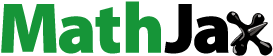
Abstract
Context: The defatted seeds of Camellia oleifera var. monosperma Hung T. Chang (Theaceae) are currently discarded without effective utilization. However, sapogenin has been isolated and shows antioxidative, anti-inflammatory and analgesic activities suggestive of its neuroprotective function.
Objective: In order to improve the activities of sapogenin, the nanoparticles of iron–sapogenin have been synthesized, and the neuroprotective effects are evaluated.
Materials and methods: Structural characters of the nanoparticles were analyzed, and the antioxidant effect was assessed by DPPH method, and the neuroprotective effect was evaluated by rotenone-induced neurodegeneration in Kunming mice injected subcutaneously into the back of neck with rotenone (50 mg/kg/day) for 6 weeks and then treated by tail intravenous injection with the iron–sapogenin at the dose of 25, 50 and 100 mg/kg for 7 days. Mice behaviour and neurotransmitters were tested.
Results: The product had an average size of 162 nm with spherical shape, and scavenged more than 90% DPPH radicals at 0.8 mg/mL concentration. It decreased behavioural disorder and malondialdehyde content in mice brain, and increased superoxide dismutase activity, tyrosine hydroxylase expression, dopamine and acetylcholine levels in brain in dose dependence, and their maximum changes were respectively up to 60.83%, 25.17%, 22.13%, 105.26%, 42.17% and 22.89% as compared to vehicle group. Iron–sapogenin nanoparticle shows significantly better effects than the sapogenin.
Discussion and conclusion: Iron–sapogenin alleviates neurodegeneration of mice injured by neurotoxicity of rotenone, it is a superior candidate of drugs for neuroprotection.
Introduction
Neurodegeneration is a late-onset, progressive cognition and motor disease marked by neuronal loss or death with uncertain mechanism (Jabir et al. Citation2014). Parkinson disease (PD) and Alzheimer’s disease (AD) are typical in neurodegeneration with pathological hallmarks of α-synuclein and β-amyloid in neurons of cerebral cortex, striatum, thalamus, hypothalamus, amygdale and basal forebrain (Bae et al. Citation2012; Balducci & Forloni Citation2014). A majority of studies explored the effect of oxidative stress that contributes to the cascade of events leading to neurodegeneration (Venkateshappa et al. Citation2012). Currently, there is no perfect treatment for those diseases, and traditional treatment strategy is L-dopa replacement therapy for PD (Fernandez Citation2015), and acetylcholinesterase inhibitor for AD (Kumar & Singh Citation2015). Although the clinical symptoms are mitigated, disease progression cannot be stopped (Ohno et al. Citation2015). Moreover, long-term chemical treatment leads to efficacy reduction and severe adverse effects, lowering the quality of patients’ lives (Duty Citation2010). In recent years, medication therapy has changed from direct chemical treatment to multilevel therapy, aiming at reliving the symptoms and impeding the progression of the disease at the same time (Venigalla et al. Citation2015). Natural medicines play an important role in prevention and therapy of neurodegeneration (Zhao Citation2009).
Camellia oleifera var. monosperma Hung T. Chang (Theaceae), an edible oil plant grown only in the mountain region of China inland and Taiwan, differs from tea plant (Wei et al. Citation2012). The defatted seeds of C. oleifera are usually discarded leading to great waste of natural resource and environmental pollution. Recent research discloses that the defatted seeds of C. oleifera contain many bioactive compounds such as saponins, flavonoids, polysaccharides, etc. (Luo et al. Citation2003). Saponin is one of the active ingredients in many herbs, and natural saponins have neuroprotective effects relevant to the suppression of oxidative stress, elimination of free radicals and inhibition of inflammation (Han et al. Citation2008; Wang et al. Citation2010; Ye et al. Citation2011). Our previous researches proved the antioxidative, anti-inflammatory and analgesic activities of the sapogenin isolated from the seeds of C. oleifera, disclosing its central roles (Ye et al. Citation2013a, Citation2013b, Citation2014), and will further evaluate its neuroprotective effect in this research.
Iron, an essential element for growth and breeding, is the component of haemoglobin and many enzymes such as oxidase, reductase, etc., which consist of important antioxidant system in vivo (Valerio Citation2007). Iron takes part in many brain physiological process and formation of nerve myelin sheath and lots of neurotransmitters (Lozoff et al. Citation2006). Organic iron have greater bioavailability than the inorganic (Ma et al. Citation2014), but accumulation of inorganic iron in brain is harmful and relevant to neurodegeneration (Bellingham et al. Citation2015).
We found that the sapogenin could make a stable complex with iron, and synthesized an organic iron compound (iron–sapogenin) with nanoparticle characters, which inspired us to investigate its central effects. In this article, we evaluate the antioxidant effect of iron–sapogenin nanoparticle in vitro and in vivo, and investigate its effect on neurotransmitters in central tissues in order to assess potential as a new drug for neuroprotection.
Materials and methods
Chemicals and reagents
Sapogenin (purity 98%) was isolated from the defatted seeds of C. oleifera, and prepared in our lab with structural identification (Ye et al. Citation2014). 2,2-Diphenyl-1-picrylhydrazyl (DPPH) was purchased from Wako Pure Chemical Industry, Ltd (Osaka, Japan). Rotenone for inducing neurotoxicity model in animal tests was purchased from Sigma-Aldrich Company (St. Louis, MO). Superoxidase dismutase (SOD) kit, malondialdehyde (MDA) kit, acetylcholine (Ach) kit, dopamine (DA) kit and tyrosine hydroxylase (TH) kit were purchased from Nanjing Jiancheng Bioengineering Institute (Nanjing, China). Vitamin C and other experimental reagents for the analysis were of analytical purity and bought from Qianhui Chemical Company (Guangzhou, China).
Animals
The experiments were carried out on male Kunming mice of weight 25 ± 3 g, supplied by Experimental Animal Center of South Medical University. The animals were housed under conditions of 25 ± 2 °C, 50 ± 10% humidity with a 12 h light/dark cycle. Food and water were accessible ad libitum. The experiments have been performed in accordance with the Chinese Guidelines for the use of laboratory animals, and received approval from the Animal Experimentation Ethic Committee of South China University of Technology. All efforts were made to minimize animal suffering and to reduce the number of animals used.
Synthesis of iron–sapogenin
Sapogenin (1.0 g) was dissolved in 30 mL ethanol, 1 mmol sodium carbonate powder was added under 60 °C for 1 h, then mixed with 0.5 mmol FeCl3•6 H2O ethanol solution under reflux at 70 °C. The solution pH was adjusted to 8–9 to precipitate target product by ethanol/ammonia (V/V = 1). After 8 h, the precipitation was collected by centrifugation (4000 rpm, 10 min). The precipitation was washed by 1 mol/L hydrochloric acid solution and ethanol alternately and dried in vacuum.
Structure identification
UV spectra analysis was carried out on UV-3010 ultraviolet spectrometer (Hitachi Company, Tokyo, Japan) scanning from 200 to 800 nm; IR spectra were measured on Nicolet 380 FI-IR spectrograph (Nicolet Apparatus Company, Waltham, MA) with KBr tablets from 4000 to 400 cm−1 with a resolution of 2 cm−1. Element analysis was determined on elemental analyzer Vario EL III (ELEMENTAR Company, Langenselbold, Germany).
Morphological observation
The morphology of sapogenin metal complex was observed under a JEM-2100F high resolution scanning electron microscope (SEM) (JEOL Company, Tokyo, Japan) at 200 kV. Particle size distribution and polydispersity index (PDI) of the products were determined by Nano-2S MDT-2 Malvern Particle Size Analyzer (Malvern Instruments Ltd, Malvern, UK). Before testing, the appropriate sample was dissolved in DMSO and dispersed in water with a refractive index of 1.33. Data were calculated as the average of five repetitions.
TG/DTG analysis
Thermogravimetric analysis (TG) was performed on Q6000-SDT Thermogravimetric Apparatus (TA Instrument Ltd, New Castle, DE). The nanoparticles (4 mg) were heated from 20 °C–800 °C under nitrogen flow at a rate of 20 °C/min. Differential thermal gravity (DTG) curve was plotted.
DPPH radical scavenging activity
The DPPH free radical scavenging activity was analyzed by the method as reported with some modifications (Gao et al. Citation2008). The product was dissolved in 10 mL of 95% ethanol, and diluted to 0.025, 0.05, 0.1, 0.2, 0.4 and 0.8 mg/mL. Each sample (2 mL) was mixed with DPPH (2 × 10−4 mol/L, 2 mL) in ethanol solution. The mixture was kept in the dark at room temperature for 30 min, and detected at 524 nm (absorbance Aa, ethanol as blank), the primary absorbance Ao was recorded when the extract was replaced by ethanol, and background absorbance Ab was recorded when ethanol took place DPPH. DPPH radical scavenging activity of the samples was calculated as follows:
Neurodegeneration induced by rotenone in mice
The experiment was performed according to the method illustrated by Murakami et al. (Citation2014). Mice were randomly divided into six groups of eight mice for each: normal group, vehicle group, four medicated groups including sapogenin, high, middle and low doses of iron–sapogenin groups. Vehicle and medicated groups were injected subcutaneously into the back of neck with rotenone (50 mg/kg/d) for 6 weeks. Mice appeared symptoms of tremor, epilepsy, depression and other symptoms suggesting successful model. Medicated groups of mice were tail intravenously injected with the sapogenin at 100, 25 (low dose), 50 (middle dose) and 100 mg/kg (high dose) with ethanol as vehicle, respectively, for 7 days, vehicle group was injected with the same dose of ethanol instead. After behavioural test mice were sacrificed by decapitation, the half brain was quickly separated to determine the levels of MDA, SOD, Ach and DA with the ELISA method of kit description, and sapogenin content by HPLC. The other half brain was kept for immunohistochemical analysis. HPLC (Agilent Company, Santa Clara, CA) was performed in the following conditions: column: Diamonsil C18 (150 × 4.6 mm, 5 μm), mobile phase: 80% (V/V) methanol, flow rate: 0.7 mL/min, temperature: 30 °C, injection volume: 15 μL, wavelength: 208 nm.
Behavioural test
The locomotive activities of mice were monitored by a ZZ-6 spontaneous activity apparatus (Chengdu Taimeng Software Co., China). Moving counts were collected by computer with the infrared-sensitive motion-detection system (He et al. Citation2009). Each mouse was placed in the testing chamber for 10 min of adaptation, followed by a 10 min recording.
Assay of TH expression
The test was carried out on the basis of the protocol illustrated by Zhao et al. (Citation2012). The half brain of mice was sunk in paraformaldehyde solution (pH 7.4, 40 g/L) containing 300 g/L sucrose for 48 h, and cryostat sectioned in to slices (50 μm thickness). Brain slices were incubated in TH antibody with dilution of 1:5000, stained by diaminobenzidine (DAB), and mounted to slides for photograph. Optical density of substantia nigra in the photographs was analyzed by Image-Pro Plus 6.0 software (Media Cybernetics Inc, Rockville, MD).
Statistical analysis
Data were expressed as mean ± standard deviation, and analyzed with SPSS13.0 software (IBM, Armonk, NY). Significant tests among the groups were based on one-way analysis of variance (ANOVA) and Student–Newman–Keuls (SNK) test.
Results
Structure of iron–sapogenin
Sapogenin can be chelated with ferric ion in aqueous solution. The product showed single peak in HPLC with the purity of 96% on the base of peak area. It was dissolved in DMSO at 0.2 mg/mL concentration, and scanned by Ultra-violet spectrometer. Iron–sapogenin had two absorption peaks at 276 nm and 370 nm, the former is a characteristic peak of the sapogenin, and the second suggests the linkage of carboxyl group with iron leading to the appearance of a new colour group. Iron–sapogenin has stronger absorbance intensity than the sapogenin, indicating that binding and electron transfer take place between iron atom and sapogenin molecules.
The sapogenin showed a peak of the carbonyl group at 1720 cm−1 at IR spectra, but moved to 1615 cm−1 after chelation with iron (). Its O–H vibration peak at 3419 cm−1 also became wider indicating more hydroxyl groups or water. Occurrence of a new peak at 480 nm suggested M–O vibration.
Carbon and hydrogen composition was determined with an element analyzer, and metals were measured with an atomic absorption spectrometer, the ratios of elements are listed in . It shows that four sapogenin molecules combine with one iron atom.
Table 1. Elemental analysis and mass percentage of metallic elements in the sapogenin and iron–sapogenin.
TG/DTG curves showed that the sapogenin lost its weight quickly from 300 °C to 430 °C without inflection point, but iron–sapogenin had inflection point at 100 °C accompanying quick weight loss from 300 °C to 470 °C and slow weight loss from 470 °C to 800 °C (). It indicates that iron–sapogenin is more stable than the sapogenin, and two molecules of bound water in iron–sapogenin can be calculated by its weight loss. Its molecular structure can be predicted as given in .
Characteristics of the nanoparticles
Iron–sapogenin showed nanoparticle characters while dissolved in DMSO and then dispersed in water. Its size distributed in the range of 100–200 nm, and the average nanoparticle size was 162 nm. PDI of the suspension was 0.258, displaying good dispersity. Morphology of the nanoparticle was observed and photographed with SEM, and the image shows that iron–sapogenin is like a ball with a cave in it (). The special shape indicates that four sapogenin molecules and one iron atom form a sphere with two water molecules as the cave. It means that the complex has more stable nanoparticle structure.
DPPH radical scavenging activity of the nanoparticles
DPPH determination is a classic method to evaluate free radical scavenging activity of the compounds (Musa et al. Citation2013). The sapogenin and its ferric complex could delete DPPH radicals at dose dependence, and iron–sapogenin had stronger activity than the sapogenin. It proves that iron strengthens electron withdrawing capacity of the sapogenin. It scavenged more than 90% DPPH radicals at 0.8 mg/mL concentration, however, vitamin C deleted about 80% at that concentration as shown in .
Effects of the nanoparticles on mice behaviour
Patients with neurodegeneration are usually accompanied by a lot of motor dysfunction, which can be imitated by animal tests with neurotoxicity of rotenone (Moreira et al. Citation2012). Rotenone is the major active ingredients and principal components of cuberesin from Lonchocarpus utilis used as a botanical insecticide and piscicide because of potent complex I (NADH: ubiquinone oxidoreductase) inhibitors, and known earlier to induce a PD-like syndrome after subcutaneous treatment of rats and mice (Caboni et al. Citation2004).
Spontaneous activities of mice, the index to assess behavioural disorder, were determined by locomotion test (Wang et al. Citation2014). The results showed that rotenone significantly (p < 0.05) lessened the spontaneous activity testifying that it could induce movement disorder. The locomotion counts were increased significantly (p < 0.05) after the administration of iron–sapogenin, suggesting that it alleviated the behavioural disorders induced by rotenone, and had better effects than the sapogenin on neuroprotection. The results are shown in .
Table 2. Effect of the sapogenin and iron–sapogenin on behaviour, antioxidant levels and neurotransmitters in brain of mice.
Antioxidant level in brain tissues of mice
MDA and SOD are always used to deduce free radical or antioxidant levels in vivo (Zhou et al. Citation2014). Mice with rotenone had higher level of MDA and lower SOD activity in brain, indicating that rotenone increased free radicals in vivo. A significant (p < 0.05) reduction of MDA content and increase of SOD activity in brain were observed in groups of iron–sapogenin compared with the vehicle (), revealing that the complex of sapogenin with iron is more effective in eliminating free radicals in vivo than the sapogenin.
Neurotransmitters in brain tissues of mice
Dopamine (DA) and acetylcholine (Ach) are important neurotransmitters related to PD, AD and other neurodegeneration (Calabresi et al. Citation2000). Rotenone caused decrease of DA and Ach level in brain, and led to injury of neurons. Sapogenin and iron–sapogenin both prevented the reduction of DA and Ach induced by rotenone, especially iron–sapogenin increased DA and Ach levels in dose dependence, and had no significant difference with normal mice at the dose of 100 mg/kg, showed the stronger neuroprotective effect ().
Protection of TH by the nanoparticles
Tyrosine hydroxylase (TH) is the key enzyme for the synthesis of monoamines neurotransmitters such as dopamine and noradrenaline in neurons, and its expression is lowered in brain tissues of patients with neurodegenerative diseases (Kastner et al. Citation1993). The expression of TH can be reflected by immunostaining intensity of brain slices in immunohistochemistry. Rotenone-treated mice had less staining in the substantia nigra of brain slices as compared with normal mice, indicating that rotenone depressed the expression of TH (). The optical density of the substantia nigra is listed in . The optical intensity has increased from low dose to high dose of the iron–sapogenin. It proves that iron–sapogenin exerts its protective effect on neurons.
Discussion
The neuroprotection of iron–sapogenin is attributed to free radical elimination in vivo. Free radicals with one or more unpaired outer shell electrons are extremely reactive and generally highly unstable. Reactive oxygen species, such as superoxide radical, hydrogen peroxide, hydroxyl radical, and singlet oxygen are main free radicals in body and of the greatest biological significance (Gutowski & Kowalczyk Citation2013). They are extremely reactive and potentially damaging transient chemical species. Free radicals generated by mitochondrial metabolism can be removed by redox system in vivo in healthful condition, however, the balance is broken in illness, and excess free radicals keep active, injure normal brain tissues and induce neurodegeneration (Labunskyy & Gladyshev Citation2013). Some antioxidants show free radical scavenging activities in vitro, but have little activity in vivo, which is crucial to therapeutic effects (Kasote et al. Citation2015). In this experiment, significant changes of MDA and SOD suggest that iron–sapogenin takes effect on neuroprotection through elimination of free radicals in brain.
On view of molecular structure, iron–sapogenin has compact organometallic structure with fewer metals in the centre, and strengthens electron transmittance between iron atom and the sapogenin molecules, which enhances free radical scavenging activity. Inorganic metals and metabolic disorders such as defective lipid metabolism may lead to metal accumulation and neurotoxicity (Nday et al. Citation2012; Colombelli et al. Citation2015), but iron–sapogenin are organic metallic compounds and beneficial to neuroprotection.
Sapogenin content in mice brain increases in dose dependence of iron–sapogenin (), and is about five times in iron–sapogenin group more than sapogenin group at the same dose (100 mg/kg). Iron may increase the permeability of blood–brain barrier through the upregulation of transferrin receptor mRNA after treatment with the iron chelator (Hersom et al. Citation2016). It is the possible mechanism of greater neuroprotective effects caused by iron–sapogenin complex.
Conclusion
The sapogenin, a bioactive compound isolated from the seeds of C. oleifera, can react with iron to form the chelate of iron–sapogenin. Its structure is deduced as four sapogenin molecules binding to one iron atom with two water molecules. It possesses nanoparticle characters with spherical shape. The linkage of sapogenin with iron strengthens electron transfer among molecules, improves free radical scavenging activity. Iron–sapogenin protects brain from damage caused by neurotoxicity of rotenone, plays an important role in antioxidative level in central tissues. It is a good candidate as a drug for neuroprotection.
Acknowledgements
The authors would like to thank the staff in South China University of Technology for data analysis and ethical committee for approval of animal tests.
Disclosure statement
The authors report no declaration of interest.
Funding
The financial support from Guangzhou Scientific Plan Project [No. 1563000123] and Guangdong Scientific Plan Project [No. 2016B090918086] is gratefully acknowledged.
References
- Bae EJ, Lee HJ, Rockenstein E, Ho DH, Park EB, Yang NY, Desplats P, Masliah E, Lee SJ. 2012. Antibody-aided clearance of extracellular α-synuclein prevents cell-to-cell aggregate transmission. J Neurosci. 32:13454–13469.
- Balducci C, Forloni G. 2014. In vivo application of beta amyloid oligomers: a simple tool to evaluate mechanisms of action and new therapeutic approaches. Curr Pharm Des. 20:2491–2505.
- Bellingham SA, Guo B, Hill AF. 2015. The secret life of extracellular vesicles in metal homeostasis and neurodegeneration. Biol Cell. 107:389–418.
- Caboni P, Sherer TB, Zhan N, Taylor G, Na HM, Greenamyre JT, Casida JE. 2004. Rotenone, deguelin, their metabolites, and the rat model of Parkinson's disease. Chem Res Toxicol. 17:1540–1548.
- Calabresi P, Centonze D, Gubellini P, Marfia GA, Pisani A, Sancesario G, Bernardi G. 2000. Synaptic transmission in the striatum: from plasticity to neurodegeneration. Prog Neurobiol. 61:231–265.
- Colombelli C, Aoun M, Tiranti V. 2015. Defective lipid metabolism in neurodegeneration with brain iron accumulation (NBIA) syndromes: not only a matter of iron. J Inherit Metab Dis. 38:123–136.
- Duty S. 2010. Therapeutic potential of targeting group III metabotropic glutamate receptors in the treatment of Parkinson's disease. Br J Pharmacol. 161:271–287.
- Fernandez HH. 2015. 2015 Update on Parkinson disease. Cleve Clin J Med. 82:563–568.
- Gao DF, Zhang YJ, Yang CR, Chen KK, Jiang HJ. 2008. Phenolic antioxidants from green tea produced from Camellia taliensis. J Agric Food Chem.56:7517–7521.
- Gutowski M, Kowalczyk S. 2013. A study of free radical chemistry: their role and pathophysiological significance. Acta Biochim Polonica. 60:1–16.
- Han YX, Wei XB, Xia LI, Ding Y, Xin H, Ding H. 2008. Protective effects of gypenosides against glutamate-induced hippocampus injury in rats. J Shandong Univ. 32:1683–1688.
- He M, Zhao L, Wei MJ, Yao WF, Zhao HS, Chen FJ. 2009. Neuroprotective effects of (-)-epigallocatechin-3-gallate on aging mice induced by D-galactose. Biol Pharm Bull. 32:55–60.
- Hersom M, Helms HC, Pretzer N, Goldeman C, Jensen AI, Severin G, Nielsen MS, Holm R, Brodin B. 2016. Transferrin receptor expression and role in transendothelial transport of transferrin in cultured brain endothelial monolayers. Mol Cell Neurosci. 76:59–67.
- Jabir NR, Firoz CK, Baeesa SS, Ashraf GM, Akhtar S, Kamal W, Kamal MA, Tabrez S. 2014. Synopsis on the linkage of Alzheimer's and Parkinson's disease with chronic diseases. CNS Neurosci Ther. 21:1–7.
- Kasote DM, Katyare SS, Hegde MV, Bae H. 2015. Significance of antioxidant potential of plants and its relevance to therapeutic applications. Int J Biol Sci. 11:982–991.
- Kastner A, Hirsch EC, Agid Y, Javoy-Agid F. 1993. Tyrosine hydroxylase protein and messenger RNA in the dopaminergic nigral neurons of patients with Parkinson's disease. Brain Res. 606:341–345.
- Kumar A, Singh A. Ekavali. 2015. A review on Alzheimer's disease pathophysiology and its management: an update. Pharmacol Rep. 67:195–203.
- Labunskyy VM, Gladyshev VN. 2013. Role of reactive oxygen species-mediated signaling in aging. Antioxid Redox Signal. 19:1362–1372.
- Lozoff B, Beard J, Connor J, Barbara F, Georgieff M, Schallert T. 2006. Long-lasting neural and behavioral effects of iron deficiency in infancy. Nutr Rev. 64:S34–S43.
- Luo YM, Li B, Xie YH. 2003. Study on the chemical constituents of Camellia oleifera Abel. Chin Trad Herbal Drugs. 34:117–118.
- Ma XY, Liu SB, Lu L, Li SF, Xie JJ, Zhang LY, Zhang JH, Luo XG. 2014. Relative bioavailability of iron proteinate for broilers fed a casein-dextrose diet. Poult Sci. 93:556–563.
- Moreira CG, Barbiero JK, Ariza D, Dombrowski PA, Sabioni P, Bortolanza M, Da Cunha C, Vital MA, Lima MM. 2012. Behavioral, neurochemical and histological alterations promoted by bilateral intranigral rotenone administration: a new approach for an old neurotoxin. Neurotox Res. 21:291–301.
- Murakami S, Miyazaki I, Sogawa N, Miyoshi K, Asanuma M. 2014. Neuroprotective effects of metallothionein against rotenone-induced myenteric neurodegeneration in Parkinsonian mice. Neurotox Res. 26:285–298.
- Musa KH, Abdullah A, Kuswandi B, Hidayat MA. 2013. A novel high throughput method based on the DPPH dry reagent array for determination of antioxidant activity. Food Chem. 141:4102–4106.
- Nday CM, Malollari G, Petanidis S, Salifoglou A. 2012. In vitro neurotoxic Fe(III) and Fe(III)-chelator activities in rat hippocampal cultures. From neurotoxicity to neuroprotection prospects. J Inorg Biochem. 117:342–350.
- Ohno Y, Shimizu S, Tokudome K, Kunisawa N, Sasa M. 2015. New insight into the therapeutic role of the serotonergic system in Parkinson’s disease. Prog Neurobiol. 134:104–121.
- Valerio LG. 2007. Mammalian iron metabolism. Toxicol Mech Methods. 17:497–517.
- Venigalla M, Gyengesi E, Sharman MJ, Münch G. 2015. Novel promising therapeutics against chronic neuroinflammation and neurodegeneration in Alzheimer’s disease. Neurochem Inter. 10:1181–1185.
- Venkateshappa C, Harish G, Mahadevan A, Srinivas Bharath MM, Shankar SK. 2012. Elevated oxidative stress and decreased antioxidant function in the human hippocampus and frontal cortex with increasing age: implications for neurodegeneration in Alzheimer’s disease. Neurochem Res. 37:1601–1614.
- Wang P, Niu L, Guo XD, Li WX, Jia D, Wang XL, Ma LT, Gao GD. 2010. Gypenosides protect dopaminergic neurons in primary culture against MPP+-induced oxidative injury. Brain Res Bull. 83:266–271.
- Wang Z, Zhang Q, Yuan L, Wang S, Liu L, Yang X, Li G, Liu D. 2014. The effects of curcumin on depressive-like behavior in mice after lipopolysaccharide administration. Behav Brain Res. 274:282–290.
- Wei J, Chen XL, Sun H, Shen GX. 2012. Research progress on breeding and utilization of oil used Camellia L. Acta Agriculturae Zhejiangensis. 24:533–540.
- Ye R, Yang Q, Kong X, Han J, Zhang X, Zhang Y, Li P, Liu J, Shi M, Xiong L, et al. 2011. Ginsenoside Rd attenuates early oxidative damage and sequential inflammatory response after transient focal ischemia in rats. Neurochem Inter. 58:391–398.
- Ye Y, Chen X, Xing HT. 2013a. Hypolipidemic and antioxidant activities of hydrolyzed saponins from defatted seeds of Camellia oleifera Abel. Lat Am J Pharm. 32:409–417.
- Ye Y, Fang F, Li Y. 2014. Isolation of the sapogenin from defatted seeds of Camellia oleifera and its neuroprotective effects on dopaminergic neurons. J Agric Food Chem. 62:6175–6182.
- Ye Y, Xing HT, Chen X. 2013. Anti-inflammatory and analgesic activities of the hydrolyzed sasanquasaponins from the defatted seeds of Camellia oleifera. Arch Pharm Res. 36:941–951.
- Zhao B. 2009. Natural antioxidants protect neurons in Alzheimer's disease and Parkinson's disease. Neurochem Res. 34:630–638.
- Zhao YM, Li JQ, Lv FY, Yan Y, Xu QY. 2012. Activation of microglia and dopaminergic neurons degeneration following intraventricular injection of LPS in the substantia nigra of rats. Chin J Neuroimmunol Neurol. 19:121–145.
- Zhou Q, Yin ZP, Ma L, Zhao W, Hao HW, Li HL. 2014. Free radical-scavenging activities of oligomeric proanthocyanidin from Rhodiola rosea L. and its antioxidant effects in vivo. Nat Prod Res. 28:2301–2303.