Abstract
Context: Agastache mexicana ssp. mexicana (Kunth) Lint & Epling (Lamiaceae), popularly known as ‘toronjil morado’, is used in Mexican traditional medicine for the treatment of several diseases such as hypertension, anxiety and respiratory disorders.
Objective: This study investigates the relaxant action mechanism of A. mexicana ssp. mexicana essential oil (AMEO) in guinea-pig isolated trachea model.
Materials and method: AMEO was analyzed by GC/MS. The relaxant effect of AMEO (5–50 μg/mL) was tested in guinea-pig trachea pre-contracted with carbachol (3 × 10 − 6 M) or histamine (3 × 10 − 5 M) in the presence or absence of glibenclamide (10 − 5 M), propranolol (3 × 10 − 6 M) or 2′,5′-dideoxyadenosine (10 − 5 M). The antagonist effect of AMEO (10–300 μg/mL) against contractions elicited by carbachol (10 − 15–10 − 3 M), histamine (10 − 15–10 − 3 M) or calcium (10–300 μg/mL) was evaluated.
Results: Essential oil composition was estragole, d-limonene and linalyl anthranilate. AMEO relaxed the carbachol (EC50 = 18.25 ± 1.03 μg/mL) and histamine (EC50 = 13.3 ± 1.02 μg/mL)-induced contractions. The relaxant effect of AMEO was not modified by the presence of propranolol, glibenclamide or 2′,5′-dideoxyadenosine, suggesting that effect of AMEO is not related to β2-adrenergic receptors, ATP-sensitive potassium channels or adenylate cyclase activation. AMEO was more potent to antagonize histamine (pA2′ = −1.507 ± 0.122) than carbachol (pA2′ = −2.180 ± 0.357). Also, AMEO antagonized the calcium chloride-induced contractions.
Conclusion: The results suggest that relaxant effect of AMEO might be due to blockade of calcium influx in guinea-pig trachea smooth muscle. It is possible that estragole and d-limonene could contribute majority in the relaxant effect of AMEO.
Keywords:
Introduction
Agastache mexicana ssp. mexicana (Kunth) Lint & Epling (Lamiaceae) is native to the southwestern United States and México (Sanders Citation1987). In México, this species is popularly known as ‘toronjil morado’, and it is a commercially important species cultivated in various regions of México, such as the states of Hidalgo, México, Morelos, Puebla and Veracruz (Verano et al. Citation2013). Agastache mexicana has oval-lanceolate leaves, quadrangular, and purple coloration in basal and medium section of stem, and a sweet scent (Santillán-Ramírez et al. Citation2008). Various activities have been reported for this plant, including antinociceptive (Gonzalez-Trujano et al. Citation2012; Verano et al. Citation2013), anti-inflammatory (Adriana et al. Citation2012), antioxidant (Ibarra-Alvarado et al. Citation2010), antifungal (Juárez et al. Citation2015), spasmolytic (Gonzalez-Trujano et al. Citation2012), vasorelaxant (Ibarra-Alvarado et al. Citation2010), tracheal relaxant (Sánchez-Recillas et al. Citation2014), anxiolytic, sedative (Estrada-Reyes et al. Citation2004), and paradogically anxiogenic (Molina-Hernández et al. Citation2000). Tilianin, a glycoside flavone isolated from A. mexicana, has been reported as antihypertensive (Hernández-Abreu et al. Citation2013) and anxiolytic (González-Trujano et al. Citation2015).
Phytochemical studies of A. mexicana have revealed the presence of flavonoids, phenols, terpenoids, and alkanes (Gonzalez-Trujano et al. Citation2012; Estrada-Reyes et al. Citation2014). Methyl chavicol, linalool and d-limonene have been reported as major components of its essential oil (Estrada-Reyes et al. Citation2004). In spite of the wide use of this medicinal plant as traditional remedy for respiratory diseases, including asthma, only one study of relaxant effect of crude organic extracts has been reported in rat trachea (Sánchez-Recillas et al. Citation2014). The present study was undertaken to investigate the relaxant effect of essential oil of A. mexicana, using the guinea pig isolated trachea rings as experimental model.
Materials and methods
Drugs and reagents
Carbamoyl choline chloride (carbachol), acetylcholine chloride, histamine, propranolol hydrochloride and 2′,5′-dideoxyadenosine were purchased from Sigma Co. (St. Louis, MO). Glibenclamide was acquired from Helm de México S. A. (Ciudad de México, México). Glibenclamide, 2′,5′-dideoxyadenosine and essential oil were suspended in 0.5% Tween 80 is saline solution. All solutions were freshly prepared each time.
Experimental animals
All experiments were performed on adult male guinea pigs 350–450 g, Biosupply S. A. de C. V. (Puebla, México). The animals were maintained at constant room temperature (22 ± 2 °C) and submitted to a 12 h light/dark cycle with free access to food and water.
Procedures involving animals and their care were conducted in conformity with the Mexican Official Norm for Animal Care and Handling (NOM-062-ZOO-1999) adopted in our laboratory, and in compliance with international rules on care and use of laboratory animals. Furthermore, clearance for conducting the study was obtained from the Bioethics Committee (ENMH-CB-149-2015).
Plant material
Agastache mexicana was bought in Sonora Market, México in July 2012. The homogeneity and authenticity of the plant material was certificated by MSc Abigail Aguilar, botanist from the Herbarium of Centro Médico Nacional Siglo XXI. Samples were deposited in this Herbarium with the voucher number IMSSM16085.
Preparation of essential oil
Essential oils from A. mexicana were extracted from air-dried and ground fresh aerial parts (1467.1 g) by steam distillation for 3 h using a modified Clevenger-type apparatus. Yellowish essential oils were obtained (8.98 g, 0.68% yield) and stored in amber glass bottles at −4 °C until further analysis.
GC/MS analysis of essential oils
GC/MS analysis was performed on an Agilent 6890N GC (Agilent Technologies, Palo Alto, CA) coupled to a LECO 4D TOF (LECO Corporation, St. Joseph, MI) mass spectrometer, using a DB5 (5% phenyl methyl polysiloxane, 10 m × 0.18 mm i.d.; 0.18 μm film thickness, J & W Scientific, Folsom, CA), with the following temperature program: 3 min at 40 °C, subsequently 10 °C/min up to 280 °C at 5 °C/min, held for 10 min, and finally raised to 340 °C at 4 °C/min for 20 min isothermally. Injector and transfer line temperatures were 300 °C. Helium was the carrier gas, at a flow rate of 1.0 mL/min; injection volume: 1 μL of diluted oil in hexane (1:100); split mode (ratio: 1:75). The acquisition mass range: 45–600 m/z. All mass spectra were acquired in electron impact mode with an ionization voltage of 70 eV. A mixture of aliphatic hydrocarbons (C8-C24) (Sigma-Aldrich, St. Louis, MO) in hexane (J. T. Baker, Deventer, Netherlands) was directly injected into the GC injector under the above temperature program, in order to calculate the retention index (as the Kovats index) of each compound. Analysis was repeated three times. The data were analyzed by using MSD ChemStation software (Agilent, Version G1701DA D.01.00). The identification of the compounds found by gas chromatography-mass spectrometry was based on comparison of their Kovats index (RI) and mass spectra with those obtained from the NIST 08, Wiley 275 libraries and by comparison with data in the literature (Adams Citation1989).
Guinea-pig trachea preparation
Guinea pigs were euthanized by intraperitoneal injection of an overdose of sodium pentobarbital (150 mg/kg). The chest was opened to obtain the trachea, it was transferred to a dish containing warm Krebs–Henseleit solution (KHS), composition (mM): NaCl 118, KCl 4.7, NaH2PO4 1.2, MgSO4·7H2O 1.2, CaCl2·2H2O 2.5, NaHCO3 25, glucose 11.1. After removal of excess of connective tissue and fat, trachea was divided into eight small rings of about 2 mm of length containing from two to three cartilaginous segments. Each tracheal ring was hung between two Nichrome hooks inserted into the lumen, and placed in a 10 mL organ bath containing KHS. This solution was maintained at 37 ± 0.5 °C and bubbled constantly with 5% CO2–95% O2. Isometric tension was recorded through an eight-channel Biopac System polygraph MP100A-CE via a TSD 125C force transducer (Biopac System Inc., Goleta, CA). The data were digitalized and analyzed by specialized software for data acquisition (Acknowledge 3.9.0, Biopac System Inc., Goleta, CA). Tissues were placed under a resting tension of 1.5 g and allowed to stabilize for 60 min and then they were washed with fresh Krebs solution at 15 min intervals. The preparations were stimulated by acetylcholine 10 − 6 M at 30 min intervals and washed with fresh KHS at 15 min intervals before starting the experiments (Rodriguez-Ramos et al. Citation2011). After the stabilization period, trachea rings were contracted with carbachol (3 × 10 − 6 M) or histamine (3 × 10 − 5 M) and then cumulative concentrations of essential oil, diluted in Tween 80 0.5% (5–50 μg/mL), were added to the bath to yield the required tracheal relaxant effects and allowed to reach a steady state at each concentration. The vehicle was also added as control at the same volume (100 μL).
To evaluate the participation of ATP-sensitive potassium channel and β-adrenergic receptors, glibenclamide (10 − 5 M), propranolol (3 × 10 − 6 M), or their vehicles, were incubated for 5 min, after the carbachol (3 × 10 − 6 M) pre-contracted tissue reached a steady state, then cumulative concentrations of essential oil (5–50 μg/mL) or vehicle were added and isometric contractions were registered. Similarly, 2′,5′-dideoxyadenosine (adenylate cyclase inhibitor, 10 − 5 M) was added 5 min before the addition of cumulative concentrations of essential oil (5–50 μg/mL) or vehicle, after carbachol (3 × 10 − 6 M)-induced pre-contraction reached a steady state. The concentrations of glibenclamide, propranolol and 2′,5′-dideoxyadenosine were determined according previous works of our laboratory (Sanchez-Mendoza et al. Citation2008; Rodriguez-Ramos et al. Citation2011).
To determine the antagonistic effects of essential oil against the contractions elicited by carbachol or histamine, cumulative concentrations of carbachol (10 − 15–10 − 3 M) or histamine (10 − 15–10 − 3 M) were constructed in the absence or in the presence of different concentrations of essential oil as follows: after the tissues were incubated with different concentrations of essential oil (0, 10, 50, 100 and 300 μg/mL) or vehicle for 15 min, carbachol or histamine were cumulatively added and isometric contractions were registered. The antagonistic potential of essential oil were expressed as pA2′ values.
To determine the inhibition of contraction induced by calcium, after the stabilization period, the trachea rings were incubated in calcium-free KHS by 30 min. Then trachea rings were depolarized with potassium chloride (80 mM) and immediately washed. This procedure was repeated every 15 min during 1 h. At the least addition of potassium chloride, the trachea rings were not washed and cumulative concentrations of calcium (10–300 μg/mL) were added to contract the tissue in the presence or absence of essential oil (10, 50, 100 and 300 μg/mL).
Data analysis
The EC50 values were calculated by Sigmoid Emax model using GraphPad Prism version 6.00 for Windows (GraphPad Software, La Jolla, CA). All values are shown as mean ± SEM of at least six experiments. The experimental data were analyzed using one-way analysis of variance followed by Dunnett’s post hoc test. A value of p < 0.05 was considered significant. The antagonist effect of essential oil on cumulative concentration response curve of carbachol or histamine were expressed as the pA2′ value, calculated according to the equation pA2′ = pBx′ + Log (X-1), where pBx′ is the negative logarithm of the concentration (−Log Bx′) of antagonist (essential oil) and X is the ratio between maximal effect (Emax) of the agonist in the absence of antagonist and that in the presence of antagonist (essential oil). The pA2′ value is the abscissa to the origin of the graph Log (X-1) vs. pBx′, when X = 2 (Ko et al. Citation2002).
Results and discussion
This study clearly demonstrated that AMEO caused relaxation of contractions induced by carbachol (3 × 10 − 6 M) and histamine (3 × 10 − 5 M) in a concentration-dependent manner (); with EC50 values of 18.25 ± 1.03 and 13.30 ± 1.02 μg/mL, respectively. The previous incubation with AMEO (10–300 μg/mL) produced a decrease of maximal effect of the concentration-response curves of carbachol (10 − 15–10 − 3 M) and histamine (10 − 15–10 − 3 M) in a noncompetitive manner, with a pA2′ = −2.18 ± 0.36 (151.35 ± 2.27 μg/mL) and pA2′ = −1.51 ± 0.12 (32.13 ± 1.32 μg/mL) respectively (). In isotonic Ca2+-free high K+ (80 mM)-depolarized tracheas experiments, the situation was very different; the inhibition of Ca2+ (10 − 15–10 − 3 M)-induced contractions by AMEO (25–100 μg/mL) were reversed by an increase in extracellular Ca2 + concentrations (). The EC50 values of calcium chloride (EC50 = 5.29 ± 0.24 × 10 − 2 μg/mL) were significantly increased (F3,422 = 1039, p < 0.05) with AMEO at 25 μg/mL (EC50 = 8.62 ± 1.32 × 10 − 2 μg/mL), 50 μg/mL (EC50 = 7.92 ±2.82 × 10 − 2 μg/mL) and 100 μg/mL (EC50 = 19.87 ±3.5 ×10 − 2 μg/mL).
Figure 1. Concentration-relaxant response curve of AMEO in guinea-pig tracheal tissue after (A) carbachol (3 × 10 − 6 M) or (B) histamine (3 × 10 − 5 M)-induced contractions. Each point represents mean ± S.E.M. of at least six experiments.

Figure 2. Modified Schild plot for noncompetitive antagonism effect, according to the equation pA2′ = pBx′ + Log (X-1), where pBx′ is the negative logarithm of the concentration (-Log B′) of antagonist (AMEO) and X is the ratio between maximal effect (Emax) of (A) carbachol or (B) histamine in the absence of antagonist (AMEO) and that in the presence of antagonist (AMEO on guinea-pig tracheal tissue. The pA2′ value is the abscissa to the origin of the graph Log (X-1) vs pBx′, when X = 2. Each point represents mean ± S.E.M. of at least six experiments.
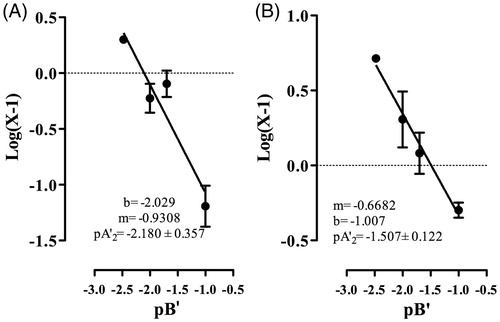
Figure 3. Calcium chloride-induced contractions on guinea-pig tracheal tissue in absence (•) or in presence of AMEO (▪ 25 μg/mL; ^ 50 μg/mL; ♦ 100 μg/mL). Each point represents mean ± S.E.M. of at least six experiments.
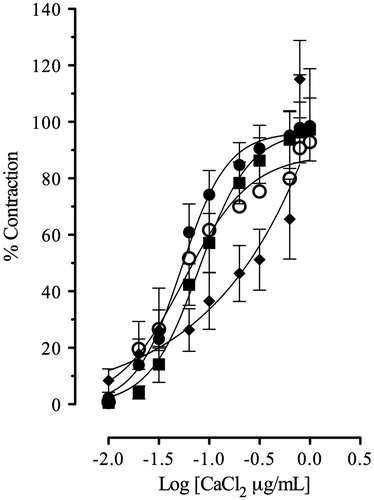
We evaluated the post-receptoral mechanism that can be involved in the relaxant effect of essential oils. The relaxant effect of AMEO in the carbachol (3 × 10 − 6 M)-induced contractions, was not affected by 2′,5′-dideoxyadenosine (3 × 10 − 6 M), an inhibitor of adenynyl cyclase (), suggesting that adenylyl cyclase is not involved in the relaxant mechanism of essential oils. In addition, openers of K + ATP channels, such as pinacidil, inhibit the acetylcholine release and decrease the contraction induced by acetylcholine and histamine in trachea (Sutovska et al. Citation2007). In this sense, glibenclamide (1 × 10 − 7 M), a K +ATP channels inhibitor, was tested on the relaxant effect of AMEO but the relaxant effect was not inhibited in the carbachol-induced pre-contractions (), denoting that K +ATP channels are not involved in the relaxant mechanism of AMEO. Also the relaxant effect of AMEO was not inhibited by propranolol (3 × 10 − 6 M; ); thus the relaxant effect on smooth muscle of trachea was not due to β2-adrenergic receptors activation. The contraction induced by carbachol and histamine is caused, at least in part, by releasing of Ca2+ from intracellular stores (Coelho-de-Souza et al. Citation1997). Due to histamine and acetylcholine act on Gq-coupled receptors in airway smooth muscle (Billington & Penn Citation2003; Perez-Zoghbi et al. Citation2009), the increased 1,4,5-inositol triphosphate (IP3) levels increase intracellular Ca2+ through release from internal stores and influx from membrane-bound channels to activate the contractile machinery in the cell through both Ca2+ and protein kinase C (PKC)-dependent mechanisms (Billington & Penn Citation2003). The blockade of Ca2+-induced contractions by AMEO together with the inhibition of carbachol- and histamine-induced contractions, suggest an intracellular site for the relaxant effect of AMEO; such as a blockade of Ca2+ release from storage site, a decrease in sensitivity to levels of intracellular Ca2+ of the contractile mechanisms and/or a direct interference with the contractile proteins. However, the full recovery of maximal Ca2+-induced contractions with high concentrations of calcium () makes it unlike that AMEO interfere directly with the contractile proteins. Therefore, the mechanism of this effect might be a blockade of cytoplasmic membrane Ca2+ channels, inhibiting the Ca2+ inflow (Coelho-de-Souza et al. Citation1997). Sánchez-Recillas et al. (Citation2014) reported the relaxant effect of hexane, dichloromethane and methanol crude extracts of A. mexicana, using the rat tracheal rings as model of study. Our study differ of that study considerably because we used essential oil of A. mexicana in guinea pig trachea rings, a model widely accepted as adequate, because rat trachea model have several shortcomings, such as the absence of response to beta adrenergic agonists. In addition Sánchez-Recillas et al. (Citation2014) reported only the relaxant effect of the crude extracts on precontracted rat trachea rings but did not study the action mechanisms.
Figure 4. Concentration-response curve of AMEO alone (^) and in the presence of (A) propranolol 3 × 10 − 6 M (▴), (B) 2′,5′-dideoxyadenosine 3 × 10 − 6 M (▪) or (C) glibenclamide 10 − 7 M (•). The graphs depict the logarithm of the concentration of AMEO (5–50 μg/mL) plotted against tension expressed as % of contraction elicited by carbachol 3 × 10 − 6 M in guinea pig-tracheal tissue. Each point represents the mean ± S.E.M of at least six experiments.

AMEO was characterized by the presence of estragole (80.28%), as the main compound of the essential oil, d-limonene (17.56%) and linalyl anthranilate (2.16%) (). The presence of estragole and d-limonene has been also reported in other plants of the genus Agastache (A. rugosa and A. foeniculum) (Wilson et al. Citation1992). The relaxant activity showed by the essential oil may be related to the presence of these compounds. Other research groups (Coelho-de-Souza et al. Citation1997; Franca et al. Citation2008) have reported that estragole has relaxant activity on intestinal smooth muscle. De Sousa et al. (Citation2008) also reported relaxant activity of d-limonene in guinea pig ileum. In addition, de Cassia da Silveira e Sa et al. (Citation2013) reported anti-asthmatic activity for estragole and prophylactic anti-inflammatory effect for d-limonene. It is possible that due to the high concentrations of estragole and d-limonene in AMEO and to previous reports on its activity, these compounds might be the components responsible for the relaxant effect of AMEO. However, we cannot discount the participation of other compounds in the relaxant effect of AMEO, or the existence of synergistic relaxant effect between them.
Table 1. Terpenoids identified in Agastache mexicana ssp. mexicana essential oil by GC/MS.
Conclusions
This study demonstrated that the Agastache mexicana ssp. mexicana essential oil produces a clear relaxant effect on guinea pig tracheal muscle. This essential oil has the ability to block the contractions induced by carbachol, histamine and calcium, and its action mechanism looks like involve the blockade of calcium influx. Possibly this effect is due majority to the presence of estragole and d-limonene, due that they are the major components in the essential oil of this plant. Finally, this study provides additional experimental support for the traditional use of Agastache mexicana ssp. mexicana in the treatment of asthma.
Disclosure statement
The authors report no conflicts of interest.
References
- Adams RP. 1989. Identification of essential oil components by ion trap mass spectrometry. San Diego (CA): Academic Press.
- Adriana G-R, María Eva G-T, Francisco P, López-Muñoz Francisco J. 2012. Anti-nociceptive and anti-inflammatory activities of the Agastache mexicana extracts by using several experimental models in rodents. J Ethnopharmacol. 142:700–705.
- Billington CK, Penn RB. 2003. Signaling and regulation of G protein-coupled receptors in airway smooth muscle. Respir Res. 4:2–23.
- Coelho-de-Souza AN, Barata EL, Magalhães PJC, Lima CC, Leal-Cardoso JH. 1997. Effects of the essential oil of Croton zehntneri, and its constituent estragole on intestinal smooth muscle. Phytother Res. 11:299–304.
- de Cassia da Silveira e Sa R, Andrade LN, de Sousa DP. 2013. A review on anti-inflammatory activity of monoterpenes. Molecules. 18:1227–1254.
- de Sousa DP, Junior GA, Andrade LN, Calasans FR, Nunes XP, Barbosa-Filho JM, Batista JS. 2008. Structure and spasmolytic activity relationships of monoterpene analogs found in many aromatic plants. Z Naturforsch C. 63:808–812.
- Estrada-Reyes R, Aguirre Hernandez E, Garcia-Argaez A, Soto Hernandez M, Linares E, Bye R, Heinze G, Martinez-Vazquez M. 2004. Comparative chemical composition of Agastache mexicana subsp. mexicana and A. mexicana subsp. xolocotziana. Biochem Syst Ecol. 32:685–694.
- Estrada-Reyes R, Ferreyra-Cruz OA, Dorantes-Barron AM, Moreno AJ, Lopez-Rubalcava C, Heinze G, Martinez-Vazquez M. 2014. Central nervous system effects and chemical composition of two subspecies of Agastache mexicana; an ethnomedicine of Mexico. J Ethnopharmacol. 153:98–110.
- Franca CS, Menezes FS, Costa LC, Niculau ES, Alves PB, Pinto JE, Marcal RM. 2008. Analgesic and antidiarrheal properties of Ocimum selloi essential oil in mice. Fitoterapia. 79:569–573.
- González-Trujano ME, Ponce-Muñoz H, Hidalgo-Figueroa S, Navarrete-Vázquez G, Estrada-Soto S. 2015. Depressant effects of Agastache mexicana methanol extract and one of major metabolites tilianin. Asian Pacific J Tropical Med. 8:185–190.
- Gonzalez-Trujano ME, Ventura-Martinez R, Chavez M, Diaz-Reval I, Pellicer F. 2012. Spasmolytic and antinociceptive activities of ursolic acid and acacetin identified in Agastache mexicana. Planta Med. 78:793–796.
- Hernández-Abreu O, Torres-Piedra M, García-Jiménez S, Ibarra-Barajas M, Villalobos-Molina R, Montes S, Rembao D, Estrada-Soto S. 2013. Dose-dependent antihypertensive determination and toxicological studies of tilianin isolated from Agastache mexicana. J Ethnopharmacol. 146:187–191.
- Ibarra-Alvarado C, Rojas A, Mendoza S, Bah M, Gutierrez DM, Hernandez-Sandoval L, Martinez M. 2010. Vasoactive and antioxidant activities of plants used in Mexican traditional medicine for the treatment of cardiovascular diseases. Pharm Biol. 48:732–739.
- Juárez ZN, Hernández LR, Bach H, Sánchez-Arreola E, Bach H. 2015. Antifungal activity of essential oils extracted from Agastache mexicana ssp. Xolocotziana and Porophyllum linaria against post-harvest pathogens. Ind Crops Prod. 74:178–182.
- Ko WC, Wang HL, Lei CB, Shih CH, Chung MI, Lin CN. 2002. Mechanisms of relaxant action of 3-O-methylquercetin in isolated guinea pig trachea. Planta Med. 68:30–35.
- Molina-Hernández M, Téllez-Alcántara P, Martínez E. 2000. Agastache mexicana may produce anxiogenic-like actions in the male rat. Phytomedicine. 7:199–203.
- Perez-Zoghbi JF, Karner C, Ito S, Shepherd M, Alrashdan Y, Sanderson MJ. 2009. Ion channel regulation of intracellular calcium and airway smooth muscle function. Pulm Pharmacol Ther. 22:388–397.
- Rodriguez-Ramos F, Gonzalez-Andrade M, Navarrete A. 2011. Gnaphaliin a and b relax smooth muscle of guinea-pig trachea and rat aorta via phosphodiesterase inhibition. J Pharm Pharmacol. 63:926–935.
- Sanchez-Mendoza ME, Castillo-Henkel C, Navarrete A. 2008. Relaxant action mechanism of berberine identified as the active principle of Argemone ochroleuca sweet in guinea-pig tracheal smooth muscle. J Pharm Pharmacol. 60:229–236.
- Sánchez-Recillas A, Mantecón-Reyes P, Castillo-España P, Villalobos-Molina R, Ibarra-Barajas M, Estrada-Soto S. 2014. Tracheal relaxation of five medicinal plants used in Mexico for the treatment of several diseases. Asian Pacific J Tropical Med. 7:179–183.
- Sanders RW. 1987. Taxonomy of Agastache section Brittonastrum (Lamiaceae Nepeteae). Syst Bot Monographs. 15:1–92.
- Santillán-Ramírez MA, López-Villafranco ME, Aguilar-Rodríguez S, Aguilar-Contreras A. 2008. Estudio etnobotánico, arquitectura foliar y anatomía vegetativa de Agastache mexicana ssp. Mexicana y A. mexicana ssp. xolocotziana. Revista Mexicana de Biodiversidad. 79:513–524.
- Sutovska M, Nosalova G, Franova S. 2007. The role of potassium ion channels in cough and other reflexes of the airways. J Physiol Pharmacol. 58:673–683.
- Verano J, Gonzalez-Trujano ME, Deciga-Campos M, Ventura-Martinez R, Pellicer F. 2013. Ursolic acid from Agastache mexicana aerial parts produces antinociceptive activity involving trpv1 receptors, cgmp and a serotonergic synergism. Pharmacol Biochem Behav. 110:255–264.
- Wilson LA, Senechal NP, Widrlechner MP. 1992. Headspace analysis of the volatile oils of agastache. J Agric Food Chem. 40:1362–1366.