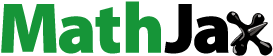
Abstract
Context: Control of African trypanosomiasis relies on chemotherapy, but the development of resistance and the problem of drug residues require research for alternatives. Triterpenes and phenolics, the major constituents of Pleurotus sajor-caju (Fr.) Singer (Pleurotaceae), are reported to be effective against trypanosomiasis.
Objective: Trypanocidal effect of whole Pleurotus sajor-caju aqueous extract was investigated in vivo against Trypanosoma congolense.
Materials and methods: Mice (25–32 g) were divided into seven groups of six animals. Mice in groups A–F received 2.5 × 104 trypanosomes, while group G was uninfected. Extracts (100–250 mg/kg) were administered intraperitoneally for 5 days to groups A–D while diminazine aceturate (group E) and normal saline (group F) served as positive and negative controls, respectively. Parasitemia, survival time, body weight and haematological parameters were monitored for 60 days post-treatment.
Results: Parasitemia decreased significantly (p < 0.01) post-treatment with 200 and 250 mg/kg of the extract and became undetectable by day 16 and 12 post-infection, respectively; the ED50 was 221.5 mg/kg. The packed cell volume (PCV) and the weight of mice treated with 250 mg/kg extract were 46.20 ± 2.6% and 32.05 ± 3.63 g, respectively, which is higher than the group treated with diminazine aceturate. The mean survival time of animals in groups D and E was >60 days, while that of group F was <4 days. Differential leucocyte count on day 68 post-infection in groups C, D and E were not significantly different.
Conclusion: Pleurotus sajor-caju therefore could be a potential source of new trypanocidal drugs.
Introduction
African trypanosomes are protozoan pathogens, causing human sleeping sickness and nagana, a related disease in cattle. The major pathogenic tsetse-transmitted trypanosome species are Trypanosoma congolense, Trypanosoma vivax and Trypanosoma brucei in cattle, sheep and goats, Trypanosoma simiae in pigs and Trypanosoma brucei in dogs. Within this region, ∼46–62 million of cattle and other livestock species are at risk of the disease (Swallow Citation2000). Trypanosomiasis is an important constraint to livestock development in sub-Saharan Africa. The causative agents of the Human African trypanosomiasis are Trypanosoma brucei gambiense and Trypanosoma brucei rhodesiense. The disease is found in many regions of the world, but mainly in sub-Saharan Africa between latitude 14°N and 29°S (World Health Organization [WHO] Citation1998). Approximately 9 million km2 of sub-Saharan Africa, representing about one-third of the total land, is invaded by tsetse flies (Mattioli et al. Citation2004); these flies are found in 37 countries, mostly in Africa. The economic losses attributed to African animal trypanosomiasis are due to decreased meat and milk production as a result of infertility, morbidity and mortality.
Although an increasing number of reports on drug resistance in animal trypanosomes in Africa have been published (Holmes et al. Citation2004), it is not clear whether this increase is due to a higher incidence of drug-resistant trypanosome strains or to a growing interest of the scientific community in this area of research. There is also the problem of the influx of fake drugs in Africa containing less active compound than required, leading to the mistaken impression that the strains are resistant, when in fact the drug is simply under-dosed. The estimation of its regional prevalence suggests 17 African countries (Delespaux et al. Citation2008), including Kenya (Murilla et al. Citation2002), Uganda, Tanzania (Eisler et al. Citation2000), Ethiopia (Tewelde et al. Citation2004), Zambia (Sinyangwe et al. Citation2004), Zimbabwe (Joshua et al. Citation1995), Cameroon (Mamoudou et al. Citation2008), Nigeria (Geerts et al. Citation2001), and Burkina Faso (McDermott et al. Citation2003), demonstrate area-wide resistance in at least one region of each country. The total value of the market for trypanocides for African farmers may exceed US$25 million, but this is considered insufficient by pharmaceutical companies to justify investment in the development of new drugs (FAO Citation2016). Therefore, the challenge remains to develop a cheap alternative chemotherapeutic control measure. Wild and cultivated mushrooms contain a diversity of biomolecules with nutritional (Kalac Citation2009) and/or medicinal properties (Poucheret et al. Citation2006). Due to these properties, mushrooms have been recognized as functional foods, and as a source for the development of medicines and nutraceuticals. Fruiting bodies, mycelia and spores accumulate a variety of bioactive metabolites with immunomodulatory, cardiovascular, liver anti-fibrotic, antiinflammatory, antidiabetic, antiviral, antioxidant, antitumor and antimicrobial properties (Zaidman et al. Citation2005; Poucheret et al. Citation2006; Beattie et al. Citation2010). Ergosterol peroxide (5α, 8α-epidioxy-22E-ergosta-6, 22-dien-3β-ol) isolated from Pleurotus ostreatus (Jacq.) P. Kumm. (Pleurotaceae) had an inhibitory concentration (IC50) of 6.74 μg/mL on Trypanosoma cruzi, but showed no lytic action on erythrocytes and no cytotoxic effect on mammalian cells at concentrations higher than 1600 μg/mL (Ramos-Ligonio et al. Citation2012). The phytochemical screening of cold and hot aqueous and silver nanoparticles extracts of Pleurotus sajor-caju (Fr.) Singer revealed bioactive compounds such as cucurbitacin, triterpenes, sterols, alkaloids, vitamins and minerals, and has also proved to contain antioxidant and therapeutic properties (Devi & Krishnakumari Citation2015). Phytochemical screening of aqueous and methanol extracts of Pleurotus sajor-caju revealed the presence of similar bioactive compounds in comparable quantity. The bioactive compounds include phenolics, flavonoids, saponins, alkaloids, glycosides, sterols, tannins, β-carotene, ascorbic acid, carbohydrates, proteins, amino acids and fatty acids (Johnsy & Kaviyarasan Citation2014). The methanol and aqueous extracts of the P. sajor-caju have been reported to possess antimicrobial and antioxidant activities (Pandiarajan et al. Citation2012; Johnsy & Kaviyarasan Citation2014). The triterpenes and phenolic content of Pleurotus sajorcaju is high and the information on the trypanocidal efficacy of these mushrooms is sparse. Thus, this study has provided evidence for the efficacy of Pleurotus sajor-caju and its effect on the hematological parameters in mice experimentally infected with Trypanosoma congolense.
Materials and methods
Extraction of mushroom
Fresh mushroom (Pleurotus sajor-caju) verified and cultivated in Forestry Research Institute of Nigeria, Ibadan, Nigeria, was collected April 2015 from the institute. The macro-fungus materials were air-dried at 45 °C in a hot air drier and grounded to a powder using a grinder. The extract was prepared by maceration with shaking for 2 h in water with a 10:1 solvent to dry weight ratio according to the method described by Eloff (Citation1998). The mixture was filtered using a previously weighed filter paper. A dark brown filtrate was obtained. The residue was dried, weighed again and the difference in weight gave the amount of powdered mushroom dissolved in water (w/v). Appropriate aliquots of the aqueous extract were taken and diluted to prepare graded doses of the extract for the assay.
Animals
The study was conducted with the permission of the University of Ibadan Animal Ethics Committee (UIACUREC/App/2015/019) and in line with the guidelines of the committee. BALB/c white male mice (25–32 g) were bought from the National Veterinary Research Institute Vom, Nigeria. They were allowed to acclimatize for 2 weeks at the experimental site. They were housed in cages under standard conditions of temperature (28–32 °C) and relative humidity of 70–80% with a light period of 12 h/day and were allowed to access water and food ad libitum.
Trypanosome stock
A susceptible strain of Trypanosoma congolense (Karu strain) was collected from the Nigerian Institute of Trypanosome and Onchorcerciasis Research (NITOR) Vom, Plateau State, Nigeria. It was kept in mice and transported to the animal house to be used as donor mice.
Toxicity test
Acute toxicity study was done according to OECD (Citation2001) guideline for testing of chemicals using mice. Twelve BALB/c albino mice were randomly divided into three groups (4 mice). After being fasted for 2 h, mice in the first group were given water; the second and third groups were given (intraperitoneally) 200 and 400 mg/kg of the aqueous extract of Pleurotus sajor-caju, respectively, and observed for any signs of toxicity in the first 4 h and subsequently daily for 10 days to assess safety of the extract. Animals were observed for gross changes, such as loss of appetite, hair erection, lacrimation, tremors, convulsions, salivation, diarrhea and mortality. Blood samples were collected to determine white blood cell count, red blood cell count, haemoglobin concentration (Hb) and packed cell volume (PCV) on day 10 after the administration of extract.
Determination of in vivo antitrypanosomal activity
Mice were divided into seven groups (A–G) with six mice (26–32 g) in each group. Mice in groups A–F were inoculated intraperitoneally with trypanosomes (2.5 × 104) while group G was not infected. The mice were screened for trypanosomes three days post infection using rapid matching counting method (Herbert & Lumsden Citation1976). When the parasitemia was 5 × 108 trypanosomes/mL on day 9 post infection, the mice in groups A–D were treated daily with 100, 150, 200 and 250 mg/kg aqueous extract of Pleurotus sajor-caju, respectively. Animals were treated for 5 days intraperitoneally. Group E served as the positive control and received diminazine aceturate (Diminaveto®, Arendonk, Belgium) at 7 mg/kg. Group F (infected) and G (uninfected) served as untreated control groups, and received normal saline. Mice were monitored daily, during and after treatment for parasitemia using rapid matching counting method (Herbert & Lumsden Citation1976) for 60 days after treatment to observe for possible relapse. The trypanocidal activity was evaluated by the mean survival time of treated mice for each dose. Treatment was considered to be successful when the mean survival time exceeded 60 days and the mice remained aparasitemic. Cure rates were calculated and expressed as percentages on day 60 post treatment. Blood samples for haematological analysis were obtained from the tail before treatment and day 60 post treatment. Haematological analysis was based on standard protocols (Jain Citation1986). White blood cell count (WBC) was done in a coulter counter (Jain Citation1986). Packed cell volume (PCV) was measured using micro haematocrit method (Schalm et al. Citation1975). Differential leucocyte counts were determined by microscopic examination of Giemsa stained blood smear (Jain Citation1986).
Statistical analysis
Determination of Effective Dose on 50% of the parasites (ED50) of a sigmoidal concentration response (variable slope) curve and comparison of haematological parameters by one-way ANOVA and Tukey’s multiple comparison test were performed using GraphPad Prism version 5 for Windows (GraphPad, San Diego, CA). The results were expressed as means ± S.E.M. significant difference between control group F and the treated groups using the Student’s t-test. The ED50 was determined by computing the concentration of extract that gave a response halfway between the minimum and maximum responses in a concentration–response sigmoid curve. The relation below gives the trypanocidal activity (TA):
Results
Yields of extract
The aqueous extract of Pleurotus sajor-caju gave a yield of 2.58 g (25.8% w/w).
Toxicity test
The preliminary acute toxicity test conducted revealed that there was no sign of toxicity (eye blinking, panting, lethargy, salivation or incoordination) or mortality in any of the treated groups of mice including those that received 400 mg/kg body weight which is more than the highest dose of Pleurotus sajor-caju aqueous extract use for the study. The haematological parameters observed was within the normal range in all the treated groups including those that received 400 mg/kg body weight extract ().
Table 1. Blood parameters of mice tested for Pleurotus sajor-caju toxicity.
Assessment of parasitemia and death
The statistical parameter of the curve fitting analysis and the best-fit ED50 values for the aqueous extract was 221.5 mg/kg (). The in vivo study showed significant efficacy of the extract applied for 5 days (day 9–13 post-infection). Parasitemia decreased after initiation of treatment with 200 and 250 mg/kg of the extract and became undetectable by day 16 and 12 post infection, respectively (). However, mice treated with 100 and 150 mg/kg died before day 60 post-treatment. The mice treated with 200 and 250 mg/kg of the extract remained aparasitemic until day 68 post infection. Animals in groups D and E exhibited the most potent trypanocidal activity with a mean survival of >60 days. The most effective dose treated with 250 mg/kg aqueous Pleurotus sajor-caju cured 100% of mice ().
Figure 1. In vivo concentration-response curve of aqueous extract of Pleurotus sajor-caju against blood stream Trypanosoma congolense using global sigmoidal model of curve fitting.

Figure 2. Parasitaemia in mice infected with Trypanosoma congolense post-treatment with Pleurotus sajor-caju bark extract and diminazine aceturate with time (days after the first day of treatment).

Table 2. Treatment of Trypanosoma congolense with Pleurotus sajor-caju and diminazine aceturate.
The packed cell volume (PCV) and the weight showed a similar course in mice treated with aqueous extract and diminazine aceturate (). After a drop to between 40% and 45% by day 9 post infection, the PCV recovered and reached normal values of 50–55% by day 68 post infection. The weight ranged between 25–32 g before treatment and 27–38 g 60 days after treatment. However, mice treated with 100 and 150 mg/kg exhibited signs of anorexia and weight loss, while the groups treated with 200 and 250 mg/kg had an appreciable weight gain as parasitemia decreases, suggesting relative effectiveness of Pleurotus sajor-caju. There was no significant difference (p > 0.05) in the PCV and weight of the groups treated with aqueous extract and the group treated with diminazine aceturate. However, the PCV and the weight of the group treated with 250 mg/kg extract was higher than the group treated with diminazine aceturate (). The differential white blood cell counts on day 68 post infection are shown in .
Discussion
The problem of trypanocidal resistance and an increasing concern over the presence of drug residues in animal products, when pure compounds are administered, has led to a resurgence of interest in the use of phytomedicines, in the form of extracts containing a mixture of compounds. This study was stimulated by a report of antimicrobial potential of edible mushrooms (Mendel Citation2015). The objective of the present study, therefore, was to evaluate the trypanocidal activity of the aqueous extract in mice models. Pleurotus sajor-caju extract was shown in vivo to exhibit trypanocidal activity in a concentration-dependent manner. The aqueous extract demonstrated an ED50 value of 221.5 mg/kg on day 13 post infection, which is the fourth day of treatment. The cure rate of animals treated with 250 mg/kg of the extract was 100% and the animals remained aparasitemic for more than 60 days post infection. This is remarkable because previous studies (Ekanem et al. Citation2008; Abu & Uchendu Citation2011; Tsegabirhan et al. Citation2014; Feyera et al. Citation2014) reported relapse when mice infected with trypanosomes are treated with either plant extracts or the commercial trypanocide (diminazine aceturate). For instance, Feyera et al. (Citation2014) reported that 400 mg/kg dichloromethane and 80% methanol extracts of Artemisia abyssinica Sch. Bip. ex A. Rich. (Asteraceae) did not completely eliminate T. congolense from the blood stream of infected mice, but considerably reduced the level of parasitemia. In a similar study with Basidiomycota, ergosterol peroxide extracted from Pleurotus ostreatus had an inhibitory concentration (IC50) of 6.74 μg/mL on T. cruzi, but showed no lytic action on erythrocytes and no cytotoxic effect on mammalian cells at concentrations higher than 1600 μg/mL (Ramos-Ligonio et al. Citation2012). The results of these studies reinforce the potential of Basidiomycota fungi as sources of bioactive natural products that may be developed into new therapeutic agents for neglected diseases, such as trypanosomiasis and leishmaniasis. Basidiomycota could also be a potential source of nematocide as shown by Stadler et al. (Citation1994) after screening for nematicidal activities in cultures of Basidiomycetes, and reported that cultures of Pleurotus pulmonarius and Hericium coralloides exhibited toxic effects towards the saprophytic nematode Caenorhabditis elegans.
The positive effect of trypanocides can further be assessed from weight measurements of the animals. Animals treated with the extract, on average, maintained their body weight at comparable levels to pretreatment values while those in the untreated infected group showed progressive reduction in body weights until death. Mice in groups A and B treated with 100 and 150 mg/kg, respectively, exhibited signs of weight loss, anorexia and death, while groups C and D treated with 200 and 250 mg/kg, respectively, had an increased weight gain as parasitemia decreases although the weight gain is not significantly different compared with the weight before treatment, suggesting relative effectiveness of Pleurotus sajor-caju. Peter et al. (Citation2009) also reported progressive weight increase with decrease parasitemia in mice infected with trypanosome. Tukey’s multiple comparison tests (post-ANOVA) revealed the relative parasitemia level at different dose and level of significance within the groups. It has also been shown that the level of anaemia gives a reliable indication of the disease status and productive performance of trypanosome-infected animals (Ekanem et al. Citation2006). The level of parasitemia was significantly (p < 0.05) higher in group A (100 mg/kg) compared to groups D and E treated with 200 and 250 mg/kg of the extract and diminazine aceturate, respectively. However, there was no significant difference (p > 0.05) in the level of parasitemia between the animals treated with 250 mg/kg of the extract and diminazine aceturate indicating that if the active compound in the extract is isolated and the dose reduces, their efficacy could be comparable to that of diminazine aceturate. There was no significant difference (p > 0.05) in the PCV and weight of the groups treated with 250 mg/kg extract and diminazine aceturate. However, the PCV and the weight of the groups treated with the extract were higher than the group treated with diminazine aceturate. The result of this study showed that P. sajor-caju extract could possess hematinic potential since they are able to control anaemia, especially at the later stages of the infection, by minimizing drops in PCV values. The differential white blood cell count on day 68 post infection in groups C, D and E were not significantly different. The leucocytosis observed could be a result of increase in the number or proportion of lymphocytes in the blood have been reported in trypanosomiasis and these conditions might be the result of wax and wear syndrome on the immune system of the animal, which is due to the changing variable surface glycoprotein of the trypanosomes (Abubakar et al. Citation2005) that demands the immune system to continuously produce antibodies and hence keep the lymphocytes level high. However, the groups treated with higher doses of the extract that significantly lowered parasitemia seemed to prevent lymphocytosis, which suggests that this phenomenon is a result of parasite burden. Hence, the relatively reduced percentage of lymphocytes post treatment suggests that the extracts contributed to the attenuation of the immunological reaction by reducing parasitemia.
The mice infected with T. brucei showed leucopenia, lymphocytosis, neutropenia and monocytosis in all the groups before treatment, except the uninfected control, before treatment commenced. The leucocyte count increased in the groups of mice treated with 200 and 250 mg/kg body weight. However, the leucocyte number was low in the group of mice treated with diminazine aceturate. Similarly, an increased neutrophil count was observed in the groups of mice treated with 200 and 250 mg/kg body weight, but the neutrophil number was lower in the group of mice treated with diminazine aceturate compared with the uninfected control. Pathogenic microorganisms are principally phagocytized by neutrophils, while lymphocytes are responsible for humoral and cellular immunity (Schalm et al. Citation1975; Baker & Silverton Citation1985). The decrease in differential leukocytes count following trypanosomes infection is consistent with reports that leucopenia characterized by neutropenia was seen in cats experimentally infected with T. brucei (Nfon et al. Citation2000). There was an increase in the number of lymphocytes (lymphocytosis) in the infected group before treatment compared to the uninfected control, until after treatment when the number of lymphocyte decreased (lymphopenia) except in the group of mice treated with diminazine aceturate with a higher number of lymphocyte. The decrease in lymphocyte numbers of leucocytes and neutrophils suggest that T. brucei caused marked antigenic stimulation leading to accelerated transformation of lymphocytes to plasma cells and transferred lymphocytes resulting to lymphopenia (Marrison et al. Citation1982; Anosa Citation1988b). Similarly, the severe fall in neutrophil number in the infected mice might have been caused by marked depression of precursor cells and marked phagocytosis of neutrophil precursor cell in the bone marrow (Anosa Citation1988b; Anosa et al. Citation1997) and spleen (Anosa & Kaneku Citation1984; Anosa Citation1988a). Eosinophilia was observed in the group of mice treated with 100 and 150 mg/kg body weight before treatment, however, mice treated with 200 and 250 mg/kg and diminazine aceturate showed eosinopenia. Anosa and Kaneku (Citation1984) also reported eosinophilia in T. brucei-infected deer mice. The decrease in the number of eosinophil is due to the therapeutic effect of the extract and diminazine aceturate, which reduced the number of T. brucei. The number of monocytes (monocytosis) in the infected group before treatment increased compared to the uninfected control, until after treatment when the number of monoocyte decreased. The monocytosis observed in mice infected with T. brucei before treatment may be due to increased demand for removal of particulate matter arising from severe pathology.
Treatment of parasitic diseases still represents a major challenge. Drug efficacy is mostly limited by the inability of the pharmaceutical to reach its target in a sufficient concentration and for a sufficient duration. In a study by Rosa et al. (Citation2009), Basidiomycota fungi representing 84 species and 17 families were screened in a bioassay of enzyme trypanothione reductase (TryR) from Trypanosoma cruzi, and amastigote forms of Leishmania amazonensis and reported that 34 of the extracts inhibited the activity of TryR and extracts from Gymnopilus areolatus Murrill (Cortinariaceae), Irpex lacteus (Fr.) Fr. (Meruliaceae), Lentinus strigosus Fr. (Polyporaceae), Nothopanus hygrophanus (Mont.) Singer ex Pegler (Marasmiaceae), Pleurotus flabellatus Sacc. (Pleurotaceae), and unidentified Basidiomycetes were toxic to Leishmania amazonensis Ross (Trypanosomatidae). Since Pleurotus spp extract used in their study demonstrated trypanocidal activity, this suggests that investigations into the trypanocidal efficacy of the extract of Pleurotus sajor-caju in vivo may also be of benefit.
Conclusions
Based on this in vivo study, Pleurotus sajor-caju was effective in reducing and eliminating Trypanosoma congolense. Therefore, the use of the extract may be a useful and effective product for the control of African human and animal trypanosomiasis. However, further spectroscopic studies on the active principles and the development of quality assurance protocols involving the use of reference substance of mushroom origin for this extract is warranted. Unambiguous structure elucidation of the active principles could provide leads for tyrpanocidal drug discovery and suitable bioactive marker compounds for standardization of the extract as a phytomedicine.
Disclosure statement
The authors report no conflicts of interest. The authors alone are responsible for the content and writing of this article.
References
- Abu AH, Uchendu CN. 2011. In vivo trypanocidal activity of hydroethanolic extract of Hymenocardia acida stem bark in rats. Vet World. 4:113–116.
- Abubakar A, Iliyasu B, Yusuf AB, Igweh AC, Onyekwelu NA, Shamaki BU, Afolayan DO, Ogbadoyi EO. 2005. Antitrypanosomal and hematological effects of selected Nigerian medicinal plants in Wistar rats. Biokemistri. 17:95–99.
- Anosa VO, Kaneku JJ. 1984. Pathogenesis of T. brucei infection in deer mice (P. maniculatus). Ultrastructural pathology of the spleen, liver, heart and kidney. Vet Pathol. 21:229–237.
- Anosa VO. 1988a. Hematological and biochemical changes in human and animal trypanosomiasis part 1. Rev Elev Med Vet Pays Trop. 41: 65–78.
- Anosa VO. 1988b. Hematological and biochemical changes in human and animal trypanosomiasis part 1. Rev Elev Med Vet Pays Trop. 41: 151–164.
- Anosa VO, Logan-Henfrey LL, Wells CW. 1997. The hematology of T. congolense infection in cattle II. Macrophage structure and function in the bone marrow of Boran cattle. Comp Haematol Int. 7:23–29.
- Baker FJ, Silverton RE. 1985. Introduction to medical laboratory technology, 6th ed. London: Macmillan Press. p. 320–328.
- Beattie KD, Rouf R, Gander L, May TW, Ratkowsky D, Donner CD, Gill M, Grice ID, Tiralongo E. 2010. Antibacterial metabolites from Australian macrofungi from the genus Cortinarius. Phytochemistry. 71:948–955.
- Delespaux V, Geysen D, Van den Bossche P, Geerts S. 2008. Molecular tools for the rapid detection of drug resistance in animal trypanosomes. Trends Parasitol. 24:236–242.
- Devi MR, Krishnakumari S. 2015. Qualitative screening of phytoconstituents of Pleurotus sajor caju (Fries singer) and comparison between hot and cold: aqueous and silver nanoparticles extracts. J Med Plants Stud. 3:172–176.
- Eisler MC, McDermott JJ, Mdachi R, Mbwambo H, Sinyangwe L, Machila N, Delespaux V, Geerts S ,Brandt J, Peregrine AS et al. 2000. Area-wide appraisal of drug resistance in trypanosomes infecting cattle in East and Southern Africa. ICPTV Newslett. 2:16–18.
- Ekanem JT, Kolawole OM, Abbah OC. 2008. Trypanocidal potential of methanolic extracts of Bridelia ferruginea Benth in Rattus novergicus. Afr J Biochem Res. 2:045–050.
- Ekanem JT, Majolagbe OR, Sulaiman FA, Muhammad NO. 2006. Effects of honey supplemented diet on the parasitemia and some enzymes of T. brucei-infected rats. Afr J Biotech. 5:1557–1561.
- Eloff JN. 1998. Which extractant should be used for the screening and isolation of antimicrobial components from plants?. J Ethnopharmacol. 60:1–8.
- Feyera T, Getachew T, Workineh S. 2014. Evaluation of in vivo antitrypanosomal activity of crude extracts of Artemisia abyssinica against a Trypanosoma congolense isolate. Comp Alt Med. 14:117–125.
- Food and Agriculture Organization (FAO). 2016. Agriculture and consumer protection department, Animal production and health. Available from: http://www.fao.org/ag/againfo/programmes/en/paat/par_control.html.
- Geerts S, Holmes PH, Diall O, Eisler MC. 2001. African bovine trypanosomiasis: the problem of drug resistance. Trends Parasitol. 17:25–28.
- Herbert WJ, Lumsden WH. 1976. Trypanosoma brucei: a rapid “matching” method for estimating the host's. Exp Parasitol. 40:427–431.
- Holmes PH. 2004. Current chemotherapy of animal trypanosomiasis. In: Maudlin I, Holmes PH, and Miles MA, editors. The trypanosomiasis: CABI Publishing, p. 431–444.
- Jain NC. 1986. Schalm's veterinary hematology. 4th ed. Philadelphia (PA): Lea and Febiger. p. 610–612.
- Johnsy G, Kaviyarasan V. 2014. Preliminary phytochemical screening, antimicrobial and antioxidant activity of methanol and water extracts of Lentinus sajor-caju. World J Pharm Pharm Sci. 3:1459–1472.
- Joshua RA, Obwolo MJ, Bwangamoi O, Mandebvu E. 1995. Resistance to diminazine aceturate by Trypanosoma congolense from cattle in the Zambezi Valley of Zimbabwe. Vet Parasitol. 60:1–6.
- Kalac P. 2009. Chemical composition and nutritional value of European species of wild growing mushrooms: a review. Food Chem. 113:9–16.
- Mamoudou A, Delespaux V, Chepnda V, Hachimou Z, Andrikaye JP, Zoli A, Geerts S. 2008. Assessment of the occurrence of trypanocidal drug resistance in trypanosomes of naturally infected cattle in the Adamaoua region of Cameroon using the standard mouse test and molecular tools. Acta Tropica. 106:115–118.
- Marrison WI, Murray M, Hindson CA. 1982. The response of the murine lymphoid system to a chronic infection with Trypanosoma congolense. II. The lymph nodes, thymus and liver . J Pathol. 138:273–288.
- Mattioli RC, Feldmann U, Hendrickx G, Wint W, Jannin J, Slingenbergh J. 2004. Tsetse and trypanosomiasis intervention policies supporting sustainable animal-agricultural development. J Food Agric Environ. 2:310–314.
- McDermott J, Woitag T, Sidibe I, Bauer B ,Diarra B, Ouédraogo D, Kamuanga M, Peregrine A, Eisler M, Zessin KH, et al. 2003. Field studies of drug-resistant cattle trypanosomes in Kénédougou Province, Burkina Faso. Acta Tropica. 86:93–103.
- Mendel F. 2015. Chemistry, nutrition, and health-promoting properties of Hericium erinaceus (Lion’s Mane) mushroom fruiting bodies and mycelia and their bioactive compounds. J Agric Food Chem. 63:7108–7123.
- Murilla GA, Peregrine AS, Ndungu JM, Holmes PH, Eisler MC. 2002. The effects of drug-sensitive and drug-resistant Trypanosoma congolense infections on the pharmacokinetics of homidium in Boran cattle. Acta Tropica. 81:185–195.
- Nfon CK, Oyewunmi OB, Nottidge HO, Taiwo VO. 2000. Experimental T. brucei and T. congolense infection in cats: clinicopathological study. Trop Vet. 18:220–227.
- Organization of Economic Co-operation and Development (OECD). 2001. The OECD guidelines for testing of chemical: 423 acute oral toxicity. France: OECD Publishing.
- Pandiarajan G, Govindaraj R, Mareeswaran J, Makesh Kumar B. 2012. Antibaterial activity and heavy metal accumulation of edible oyster mushroom (Pleurotus sajor-caju) grown on two substrates. Inter J Pharm Pharm Sci. 4:238–240.
- Peter O, Magiri E, Auma J, Magoma G, Imbuga M, Murilla G. 2009. Evaluation of in vivo antitrypanosomal activity of selected medicinal plant extracts. J Med Plant Res. 3:849–854.
- Poucheret P, Fons F, Rapior S. 2006. Biological and pharmacological activity of higher fungi: 20-Year retrospective analysis. Mycologie. 27:311–333.
- Ramos-Ligonio A, López-Monteon A, Trigos A. 2012. Trypanocidal activity of ergosterol peroxide from Pleurotus ostreatus. Phytother Res. 26:938–943.
- Rosa LH, Machado KM, Rabello AL, Souza-Fagundes EM, Correa-Oliveira R, Rosa CA, Zani CL. 2009. Cytotoxic, immunosuppressive, trypanocidal and antileishmanial activities of Basidiomycota fungi present in Atlantic Rainforest in Brazil. Int J Gen Mol Microbiol. 95:227–237.
- Schalm OW, Jain NC, Carroll EJ. 1975. Veterinary Hematology. 3rd ed. Philadelphia: Lea and Fabiger. p. 52–156.
- Sinyangwe L, Delespaux V, Brandt J, Geerts S, Mubanga J, Machila N, Holmes PH, Eisler MC. 2004. Trypanocidal drug resistance in Eastern province of Zambia. Vet Parasitol. 119:125–135.
- Stadler M, Mayer A, Anke H, Sterner O. 1994. Fatty acids and other compounds with nematicidal activity from cultures of Basidiomycetes. Planta Medica. 60:128–132.
- Swallow BM. 2000. Impacts of trypanosomosis on African agriculture. PAAT Technical Scientific Series 2, FAO.
- Tewelde T, Abebe G, Eisler MC, McDermott J, Greiner M, Afework Y, Kyule M, Münstermann S, Zessin KH, Clausen PH. 2004. Application of field methods to assess isometamidium resistance of trypanosomes in cattle in western Ethiopia. Acta Tropica. 90:163–170.
- Tsegabirhan K, Getachew T, Yacob HT, Giday M, Kebede N. 2014. Effect of crude extracts of Moringa stenopetala and Artemisia absinthium on parasitemia of mice infected with Trypanosoma congolense. Res Notes. 7:390.
- World Health Organization (WHO). 1998. Expert Committee on Control and surveillance of African Trypanosomiasis. WHO Technical Report Series No 881. Geneva: World Health Organization.
- Zaidman BZ, Yassin M, Mahajana J, Wasser SP. 2005. Medicinal mushroom modulators of molecular targets as cancer therapeutics. Appl Microbiol Biotechnol. 67:453–468.