of EOX was tested using mouse erythrocytes according to Kang et al. (Citation2009), with some modifications. Briefly, fresh blood samples were collected with a heparinized capillary to prevent blood coagulation. Erythrocytes were suspended in phosphate buffered saline (PBS) to make a 1% (v/v) solution for the haemolysis assay. Mouse red blood cell suspension was incubated with various concentrations (0–750 μg/ml) of EOX dissolved in DMSO (5%, v/v, in PBS) in plates on a shaker for 60 min and then centrifuged. The absorbance of the supernatants was read at 540 nm using a UV-Vis spectrophotometer (UV-1650PC Shimadzu) to measure the extent of red blood cell (RBC) lysis, and the concentration producing 50% haemolysis (HC50) was determined. Positive controls (100% haemolysis) and negative controls (0% haemolysis) were also determined by incubating erythrocytes with 1% Triton X-100 in PBS and 5% DMSO in PBS, respectively. The haemolysis assay was performed in quadruplicate and repeated three times.
In vitro anticancer activity
The SRB assay was performed as described by Monks et al. (Citation1991). This assay relies on the ability of SRB to bind to protein components of cells that have been fixed to tissue-culture plates by TCA. SRB is a bright-pink aminoxanthene dye with two sulphonic groups that bind to basic amino acid residues under acidic conditions and dissociate under basic conditions.
Stock cultures were grown in 5 ml of RPMI-1640 supplemented with 5% FBS. Penicillin:streptomycin mixture of 1000 U/ml:1000 μg/ml was added to RPMI-1640 (1 ml/l) for experimental cultures. Cells in 96-well plates (100 μl cells/well) were exposed to different concentrations of EOX (0.25, 2.5, 25 and 250 μg/ml) in DMSO/RPMI/5% FBS at 37 °C at 5% CO2, for 48 h. Final DMSO concentration did not affect cell viability. Cells were then fixed with TCA solution (50%, v/v), and cell proliferation was determined by spectrophotometric quantification (540 nm) of cellular protein content. DOX (0.025–25 μg/ml) was used as the positive control. Three measurements were obtained: at the beginning of incubation (T0) and 48 h post-incubation for compound-free (C) and exposed (T) cells. The choice of 48 h incubation was based on the NCI60 protocol, proposed by NCI/USA for antiproliferative activity screening. Cell proliferation was determined according to the equation: cell proliferation =100 × [(T − T0)/C − T0]. The cytostatic effect was observed when T0 ≤ T < C, while cytocidal effect occurred when T < T0. The experiments were done in triplicate to calculate the total growth inhibition (TGI) (concentration that produces TGI).
Acute preclinical toxicity study
The evaluation of acute preclinical toxicity of EOX was performed according to the guide for the conduct of non-clinical toxicology studies and safety pharmacology necessary for the development of drugs, published by the Brazilian Health Surveillance Agency (ANVISA Citation2013), with some modifications. Mice (six males and six females) were given doses of 250, 375 and 500 mg/kg EOX (i.p.), and the control group received vehicle alone (5% Tween-80 in saline). For detection of toxic signs suggestive of activity on the central nervous system (CNS) or autonomic nervous system (ANS) of a general nature, the animals were closely observed at the following times: 0, 15, 30 and 60 min, 4 h, and daily for 14 d. The animals were weight at the beginning and at the end of treatment, and consumption of water and feed was determined daily. At the end of the observation period, all surviving animals were euthanized and necropsied. The liver, kidneys, heart, thymus and spleen were removed and weighed. Organ index was determined according to the formula: Index = weight of the organ (mg)/weight of animal (g).
Genotoxicity
For the micronucleus assay, groups of six male Swiss mice were treated intraperitoneally with different doses of EOX at 88, 176 and 281 mg/kg (25, 50 and 80% of the LD50 value, respectively) (Ribeiro Citation2003). A group treated with a standard drug (cyclophosphamide, 50 mg/kg, i.p.), and a control group (saline containing 5% Tween-80) were included. After 24 h, the animals were anesthetized with sodium thiopental (40 mg/kg), and peripheral blood samples were collected from the retro-orbital plexus for blood smears. Three blood smears were prepared for each animal, and a minimum of 2000 erythrocytes were counted to determine the frequency of micronucleated erythrocytes (OECD Citation1997).
In vivo anticancer activity
Eight-day-old sarcoma 180 ascites cells (0.2 ml – 25 × 106 cells/ml) were implanted subcutaneously into the left subaxillary region of female mice (n = six/group) (Bezerra et al. Citation2008). One day after inoculation, EOX (50 or 100 mg/kg) was dissolved in 5% (v/v) Tween-80 and administered intraperitoneally for 7 d to mice transplanted with sarcoma 180 tumour. 5-FU (25 mg/kg) was used as the standard drug. The healthy group (healthy mice) and tumour control group (mice bearing sarcoma 180) were inoculated with 5% Tween-80 in 0.9% (w/v) NaCl. On the eighth day, peripheral blood samples from all mice were collected from the retro-orbital plexus under light sodium thiopental anaesthesia (40 mg/kg).
The animals were then sacrificed by cervical dislocation. The tumours were excised and weighed, and then fixed in 10% formaldehyde and submitted to histopathological analysis. The tumour growth inhibition in per cent was calculated by the following formula: Inhibition (%) = [(A − B)/A] × 100, where A is the average of the tumour weights of the tumour control group, and B is that of the treated group.
Toxicity in transplanted mice
Body weights were recorded at the beginning and end of treatment, while consumption of water and feed was evaluated daily for the 7 d of treatment. The liver, spleen, thymus and kidneys were excised and weighed for determination of their organ index. For biochemical analysis, blood samples from the animals were centrifuged, and the levels of urea, creatinine and alanine aminotransferase (ALT) and aspartate aminotransferase (AST) activities were determined. For haematological analysis, heparinized whole blood was used, and the following haematological parameters were determined: haemoglobin (Hb) level, RBC count, haematocrit (Hct) and the red cell indices mean corpuscular volume (MCV), mean corpuscular haemoglobin (MCH) and mean corpuscular haemoglobin concentration (MCHC), as well as total and differential leukocyte counts. An automatic biochemical analyzer (COBAS Mira Plus, Roche Diagnostic Systems) and an automatic haematological analyzer (Animal Blood Counter – ABC Vet, Horiba) were used. After weight determination and fixation in 10% (v/v) formaldehyde, livers and kidneys were examined for size, colour changes and haemorrhage. Portions of the livers and kidneys were cut into small pieces and then into sections of 5 μm thickness, which was stained with haematoxylin-eosin and Masson’s trichrome for the liver.
Statistical analysis
Data are presented as mean ± SEM. The HC50 value and 95% confidence interval (95% CI) were obtained by nonlinear regression. From the concentration-response curve for each tumour cell line, TGI was determined by non-linear regression analysis using the software ORIGIN 8.0 (OriginLab Corporation, Northampton, MA). The differences between experimental groups were compared by analysis of variance (ANOVA), followed by Tukey’s test (p < .05).
Results
Identification of volatile compounds in EOX
EOX was obtained by hydrodistillation, with a yield of 0.03% in relation to the weight of fresh material used. The GC-MS analysis identified nine components, corresponding to 100% of the total oil, with a mixture of monoterpenes (66.6%), sesquiterpenes (22.2%) and diterpenes (11.2%). α-Pinene (34.57%) and limonene (31.75%) were the major constituents. The other components are listed in . From the external calibration, an average of the three curves was obtained. The equations from linear regressions obtained and R2 were y = 155670x − 2E + 07 and 0.9941 for α-pinene and y = 179137x − 2E + 07 and 0.9919 for limonene. The concentrations of α-pinene and limonene in the essential oil were 148.24 ppm and 111.86 ppm, respectively.
Table 1. Chemical composition of EOX.
Haemolysis assay
EOX exhibited a concentration-dependent haemolytic effect (). The mean HC50 was 293.6 (281.6–306.1 μg/ml).
In vitro anticancer activity
The in vitro antitumor activity of EOX (0.25–250 μg/ml) was investigated in nine human tumour cell lines using the SRB assay. EOX showed selectivity in its antiproliferative action against K562 and NCI/ADR-RES cells, with TGI values of 1.8 and 45.4 μg/ml, respectively ().
Table 2. Antiproliferative activity of EOX and doxorubicin against human cancer cell linesTable Footnotea.
Acute preclinical toxicity study
EOX caused animal death in a dose-dependent manner, with an LD50 of about 351.09 mg/kg. Some characteristic effects of CNS changes were observed, that is, stimulatory effects in the first minute after oil administration, but followed by depressant effects ().
Table 3. Behavioural effects of acute intraperitoneal treatment of EOX in mice.
Effects on the ANS were also observed, including increased urination, and particularly heavy breathing (Almeida et al. Citation1999). The effects were more exacerbated at the higher doses in both males and females (). There was a reduction in consumption of water and feed, which in turn led to a reduction in body weight in the study animals ().
Table 4. Effect of intraperitoneal administration of EOX (250, 375 e 500 mg/kg) on feed and water consumption and body weight gain in mice (14 d).
Genotoxicity
EOX was evaluated for possible in vivo genotoxic effects using the micronucleus assay. The results are presented in . EOX did not induce an increase in the number of micronucleated erythrocytes in peripheral blood.
Table 5. Number of micronucleated erythrocytes in peripheral blood of mice treated with single doses of EOX and cyclophosphamide (n = 6).
Table 6. Feed and water consumption and body weight gain of animals (n = 6) subjected to different treatments (7 d).
Table 7. Effects of 5-FU and EOX on the mice organ indices (n = 6) subjected to different treatments (7 d).
In vivo anticancer activity
In vivo antitumour activity was evaluated in mice transplanted with sarcoma 180 and treated i.p. with EOX (50 and 100 mg/kg) for 7 d. A significant reduction in tumour weight was observed in both groups, in comparison to the tumour control group.
Eight days after implantation, mean tumour weight was 2.49 ± 0.10 g in the tumour control group, and 1.52 ± 0.13 and 1.13 ± 0.12 g in EOX-treated groups for 50 and 100 mg/kg, respectively. In mice treated with 5-FU (25 mg/kg), tumour weight was also significantly reduced (0.74 ± 0.05 g). Inhibition was 38.67, 54.32 and 70.16% for treatment with 50 and 100 mg/kg EOX, and 5-FU, respectively ().
Figure 2. Effect of EOX and 5-FU on tumour weight and inhibition of tumour growth in mice transplanted with sarcoma 180. Data are expressed as mean ± SEM of six animals and were analyzed by ANOVA followed by Tukey’s test. ap < .05 compared to tumour control. bp < .05 compared to EOX (50 mg/kg). cp < .05 compared to EOX (100 mg/kg).
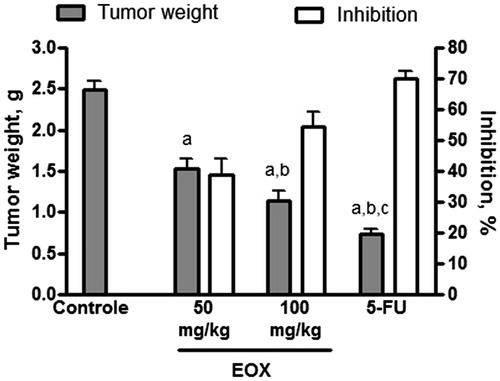
In the histopathological analysis of tumours, coagulative tumour necrosis areas were observed with quantitative variations between groups, asymmetrical mitosis, as well as local aggressive behaviour, evidenced by the invasion of surrounding fat tissue and skeletal muscle (). The same was true for the nerve fibres, while the perineurium showed no invasion. The architectural and cytological aspects of tumour in the experimental groups were similar to those observed in the tumour control group. In relation to the different tumour characteristics in the experimental groups, extensive areas of coagulative necrosis were found with 50 and 100 mg/kg EOX, corresponding respectively to 30% and 40% () of the neoplastic growth. The tumours of the animals treated with 5-FU (25 mg/kg), in turn, showed areas of necrosis corresponding to about 60% of the neoplastic growth ().
Figure 3. Histopathology of tumours of different experimental groups. (A) Tumour control: asymmetric mitoses. (B) 5-FU (25 mg/kg): coagulative necrosis areas. (C) EOX (50 mg/kg): invasion of adipose tissue (arrows). (D) EOX (50 mg/kg): invasion of skeletal muscle (arrows). (E) EOX (100 mg/kg): tumour growth advancing neural filament, without nerve infiltration (arrows). (F) EOX (100 mg/kg): coagulative necrosis areas (arrows). Haematoxylin-eosin (A, C, D, F) and Masson’s trichrome (B, E).
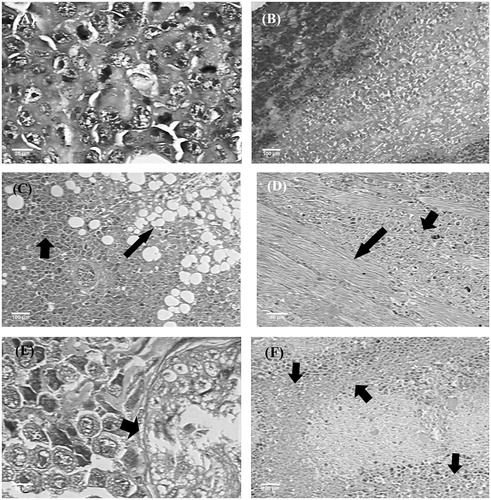
Toxicity in transplanted mice
In the toxicity study in transplanted mice, we observed that tumour implantation itself caused a reduction in feed intake in all the transplanted groups compared to the healthy group. Only treatment with 100 mg/kg EOX reduced food intake compared to the control group tumour. As a result, there was a decrease in the weights of these animals ().
To evaluate possible the toxic effects of EOX on internal organs, the animals’ liver, kidneys, spleen and thymus were excised, weighed and analyzed macroscopically (). Necrosis or haemorrhage was not observed with either dose of EOX used, nor in the group treated with 5-FU.
The toxicological study of EOX also included the evaluation of biochemical and haematological parameters. EOX treatment did not induce changes in the biochemical parameters evaluated ().
Table 8. Effects of 5-FU and EOX on biochemical and haematological parameters of peripheral blood of mice (n = 6) subjected to different treatments (7 d).
Minor changes were observed in haematological parameters. There was a significant increase in RBC count and a decrease in MCH as well in animals treated with 100 mg/kg EOX compared to the control group ().
5-FU produced marked leukopenia with lymphocytosis and neutropenia, as well as a reduction in the monocyte count, an already known side effect of this anticancer drug () (Pita et al. Citation2012). In contrast, treatment with 50 mg/kg EOX restored normal leukocyte count to levels obtained for the healthy group.
For a more detailed evaluation of EOX toxicity on the organs of the treated animals, histological analyses of the kidneys and liver were carried out. The kidneys from all experimental groups showed a normal histological appearance (data not shown).
In animals treated with 5-FU (25 mg/kg) and EOX (50 and 100 mg/kg), there was discrete hepatocellular lipid overload (microvesicular and macrovesicular steatosis) with the azonal (random) arrangement (). The sinusoidal macrophages (Kupffer cells) had become hypertrophic (). Foci of lymphohistiocytic infiltration were identified in some portal spaces (). This portal abnormality, although equally focal, was more pronounced in animals treated with 100 mg/kg EOX. Regarding the cytological aspects, hepatocytes were normal and isomorphic, showing some isolated mitosis. Small and sparse foci of hepatocytolysis accompanied by a discreet lymphohistiocytic inflammatory infiltrate within the lobule, in zones I, II and III, were present. These hepatocytolytic and inflammatory lesions, though sparse, were observed in the animals treated with the higher dose of EOX. Periportal and periseptal activities were absent. The lobular reticulin framework, in turn, was found to be smooth with some disarray in the larger foci of hepatocytolysis, associated with 100 mg/kg EOX treatment ().
Figure 4. Histopathology of livers of different experimental groups. (A) Tumour control: normal portal triad, liver beams radially distributed from vein centre lobular, and presence of hepatic sinusoids. (B) 5-FU (25 mg/kg): microvesicular steatosis (arrows). (C) EOX (50 mg/kg): lobular architecture preserved, especially the zonal distribution of hepatocytes (zones I, II and III). Presence of mild steatosis. (D) EOX (50 mg/kg): Kupffer cell intrasinusoidal hypertrophy. (E) EOX (50 mg/kg): discreet inflammatory infiltrate on space port. (F) EOX (100 mg/kg): macrovesicular steatosis (arrow), the presence of THV-terminal hepatic vein. (G) EOX (100 mg/kg): necroinflammatory focus (short arrow) bordering dividing hepatocyte (thick arrow). (H) EOX (100 mg/kg): portal inflammatory infiltrate. PVB, portal vein branch; THV, terminal hepatic vein; BD, bile duct. Haematoxylin-eosin (A, B, C, E, F) and Masson’s trichrome (D, G, H).
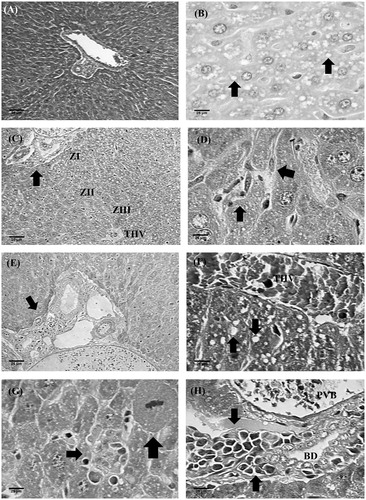
Discussion
Although the antitumour activity of essential oils of the genus Xylopia is described in the literature, the essential oil of the fruit of X. langsdorffiana had not been studied until now, both in relation to its antitumour activity and toxicity.
The chemical composition described here for EOX is compatible with literature data for volatile constituents of Xylopia species (Maia et al. Citation2005) and also with the chemical composition of the volatiles of their leaves, which characteristically contain monoterpenes and sesquiterpenes (Tavares et al. Citation2007). Spathulenol was also detected, which is considered a marker of essential oils of the genus Xylopia (Maia et al. Citation2005). The presence of the diterpene sclarene in the essential oil corroborates the biogenesis of the species under study since ent-labdane diterpenes have been isolated as common components of its fruits (Santos et al. Citation2013).
Anaemia incidence, the commonest haematological manifestation of cancer, afflicting 40–64% of patients treated for malignancies, increases with the administration of chemotherapy/radiotherapy. It reduces the quality of life and shortens survival in cancer patients (Gaspar et al. Citation2015). After receiving chemotherapy, the RBCs and haemoglobin may be decreased through their destruction and/or the inability of the bone marrow to make these cells. Some new antitumour drugs have shown the ability to cause the destruction of erythrocytes, including natural products (Zbidah et al. Citation2013).
Moreover, studies have documented that essential oils and their chemical constituents may have haemolytic effects (Mendanha et al. Citation2013). This activity of essential oils can be primarily related to their ability to interact with the cell membrane due to their lipophilic properties, which alters permeability, promoting haemolysis (Bakkali et al. Citation2008).
Therefore, in our work, we aimed to evaluate whether treatment with EOX produced haemolysis, which would be an undesirable effect in anticancer treatment. In comparison with the much lower concentrations that produced an antiproliferative effect in the SRB assay, higher concentrations were required to induce haemolysis, suggesting low toxicity to erythrocytes.
In the SRB assay, EOX showed in vitro antitumour activity especially against leukemic cells (K562) and ovary multidrug resistance phenotype cells (NCI-ADR/RES). The good activity against NCI-ADR/RES is a promising finding, since this cell line is multidrug resistant, which represents a tumour that is difficult to treat. EOX showed a much lower effect against the normal human cell line HaCat, suggesting low toxicity to non-tumour cells. Since cytotoxic substances are not selectively lethal to tumour cells and substantially affect normal cellular structures, there is a constant search for new chemotherapeutic drugs with higher antitumour activity and less toxic effects to normal cells.
Antitumour activity of limonene in animal tumour models and in vitro experiments has been reported (Yaghoub et al. Citation2006; Abirami & Nirmala Citation2014). These effects involve mainly induction of apoptosis, protein isoprenylation, inhibition of angiogenesis and immunomodulation (Sobral et al. Citation2014). In addition, limonene is considered to be a compound with low toxicity. Studies have determined that limonene does not pose a mutagenic, carcinogenic, or nephrotoxic risk to humans (Poon et al. Citation1996; Vigushin et al. Citation1998). Its metabolite, perillyl alcohol, also has potent in vitro and in vivo antitumour activity (Chen et al. Citation2015a). Induction of apoptosis, cell cycle arrest, inhibition of prenylation and isoprenylation of growth-regulatory proteins and antimetastatic activity are involved in its antitumour mechanisms of action (Sobral et al. Citation2014). Clinical trials using intranasal perillyl alcohol delivery showed this type of long-term, daily chemotherapy to be well tolerated and effective (Chen et al. Citation2015a). α-Pinene, the other major component of EOX, has shown antitumour activity by inducing apoptotic, antimetastatic, oxidative and immunomodulatory effects (Sobral et al. Citation2014), as well as G2/M cell cycle arrest (Chen et al. Citation2015b). These data suggest that the antitumour activity of EOX most likely involves the presence of limonene and α-pinene.
Cytotoxic effects have also been reported for the essential oils of Xylopia species (Ferraz et al. Citation2013; Quintans et al. Citation2013; Bakarnga-Via et al. Citation2014; Woguem et al. Citation2014); however, there is no report of activity against K562 cells. Furthermore, diterpenes obtained from X. langsdorffiana leaves have shown activity against K562 cells (Castello-Branco et al. Citation2009).
Preclinical toxicity studies are an important initial step for the safe use of drugs for both human health and the environment (Almeida et al. Citation1999). The effects of EOX in vivo were investigated by acute preclinical toxicology testing, with the aim of establishing safe doses to be used in pharmacological testing in vivo.
The reduction in consumption of water and feed, and in body weight observed in the study animals () suggest that EOX caused gastrointestinal toxicity. Many anticancer drugs cause gastrointestinal disorders, including anorexia, which is directly linked to malnutrition and weight loss (De Jonghe & Horn Citation2008). The psychopharmacological evaluation revealed late depressant effects, which can be explained by the formation of perillyl alcohol (CNS depressant agent), produced by limonene metabolism (d’Alessio et al. Citation2014). The effects on the ANS were suggestive of parasympathetic stimulation (increased urination), as well as its inhibition (heavy breathing) (Almeida et al. Citation1999). However, these effects disappeared after 4 h of treatment. In addition, all of these effects were observed with doses of EOX 5–10 times more than the minimum effective dose. The literature reports that, in general, if the lethal dose (LD50) of the test substance is three times more than the minimum effective dose, the substance is considered a good candidate for further studies (Auletta Citation1995; Amelo et al. Citation2014).
Genotoxicity tests are internationally recognized and well-defined by regulatory agencies as part of the battery of tests for the registration of new products entering the world market. The genotoxic effects of anticancer drugs on non-tumour cells are of special significance because of the possibility of inducing secondary tumours in patients undergoing treatment. Gene mutations act in carcinogenic stages, and assays that detect components permit the identification of substances with potential genotoxic risk to humans (Cavalcanti et al. Citation2010). The data showed that EOX does not have an in vivo genotoxic (clastogenic and/or aneugenic) effect, an important feature for therapeutic applicability.
In recent decades, several animal models have been developed to investigate the in vivo antitumour activity of new drugs. Herein, we used the sarcoma 180 murine tumour model to evaluate the activity of EOX. Our data point out a meaningful in vivo antitumour activity for EOX compared with other Xylopia species. The essential oil of X. frutescens has been shown to have in vivo antitumour activity against sarcoma 180 with tumour growth inhibition of 31.0 and 37.5% at 50 and 100 mg/kg, respectively. The inhibition of tumour growth produced by X. laevigata was reported to be 37.3 and 42.5%, at the above doses (Ferraz et al. Citation2013; Quintans et al. Citation2013). In this study, the histopathological findings support the idea that this focal neoplasm has local aggressiveness, with the absence of distant metastasis (Kurashige & Mitsuhashi Citation1982). Moreover, the effects observed for EOX were associated with extensive areas of coagulative necrosis in sarcoma 180 solid tumour.
In view of the various side effects displayed by anticancer agents due to toxicity to normal cells, we investigated the possible toxic effects of 7 d of treatment with EOX in transplanted mice. In this toxicity study using transplanted mice, the data agrees with the results of the acute toxicity test, suggesting gastrointestinal toxicity at 100 mg/kg. Nevertheless, these effects are observed in patients during treatments with most cancer drugs currently in use. However, the absence of changes in organ indices suggests low toxicity for EOX. 5-FU caused a decrease in thymus and spleen weight index. These organs have important functions in the immune system and are generally susceptible to the action of various drugs. Eichhorst et al. (Citation2001) showed that 5-FU causes apoptosis in different cell types including thymocytes and splenocytes, which is partially mediated by the CD95 system due to an up-regulation of CD95 and CD95L, resulting in thymus and spleen involution.
In regard to biochemical parameters, we used urea and creatinine to evaluate renal toxicity, and AST and ALT to evaluate liver toxicity. EOX did not induce changes in these parameters, suggesting that EOX did not cause renal or liver toxicity.
The changes in haematological parameters indicated normochromic microcytosis (increase in RBC count along with a decrease in MCH), which promoted a recovery of the normal haematological picture of the healthy group, demonstrating therefore a beneficial effect of this natural product, as it can be explained as an adaptive response of the animals to exposure to the essential oil over time. The decrease in lymphocyte percentage and increase in neutrophil percentage are characteristic changes in cases of tissue necrosis and the presence of tumours, and they were observed in all transplanted groups, regardless of treatment, except in the 5-FU group, as compared to the healthy group. In addition, the increase in total leukocytes in the tumour control animals compared to the healthy group is due to a leukaemoid reaction evoked by tumour presence, characterized by splenomegaly and granulocytosis, with the latter being interpreted as activation of host defences (Sato et al. Citation2005). Thus, the biochemical and haematological results showed weak clinical toxicity for EOX.
Kidney and liver are the major organs involved with drug excretion and metabolism, respectively. Histopathological analysis was performed to evaluate EOX toxicity to these organs. Corroborating the biochemical analysis data, it was observed that the kidneys from all experimental groups showed a normal histological appearance. In the liver, the changes observed in animals treated with EOX were characteristic of acute hepatitis, which is associated with foci of azonal steatosis, suggesting non-alcoholic steatohepatitis. However, a closer investigation of these effects is needed to reach a definitive conclusion. But it is important to note that these hepatotoxic effects may be reversible, as in the case of treatment with other natural products. This notion was reinforced in this study with EOX, by the absence of significant changes in the levels of liver function markers, AST and ALT.
Conclusion
EOX caused in vitro and in vivo growth inhibition of tumour cells, without major changes in the toxicity parameters evaluated. The results of this study provide essential information for further studies with EOX and the possible development of new chemotherapeutic agents.
Disclosure statement
This work was supported by the Brazilian agencies Coordenação de Aperfeiçoamento de Pessoal de Nível Superior/CAPES and Conselho Nacional de Desenvolvimento Científico e Tecnológico/CNPq. An American research scientist and author, Dr. Albert Leyva, helped with English editing of the manuscript. The authors report no declarations of interest.
References
- Abirami SKG, Nirmala P. 2014. A comparative – in vitro study of anticancer effect of Mentha piperita, Ocimum basilicum and Coleus aromaticus against human laryngeal epidermoid carcinoma (HEP-2) cell lines. J Med Plants Stud. 2:6–9.
- Adams RP. 1995. Identification of essential oil components by gas chromatography/mass spectroscopy. Carol Stream (IL): Allured Publishing Corporation.
- Agência Nacional de Vigilância Sanitária (ANVISA). 2013. Guia para a condução de estudos não clínicos de toxicologia e segurança farmacológica necessários ao desenvolvimento de medicamentos. Brasília (Brazil): Ministério da Saúde. Jan 1–48.
- Almeida RN, Falcão ACGM, Diniz RST, Quintans-Júnior LJ, Polari RM, Barbosa-Filho JM, Agra MF, Duarte JC, Ferreira CD, Antoniolli AR, et al. 1999. Metodologia para avaliação de plantas com atividade no sistema nervoso central e alguns dados experimentais. Braz J Pharmacogn. 80:72–76.
- Amelo W, Nagpal P, Makonnen E. 2014. Antiplasmodial activity of solvent fractions of methanolic root extract of Dodonaea angustifolia in Plasmodium berghei infected mice. BMC Complement Altern Med. 14:462–468.
- Auletta CS. 1995. Acute, sub chronic and chronic toxicology. London: CRC Press.
- Bakarnga-Via I, Hzounda JB, Fokou PVT, Tchokouaha LRY, Gary-Bobo M, Gallud A, Garcia M, Walbadet L, Secka Y, Dongmo PMJ, et al. 2014. Composition and cytotoxic activity of essential oils from Xylopia aethiopica (Dunal) A. Rich, Xylopia parviflora (A. Rich) Benth.) and Monodora myristica (Gaertn) growing in Chad and Cameroon. BMC Complement Altern Med. 14:125–132.
- Bakkali F, Averbeck S, Averbeck D, Idaomar M. 2008. Biological effects of essential oils – a review. Food Chem Toxicol. 46:446–475.
- Bhalla Y, Gupta VK, Jaitak V. 2013. Anticancer activity of essential oils: a review. J Sci Food Agric. 93:3643–3653.
- Bezerra DP, de Castro FO, Alves AP, Pessoa C, de Moraes MO, Silveira ER, Lima MA, Elmiro FJ, Alencar NM, Mesquita RO, et al. 2008. In vitro and in vivo antitumor effect of 5-FU combined with piplartine and piperine. J Appl Toxicol. 28:156–163.
- Castello-Branco MV, Anazetti MC, Silva MS, Tavares JF, Diniz MF, Frungillo L, Haun M, Melo PS. 2009. Diterpenes from Xylopia langsdorffiana inhibit cell growth and induce differentiation in human leukemia cells. Z Naturforsch C. 64:650–656.
- Castello-Branco MV, Tavares JF, Silva MS, Barbosa Filho JM, Anazetti MC, Frungillo L, Haun M, Diniz MFFM, Melo PS. 2011. Xylodiol from Xylopia langsdorffiana induces apoptosis in HL60 cells. Braz J Pharmacogn. 21:1035–1042.
- Castro MR, Victoria FN, Oliveira DH, Jacob RG, Savegnago L, Alves D. 2015. Essential oil of Psidium cattleianum leaves: antioxidant and antifungal activity. Pharm Biol. 53:242–250.
- Cavalcanti BC, Ferreira JR, Moura DJ, Rosa RM, Furtado GV, Burbano RR, Silveira ER, Lima MA, Camara CA, Saffi J, et al. 2010. Structure-mutagenicity relationship of kaurenoic acid from Xylopia sericeae (Annonaceae). Mutat Res. 701:153–163.
- Chen TC, Da Fonseca CO, Schönthal AH. 2015a. Preclinical development and clinical use of perillyl alcohol for chemoprevention and cancer therapy. Am J Cancer Res. 5:1580–1593.
- Chen W, Liu Y, Li M, Mao J, Zhang L, Huang R, Jin X, Ye L. 2015b. Anti-tumor effect of α-pinene on human hepatoma cell lines through inducing G2/M cell cycle arrest. J Pharmacol Sci. 3:332–338.
- De Jonghe BC, Horn CC. 2008. Chemotherapy-induced pica and anorexia are reduced by common hepatic branch vagotomy in the rat. Am J Physiol Regul Integr Comp Physiol. 294:756–765.
- d’Alessio PA, Bisson JF, Béné MC. 2014. Anti-stress effects of d-limonene and its metabolite perillyl alcohol. Rejuvenation Res. 17:145–149.
- Edris AE. 2007. Pharmaceutical and therapeutic potentials of essential oils and their individual volatile constituents: a review. Phytother Res. 21:308–323.
- Eichhorst ST, Müerköster S, Weigand MA, Krammer PH. 2001. The chemotherapeutic drug 5-fluorouracil induces apoptosis in mouse thymocytes in vivo via activation of the CD95(APO-1/Fas) system. Cancer Res. 61:243–248.
- Farag MA. 2009. Chemical composition and biological activities of Asimina triloba leaf essential oil. Pharm Biol. 47:982–986.
- Ferraz RP, Cardoso GM, da Silva TB, Fontes JE, Prata AP, Carvalho AA, Moraes MO, Pessoa C, Costaa EV, Bezerra DP. 2013. Antitumor properties of the leaf essential oil of Xylopia frutescens Aubl. (Annonaceae). Food Chem. 141:196–200.
- Gaspar BL, Sharma P, Das R. 2015. Anemia in malignancies: pathogenetic and diagnostic considerations. Hematology. 20:18–25.
- Hanahan D, Weinberg RA. 2011. Hallmarks of cancer: the next generation. Cell. 144:646–674.
- Kang C, Munawir AI, Cha M, Sohn ET, Lee H, Kim JS, Yoon WD, Lim D, Kim E. 2009. Cytotoxicity and hemolytic activity of jellyfish Nemopilema nomurai (scyphozoan: rhizostomeae) venom. Comp Biochem Physiol C Toxicol Pharmacol. 150:85–90.
- Kurashige S, Mitsuhashi S. 1982. Macrophage activities in sarcoma 180-bearing mice and EL4 bearing mice. Gan. 73:85–90.
- Maia JGS, Andrade EHA, Silva ACM, Oliveira J, Carreira LMM, Araújo JS. 2005. Leaf volatile oils from four Brazilian Xylopia species. Flavour Frag J. 20:474–477.
- Mendanha SA, Moura SS, Anjos JL, Valadares MC, Alonso A. 2013. Toxicity of terpenes on fibroblast cells compared to their hemolytic potential and increase in erythrocyte membrane fluidity. Toxicol in Vitro. 27:323–329.
- Mohsenzadeh F, Chehregani A, Amiri H. 2011. Chemical composition, antibacterial activity and cytotoxicity of essential oils of Tanacetum parthenium in different developmental stages. Pharm Biol. 49:920–926.
- Monks A, Scudiero D, Skehan P, Shoemaker R, Paull K, Vistica D, Hose C, Langley J, Cronise P, Vaigro-Wolff A, et al. 1991. Feasibility of a high-flux anticancer drug screen using a diverse panel of cultured human tumor cell lines. J Natl Cancer Inst. 83:757–766.
- Moreira IC, Roque NF, Vilegas W, Zalewski CA, Lago JH, Funasaki M. 2013. Genus Xylopia (Annonaceae): chemical and biological aspects. Chem Biodivers. 10:1921–1943.
- NIST/EPA/HIH Mass Spectral Library. Nist Mass Spectral Search Program (NIST 05, Version 2.0d). 2005. The NIST Mass Spectrometry Data Center: Gaithersburg, MD.
- Organisation for Economic Co-operation and Development (OECD). 1997. Mammalian Erythrocyte Micronucleus Test. Paris, France: Guideline for testing of chemicals n. 474. Jul 1–10.
- Pita JC, Xavier AL, de Sousa TK, Mangueira VM, Tavares JF, de Oliveira Júnior RJ, Veras RC, Pessoa HL, da Silva MS, Morelli S, et al. 2012. In vitro and in vivo antitumor effect of trachylobane-360, a diterpene from Xylopia langsdorffiana. Molecules. 17:9573–9589.
- Poon GK, Vigushin D, Griggs LJ, Rowlands MG, Coombes RC, Jarman M. 1996. Identification and characterization of limonene metabolites in patients withadvanced cancer by liquid chromatography/mass spectrometry. Drug Metab Dispos. 24:565–571.
- Quintans JS, Soares BM, Ferraz RP, Oliveira AC, da Silva TB, Menezes LR, Sampaio MF, Prata AP, Moraes MO, Pessoa C, et al. 2013. Chemical constituents and anticancer effects of the essential oil from leaves of Xylopia laevigata. Planta Med. 79:123–130.
- Ribeiro LR. 2003. Teste do micronúcleo em medula óssea de roedores in vivo. Canoas: Ulbra. Mutagênese Ambiental.
- Santos PF, Duarte MC, Bezerra DAC, Agra MF, Barbosa Filho JM, Silva MS, Tavares JF. 2013. Diterpenes from Xylopia langsdorffiana. Helv Chim Acta. 96:1085–1092.
- Sato DY, Wal R, de Oliveira CC, Cattaneo RI, Malvezzi M, Gabardo J, Buchi DF. 2005. Histopathological and immunophenotyping studies on normal and sarcoma 180-bearing mice treated with a complex homeopathic medication. Homeopathy. 94:26–32.
- Sobral MV, Xavier AL, Lima TC, de Sousa DP. 2014. Antitumor activity of monoterpenes found in essential oils. ScientificWorldJournal. 2014:953451. doi: 10.1155/2014/953451.
- Tavares JF, Silva MVB, Queiroga KF, Martins RM, Silva TMS, Camara CA, Agra MF, Barbosa-Filho JM, Silva MS, Marques MOM. 2007. Composition and molluscicidal properties of essential oils from leaves of Xylopia langsdorffiana A. St. Hil et Tul. (Annonaceae). J Essent Oil Res. 19:282–284.
- Tung YT, Yen PL, Lin CY, Chang ST. 2010. Anti-inflammatory activities of essential oils and their constituents from different provenances of indigenous cinnamon (Cinnamomum osmophloeum) leaves. Pharm Biol. 48:1130–1136.
- Van den Dool H, Kratz PD. 1963. A generalization of the retention index system including liner temperature programmed gas-liquid partition chromatography. J Chromatogr. 11:463–467.
- Vigushin DM, Poon GK, Boddy A, English J, Halbert GW, Pagonis C, Jarman M, Coombes RC. 1998. Phase I and pharmacokinetic study of D-limonene inpatients with advanced cancer. Cancer Research Campaign Phase I/II Clinical Trials Committee. Cancer Chemotherapy Pharmacol. 42:111–117.
- Wei A, Shibamoto T. 2007. Antioxidant activities and volatile constituents of various essential oils. J Agric Food Chem. 55:1737–1742.
- Woguem V, Fogang HP, Maggi F, Tapondjou LA, Womeni HM, Quassint L, Bramucci M, Vitali LA, Petrelli D, Lupidi G, et al. 2014. Volatile oil from striped African pepper (Xylopia parviflora, Annonaceae) possesses notable chemopreventive, anti-inflammatory and antimicrobial potential. Food Chem. 149:183–189.
- Yaghoub A, Mani A, Fatemeh M. 2006. New elaborated technique for isolation and purification of limonene from orange oil. Iran J Pharm Sci. 2:87–90.
- Zbidah M, Lupescu A, Herrmann T, Yang W, Foller M, Jilani K, Lang F. 2013. Effect of honokiol on erythrocytes. Toxicol in Vitro. 27:1737–1745.