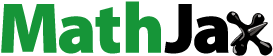
Abstract
Context: Sonchus oleraceus L. (Asteraceae) (SO) is a dietary and traditional medicinal plant in China. However, its underlying mechanism of action as an anti-inflammatory agent is not known.
Objective: This study evaluates the anti-inflammatory activity of aqueous extract of SO.
Materials and methods: The extract of SO was used to treat RAW 264.7 cells (in the working concentrations of 500, 250, 125, 62.5, 31.3 and 15.6 μg/mL) for 24 h. Pro-inflammatory cytokines and mediators produced in LPS-stimulated RAW 264.7 cells were assessed. Meanwhile, the expression level of TLR-4, COX-2, pSTATs and NF-κB was tested. Moreover, the anti-inflammatory activity of the extract in vivo was assessed using xylene-induced mouse ear oedema model and the anti-inflammatory compounds in the extracts were analyzed by HPLC-MS.
Results: SO extract significantly inhibited the production of pro-inflammatory cytokines and mediators at gene and protein levels with the concentration of 31.3 μg/mL, and suppressed the expression of TLR-4, COX-2, NF-κB and pSTAT in RAW 264.7 cells. The anti-inflammatory activity of SO in vivo has significant anti-inflammatory effects with the concentration of 250 and 125 mg/kg, and less side effect on the weights of the mice at the concentration of 250 mg/kg. Moreover, HPLC-MS analysis revealed that the anti-inflammatory compounds in the extract were identified as villosol, ferulaic acid, β-sitosterol, ursolic acid and rutin.
Discussion and conclusion: This study indicated that SO extract has anti-inflammatory effects in vitro and in vivo, which will be further developed as novel pharmacological strategies in order to defeat inflammatory diseases.
Introduction
Inflammation is a defence mechanism against various infections and injuries that induces fever, swelling, pain and various functional disorders. Acute inflammation can cause serious damage and even death in extreme cases (Angus et al. Citation2001). When acute inflammation shifts to chronic inflammation, other diseases may be induced, including cancer, atherosclerosis, osteoarthritis and Alzheimer’s disease (Ferrucci et al. Citation2004; Vasto et al. Citation2007). How to control inflammation and inflammation-mediated illnesses remains a serious challenge. The exploration and development of anti-inflammatory drugs are major issues in current medical science.
Macrophages, the representative immune cells involved in innate immunity, play a key role in triggering inflammatory reactions by secreting pro-inflammatory cytokines, such as tumour necrosis factor (TNF)-α, interleukin (IL)-1β, IL-6 and inflammatory mediators, including nitric oxide (NO), cyclooxygenase-2 (COX-2) and prostaglandin E2 (PGE2) (Raetz & Whitfield Citation2002; Woods et al. Citation2009). As a component of Gram-negative bacteria membranes, lipopolysaccharide (LPS) is known to stimulate immune cells to secrete pro-inflammatory cytokines and is often used as an established model in inflammation research.
Sonchus oleraceus L. (Asteraceae) (SO) is a dietary and traditional medicinal plant in Chinese folk culture that can be cooked and eaten to treat inflammatory disease. Currently, studies have indicated that the extract of SO exert many bio-activities, including antioxidant (McDowell et al. Citation2011; Yin et al. Citation2007), antibacterial (Xia et al. Citation2011), anxiolytic (Cardoso Vilela et al. Citation2009), antinociceptive (Vilela et al. Citation2009), anti-ageing (Ou et al. Citation2015), antitumour (Volynskii Iu et al. Citation1976; Han et al. Citation2005) and anti-inflammatory properties (Vilela et al. Citation2010). But, its underlying mechanism of the anti-inflammatory action is unknown.
In this study, the anti-inflammatory effects of SO extract were evaluated using a LPS-stimulated RAW 264.7 cell (mouse macrophages) model. The production levels of the pro-inflammatory cytokines, TNF-α, IL-1β and IL-6, and other inflammatory mediators, including NO and PGE-2, in RAW 264.7 cells were tested. The expression levels of cyclooxygenase-2 (COX-2), Toll-like receptor 4 (TLR-4), nuclear factor-κB (NF-κB) and phosphorylated (activated) signal transducers and activators of transcription (pSTAT) 1 and pSTAT3 in RAW 264.7 cells were also analyzed. Then, the anti-inflammatory effects of SO extract in vivo were assessed using the xylene-induced mouse ear oedema model. Furthermore, the bioactive compounds in the SO extracts were evaluated by HPLC-MS analysis.
Materials and methods
Preparation of Sonchus oleraceus extracts
Entire plants of S. oleraceus were provided by the Desert Plants & Ecological Civilization Research Center of Northwest University. Dried plant material (100 g) was extracted with 1 L of doubly distilled (dd) water at 70 °C for 48 h. The final aqueous extract was lyophilized in a freeze dryer (ALPHA 1-2/LD-2, Marin Christ, Osterode, Germany), and the lyophilized powder was collected and stored at −20 °C.
Cells
The RAW 264.7 cell line was purchased from the American Type Culture Collection (ATCC, Manassas, VA) and maintained in DMEM cell culture media (Gibco BRL, Gaithersburg, MD) supplemented with 10% foetal calf serum, 100 IU/mL penicillin, and 100 mg/mL streptomycin (Genview, Carlsbad, CA) in a humidified 5% CO2 atmosphere at 37 °C.
Cell viability and proliferation assay
Lyophilized powder of S. oleraceus (SO) extract was prepared as a storage solution (100 × working solution) at 50 mg/mL and as gradient diluted solutions (50, 25, 12.5, 6.25, 3.13 and 1.56 mg/mL). RAW 264.7 cells (5 × 105) were suspended in 200 μL DMEM media with the 1% storage solutions of the SO extract (working concentrations of 500, 250, 125, 62.5, 31.3 and 15.6 μg/mL) and cultured in 24-well plates (Costar, New York, NY) at 37 °C in a 5% CO2 incubator for 24 h. After treatment with the SO extract, the RAW 264.7 cells in each group were dissociated, collected by centrifuging at 1000 g for 5 min, washed with PBS and re-suspended in 600 μL DMEM media. Then, 20 μL CCK-8 (Cell Counting Kit-8, Dojindo, Japan) was added to 200 μL cell suspensions per well in a 96-well plate in triplicate for each group. The same quantity of cells without extract was used as the control group. The 96-well plate was incubated for 2 h at 37 °C in a 5% CO2 incubator. The absorbance (A) values per well were recorded at 450 nm using a microplate reader (BioTek Synergy-4, VT). The cells in the control group were considered as 100% viable. The inhibition rate was calculated according to the following equation:
Pro-inflammatory-related gene expression assay
After treatment with LPS (1 μg/mL) alone, with SO extract, or with dexamethasone (1 μmol/mL as the positive control) for 24 h, the total RNA of the RAW 264.7 cells was isolated using TRIzol reagent (Invitrogen, Carlsbad, CA). Relative quantitative PCR (RT-qPCR) and the SYBR Green random mixing method were used to test the mRNA expression of pro-inflammatory cytokines and mediators: TNF-α, IL-1β, IL-6, COX-2 and PGE2. Glyceraldehyde-3-phosphate dehydrogenase (GAPDH) gene was used as the internal control. Relative changes in gene expression were calculated according to the 2−ΔΔCt method (Livak & Schmittgen Citation2001). All primers used are shown in . RT-qPCR was performed according to the instructions of the TransStart Top Green qPCR SuperMix kit (TransGen Biotech, Beijing, China). The reaction mixtures were incubated for 30 min at 48 °C, followed by 40 cycles of PCR at 94 °C for 5 s, 55 °C for 15 s and 72 °C for 10 s. At the end of 40 cycles, a melting curve analysis was conducted to confirm the existence of an amplified product in the expected size.
Table 1. Primer pairs used for RT-qPCR analysis.
Analysis of TLR-4, COX-2 and pSTATs
The expression of TLR-4 and COX-2 in the RAW 264.7 cells were analyzed using flow cytometry. The pSTAT1 and pSTAT3 were also analyzed to explore the related signalling pathway by which the extracts acted on the cells.
After treatment, RAW 264.7 cells in each group were dissociated to pellets (1 × 105 each) and washed twice with PBS, and then the TLR-4 antibody (eBioscience, Shanghai, China) was added to the cells. After staining for 10 min, the pellets were analyzed by flow cytometry. By using fixation/permeabilization concentrate (Affymetrix eBioscience, San Diego, CA) pre-permeated, the remaining prepared pellets were stained with pSTAT1, pSTAT3 (Santa Cruz Biotechnology, Dallas, TX) or COX-2 antibodies (Abcam, Shanghai, China) for 10 min to test the pSTATs and COX-2 levels.
Western blot assay for NF-κB
For Western blot analysis, RAW 264.7 cells in each group (2 × 107 each) were dissociated, washed with PBS and re-suspended in RIPA lysis buffer (CWbiotech, Beijing, China) supplemented with protease inhibitor (CWbiotech, Beijing, China) on ice for 20 min. The lysate of the RAW 264.7 cells was quantified via a BCA (BCA Protein Assay Kit, Beyotime Institute of Biotechnology, Haimen, China) assay. The lysate was separated by 12% Tris-glycine gels in equal amounts, and then transferred onto polyvinylidene fluoride (PVFD) membranes. Under gentle shaking, the blots were first blocked in 5% nonfat milk for 1 h. After washing in TBST three times, the blots were incubated with NF-κB primary antibody (Abcam, MA, 1:5000 dilution) and anti-β actin antibody (TransGen Biotech, Beijing, China, 1:5000 dilution) overnight at 4 °C. After washing in TBST three times, the blots were incubated with the subsequently secondary antibodies (Goat anti-rabbit IgG, HRP conjugated, Zhuangzhibio, Xi’an, China, 1:2000 dilution) at 4 °C for 50 min under gentle shaking. The blots were washed again with TBS, and then were immersed in the luminous liquid (EasySee® Western Blot Kit, TransGen Biotech, Beijing, China) for 1 min. The signals were detected by exposure of the film in a darkroom. The protein bands were quantified using PhotoShop software, and the relative ratio (grey value(experiment)/grey value(internal control)) was calculated.
Test for TNF-α, IL-1β, IL-6, PGE2 and NO
The secretion of pro-inflammatory cytokines (TNF-α, IL-1β and IL-6) and PGE2 in the RAW 264.7 cells were assessed using the corresponding ELISA Kits (BD Bioscience, CA; R&D Systems, Minneapolis, MN). RAW 264.7 cells in each group (8 × 105) were centrifuged. The cell-free supernatants were harvested and concentrated by using an ultrafiltration cube (Millipore, Shanghai, China). Then, the pro-inflammatory cytokines and mediators in the supernatants were assessed by using specific ELISA kits. Each sample was tested twice, and the A values were recorded at 450 nm and 570 nm. According to the A values (A450–570), standard curves were created by using the standards in Curve Expert 13.0. The quantities of these cytokines secreted by RAW 264.7 cells were calculated from each of the standard curves. NO concentration was measured using an NO detection kit (Jiancheng Bioengineering Institute, Nanjing, China) according to the instructions.
Xylene-induced mouse ear oedema
Fifty Kunming (KM) mice (adult males weighing between 16.32–21.02 g, mean 18.6 ± 2.1 g) provided by the Department of Laboratory Animal Science of Xi'an Jiaotong University were used in the ear oedema experiment. This animal study was approved by the Ethic Committees of Northwestern Polytechnical University (License: NWPU:2015002). All animals were housed under standardized animal house conditions (12 h light/dark cycle at 22 °C, and relative humidity 50%) with free access to food and water. All experimental procedures conformed to the IACUC policy.
Xylene was used to induce ear oedema based on a previously described method with minor modifications (Saraiva et al. Citation2011). The mice were randomly divided into five groups (10 in each group) (SO low, SO medium, SO high, positive control and control groups) and orally administered SO extract (0.5 mL each) at three concentration gradients (low: 125 mg/kg; medium: 250 mg/kg; and high: 500 mg/kg) or acetylsalicylic acid (100 mg/kg serving as the positive control) for five consecutive days. The control group was orally administered with 0.5 mL dd water. After 1 h of the final feeding, 50 μL xylene was applied with a micropipette to the left ears of the mice, and the right ears served as controls. After 45 min of treatment, the mice were sacrificed. Biopsies of the ears were obtained using a 45 mm diameter punch. The ear oedema was evaluated by measuring the weight of ear slice. The oedema rate of the ear was calculated according to the following equation:
Determination of major bioactive compounds in the S. oleraceus aqueous extract
To measure the major compounds in the S. oleraceus extract, HPLC-MS analysis was performed using an Agilent 1200 series equipped (Agilent Technologies, Santa Clara, CA) with a C18 column (XBridge 5 μm, 4.6 × 150 mm) and Agilent online and offline LC-MS software. After dissolving the lyophilized powder, the solution of extract was filtered through a filter membrane (0.45 μm, Navigator nylon organic filter). The column was operated at 40 °C. The mobile phases consisted of A (0.05% aq. trifluoroacetic acid, HPLC grade, H2O) and B (acetonitrile). B multistep gradient was used for all analyses, as follows: 0–10 min, 0–30% (v/v) B; 10–13 min, 30% B; 13–15 min, 0% B. The flow rate was 1.0 mL/min, and the sample injection volume was 8 μL. The operating parameters were adjusted as follows: ion source electrospray capillary voltage of 3000 V and capillary temperature of 350 °C. Helium was used as the collision gas and nitrogen as the nebulizing gas. Desolvation was assisted using a counter current nitrogen flow set at a flux of 12 L/min. Full scan mass acquisitions were performed in both negative and positive ion modes by scanning the m/z range from 200 to 1200 mass units. By using helium as the collision gas, collision-induced dissociation (CID) spectra were obtained in the ion trap with voltage ramping cycles from 5 to 30 V. Characterization of the constituents was carried out based on the UV and mass spectra data, together with the fragmentation profiles obtained through mass spectrometry (MS spectra), which were compared with literature data and also based on constituents reported for this species in other studies.
Data analysis
Statistical analyses and IC50 calculations were performed by using SPSS 16.0 statistical software (IBM, Armonk, NY). The data were presented as the mean ± SE. The results were analyzed using analysis of variance (ANOVA). Multiple comparisons were performed using the LSD test to evaluate significant differences between the groups. Statistical significance was defined as p < 0.05.
Results
Cell viability and IC50
The results showed that the SO extract could not inhibit the viability of the RAW 264.7 cells at a low concentration. The cells in the control group were considered as 100% viable. As shown in , when the concentrations of SO extract were from 15.6 to 500 μg/mL, the viabilities of the RAW 264.7 cells were 96.71 ± 1.62%, 95.32 ± 1.96%, 76.29 ± 5.48%, 71.52 ± 5.19%, 66.70 ± 4.04% and 62.20 ± 5.02%, respectively. The IC50 value of the SO extract on the RAW 264.7 cells was 702.8 μg/mL by using SPSS 16.0 statistical software. The SO extract administered at 31.3 μg/mL had no significant inhibitory effects on the RAW 264.7 cell viability. For this reason, this concentration was used in the subsequent experiments.
Figure 1. Viabilities and IC50 of RAW 264.7 cells after SO extract treatment. Viability and IC50 of RAW 264.7 cells after S. oleraceus L. (SO) extract treatment, the data derived from absorbance value (A value) (Inhibition rate (%) = (1−A (experiment)/A(control)) × 100%.) and represents mean ± SE of six independent experiments. One-way ANOVA and LSD test (n = 6).

SO inhibited the gene expression of pro-inflammatory cytokines and mediators
By using the RT-qPCR method, the expression levels of inflammatory-related genes (TNF-α, IL-1β, IL-6, COX-2 and PGE2) were assessed. After stimulation by LPS, the expression levels of inflammatory-related genes in the RAW 264.7 cells were significantly up-regulated to 3.29-fold (TNF-α), 3.58-fold (IL-1β), 4.19-fold (IL-6), 2.27-fold (COX-2) and 3.94-fold (PGE2) compared with the control. The gene expression levels decreased in the presence of SO extract and dexamethasone. In the SO group, the expression levels of genes were 0.80-fold (TNF-α), 1.22-fold (IL-1β), 1.44-fold (IL-6), 1.72-fold (COX-2) and 1.14-fold (PGE2) of the control levels. The expression levels in the positive control group were 0.97-fold (TNF-α), 0.86-fold (IL-1β), 1.31-fold (IL-6), 1.18-fold (COX-2) and 1.03-fold (PGE2) of the control levels ().
Table 2. SO extract and dexamethasone down-regulated pro-inflammatory related genes in LPS stimulated RAW 264.7 cells (n = 6).
SO inhibited the expression of pro-inflammatory cytokines and NO
The expression levels of pro-inflammatory cytokines were analyzed via ELISA (). The results showed that after stimulation by LPS, the expression levels of TNF-α, IL-1β, IL-6 and PGE2 significantly increased to 1662.7 ± 83.1 pg/mL (TNF-α), 119.7 ± 18.4 pg/mL (IL-1β), 243.7 ± 34.6 pg/mL (IL-6) and 2192.9 ± 177.6 pg/mL (PGE2) compared with 426.3 ± 86.1 pg/mL (TNF-α), 20.9 ± 7.7 pg/mL (IL-1β), 13.7 ± 15.4 pg/mL (IL-6) and 777.0 ± 92.2 pg/mL (PGE2), respectively, in the control group. Compared with positive control, SO extracts had the same effects on inhibiting the expression of TNF-α and PGE2. The expression levels of the two types of cytokines were 1136.0 ± 150.3 pg/mL (TNF-α) and 1420.1 ± 137.2 pg/mL (PGE2) in the SO group compared with 1147.3 ± 174.4 pg/mL (TNF-α) and 1342.6 ± 98.7 pg/mL (PGE2), respectively, in the positive control group. The effects of the SO extract on IL-1β and IL-6 were different from dexamethasone, while compared with the LPS group, the expression of the two interleukins were significantly decreased. SO extracts also could decrease the NO level in the culture supernatants. The NO concentration was approximately 15.3 ± 1.5 μmol/L in the SO group and 13.3 ± 2.5 μmol/L in the positive control, which was markedly reduced compared with 31.3 ± 3.5 μmol/L in LPS-stimulated group.
Figure 2. Pro-inflammatory cytokines and NO secretion of RAW 264.7 cell LPS stimulated RAW 264.7 were treated by SO extract or dexamethasone (Positive Control, PC). The supernatants were collected and the concentrations of TNF-α, IL-1β, IL-6, PGE-2 and NO were detected, respectively. (A) The secretion level of IL-1β in supernatant; (B) the secretion level of IL-6 in supernatant; (C) the secretion level of TNF-α in supernatant; (D) the secretion level of PGE2 in supernatant; (E) the secretion level of NO in supernatant. The data represents mean ± SE of three independent experiments. One-way ANOVA and LSD tests were performed, *p < 0.05 compared with the control (n = 4). #p < 0.05 compared with the LPS group.

SO extracts inhibited the expression of COX-2 and TLR-4
The expression of COX-2 and TLR-4 on RAW 264.7 cells in each group were assessed by flow cytometry. As shown in and , the results indicated that the expression of TLR-4 and COX-2 were both increased after LPS stimulation. The percentage of COX-2 in the RAW 264.7 cells in the control group was 64.95 ± 3.12%, and the mean fluorescence intensity (MFI) was 52.86 ± 4.56. The results of TLR-4 in the control group were 30.79 ± 3.11% and 49.05 ± 4.26 (MFI). After LPS stimulation, the percentage of COX-2 was increased to 85.92 ± 4.50%, and the percentage of TLR-4 was also increased to 54.33 ± 3.73%. The MFI of COX-2 also increased to 62.38 ± 3.49. However, the MFI of TLR-4 had no significant change, which was 51.01 ± 2.31 in the presence of LPS. The SO extracts inhibited the expression of both COX-2 and TLR-4 in LPS-stimulated RAW 264.7 cells, which induced the same effects as positive control. In the positive control group, the % and MFI of COX-2 were 74.89 ± 3.89% and 39.86 ± 2.13, respectively, and for TLR-4 were 32.60 ± 2.15% and 51.43 ± 2.1, respectively. In the SO group, the % and MFI were 72.92 ± 2.53% and 45.06 ± 4.23 for COX-2 and 43.36 ± 2.92% and 53.57 ± 4.19 for TLR-4, respectively (p < 0.05 compared with LPS stimulated group).
Figure 3. The expression of TLR-4 in RAW 264.7 cells. (A) The expression of TLR-4 in RAW 264.7 cells was tested by flow cytometry (a representative flow cytometry peak figure); (B) The bar graph showed percentage and mean fluorescence intensity (MFI) of TLR-4 on RAW 264.7 cells. Dexamethasone (Positive control, PC). The data represent the mean ± SE from four independent experiments. One-way ANOVA and LSD test. *p < 0.05 compared with control. #p < 0.05 compared with the LPS group (n = 4).

Figure 4. The expression of COX-2 in RAW 264.7 cells. (A) The expression of COX-2 in RAW 264.7 cells was tested by flow cytometry (a representative flow cytometry peak figure); (B) the bar graph showed positive percentage and mean fluorescence intensity (MFI) of COX-2 on RAW 264.7 cells. Dexamethasone (Positive control, PC). The data represent the mean ± SE from four independent experiments. One-way ANOVA and LSD test, *p < 0.05 compared with control. #p < 0.05 compared with the LPS group (n = 4).

SO extracts decreased the NF-κB, pSTAT1 and pSTAT3 levels
The potential signalling factors correlated with the anti-inflammatory effects of SO extract were assayed by testing for the expression of NF-κB, pSTAT1 and pSTAT3. The Western blot results showed that stimulation by LPS-induced cells to express more NF-κB (). By analyzing the grey value, the SO extract treatment decreased the expression of NF-κB in the LPS-stimulated cells, but the effects were not equivalent to the treatment with dexamethasone. The relative ratios in each group were 59.79 ± 4.09% (control group), 61.16 ± 2.44% (positive group) and 77.64 ± 2.80% (SO group) compared with 86.65 ± 2.77% (LPS group). The production levels of pSTAT1 and pSTAT3 in cells were also analyzed by flow cytometry. The results showed that LPS stimulation could increase the MFI of pSTAT1 rather than increase its percentages ( and ). The MFI of pSTAT1 in the LPS group was 117.21 ± 4.75 compared with 56.5 ± 4.95 in the control. Moreover, the LPS did not affect the percentage or MFI of pSTAT3 in the RAW 264.7 cells. In the presence of dexamethasone and SO extracts, the MFI of both pSTAT1 and pSTAT3 were decreased to 74.84 ± 3.75 (dexamethasone) and 74.02 ± 2.58 (SO extracts) for pSTAT1 and 122.69 ± 3.96 (dexamethasone) and 108.27 ± 2.53 (SO extracts) for pSTAT3 (p < 0.05 compared with LPS stimulated group).
Figure 5. The expression of NF-κB in RAW 264.7 cells. (A) The expression of NF-κB in RAW 264.7 cells was tested by Western-blot, the β-actin was used as the internal control. (B) the bar graph of relative ratio (grey value (experiment)/grey value (internal control)). Dexamethasone (Positive control, PC). One-way ANOVA and LSD test, *p < 0.05 compared with control. #p < 0.05 compared with the LPS group (n = 4).

Figure 6. The expression of pSTAT1 in RAW 264.7 cells. (A) The expression of pSTAT1 in RAW 264.7 cells was tested by flow cytometry (a representative flow cytometry peak figure); (B) The bar graph showed positive percentage and mean fluorescence intensity (MFI) of pSTAT1 on RAW 264.7 cells. Dexamethasone (Positive control, PC). The data represent the mean ± SE from four independent experiments. One-way ANOVA and LSD test, *p < 0.05 compared with control; #p < 0.05 compared with the LPS group (n = 4).

Figure 7. The expression of pSTAT3 in RAW 264.7 cells. (A) The expression of pSTAT3 in RAW 264.7 cells was tested by flow cytometry (a representative flow cytometry peak figure); (B) The bar graph showed positive percentage and mean fluorescence intensity (MFI) of pSTAT1 on RAW 264.7 cells. Dexamethasone (Positive control, PC). The data represent the mean ± SE from four independent experiments. One-way ANOVA and LSD test, *p < 0.05 compared with control; #p < 0.05 compared with the LPS group (n = 4).

SO extracts reduced the oedema of mouse ears
Fifty KM mice were used to analyze the anti-inflammatory effects of the SO extract in vivo. After 5 days of oral administration, the SO extract induced inhibitory effects on mouse ear oedema at low and mid-level concentrations (). The oedema rates of each group were 81.88 ± 17.63% (SO low concentration group), 62.99 ± 19.57% (SO medium concentration group), 105.21 ± 20.26% (SO high concentration group) and 71.06 ± 16.20% (PC) compared with 108.70 ± 16.34% of the control group. It was shown that SO exerts significant anti-inflammatory effects at the mid-level concentration (250 mg/kg) and low concentration (125 mg/kg). The optimum concentration was 250 mg/kg, which induced greater effects than acetylsalicylic acid and had less effect on the weights of the mice.
Figure 8. The oedema rate of ear and increased body weight in mice model. (A) The oedema rate of mice ear; (B) the increased body weight. (PC: Positive Control) Dexamethasone (Positive control, PC). The data represent the mean ± SE from four independent experiments. One-way ANOVA and LSD test, *p < 0.05 compared with control (n = 10).

Analysis of bioactive compounds in S. oleraceus aqueous extracts
The main bioactive compounds in S. oleraceus aqueous extract were analyzed by HPLC-MS (). According to the fragmentation profiles of standard samples (Li Citation2006), six main bioactive compounds were detected in the extracts: villosol, ferulaic acid, β-sitosterol, ursolic acid, rutin and β-daucosterin. The contents of these compounds were 2.21% (villosol), 0.39% (ferulaic acid), 6.15% (β-sitosterol), 0.49% (ursolic acid), 7.8% (rutin) and 0.98% (β-daucosterin).
Figure 9. LC-MS analysis of bioactive compounds in the SO extracts. (A) LC-UV chromatogram monitored at 220 nm and LC-MS positive-ion ESI-MS total ion current (TIC) profile. (B) (a) Villosol at RT 5.689; (b) Ferulaic acid at RT 5.753; (c) β-Sitosterol at RT 7.355; (d) Ursolic acid at RT 9.674; (e) Rutin at RT 9.859; (f) β-Daucosterin at RT 10.704.

Discussion
Inflammation is a complex process that is induced by interactions between soluble factors and cells. When responding to traumatic, infectious, toxic or/and autoimmune injury, the body induces inflammation (Nathan Citation2002). Macrophages are important immunocytes and play a key role in the inflammatory process by secreting many pro-inflammatory cytokines and mediators. Inflammation and the control and relief of the corresponding diseases represent universal challenges in medical science.
Sonchus oleraceus (SO) has been used to treat many types of inflammation, including swelling and pain, dysentery, appendicitis, arthritis and so on, in China. Although anti-inflammatory herbs have been used throughout history, the underlying mechanism has not been elucidated.
By using an LPS-stimulated RAW 264.7 cell model, the anti-inflammatory effects of SO extract were thoroughly assessed in the present study. It was shown that the IC50 of SO extract on RAW 264.7 cells was 702.8 μg/mL, and when the concentration of SO extract decreased to 31.3 μg/mL, there were almost no inhibitory effects on RAW 264.7 cells viability. For this reason, the concentration (31.3 μg/mL) was used to assess the effects of SO extract in LPS-induced inflammation in the subsequent experiments.
After activation by LPS, macrophages secrete TNF-α, which induces many physiological changes, including inflammation, septic shock and cytotoxicity (Doufas et al. Citation2013), and then TNF-α triggers the production of IL-1β and IL-6 (Beutler & Cerami Citation1988; Zhang & An Citation2007). IL-1β and IL-6 are small molecules that modulate inflammation and immunity. In this study, the results showed that the SO extract decreased the production of these three cytokines and acted to inhibit the production of TNF-α, similar to dexamethasone.
As the most abundant prostaglandin in the human body, PGE2 is an important mediator of inflammatory symptoms, including fever and pain. PGE2 is synthesized by the COX enzyme that is expressed in all processes of inflammation (Funk Citation2001; Lee et al. Citation2011). The results of this study showed that SO extracts could inhibit the production of PGE2 via inhibiting the expression of COX-2. NO is generated by nitric oxide synthases (NOSs) and is known as an inflammation modulator involved in various physiological functions (Bogdan Citation2001). The production of NO was also inhibited by SO extract, which served as the positive control.
Macrophages express TLRs that play key roles in recognizing microbial flora and inducing subsequent immune responses (Yayeh et al. Citation2012). Studies have demonstrated that, after stimulation by bioactive compounds obtained from fungi, TLRs could trigger various biological processes via TLR-mediated signal pathways. These TLRs initiate a series of kinase phosphorylations and cause NF-κB activation (Akira Citation2003; Medvedev et al. Citation2007). The flow cytometry results indicated that SO treatment could inhibit the expression of TLR-4 on LPS-stimulated RAW 264.7 cells, which may be the underlying mechanism of the decreased production of pro-inflammatory cytokines and mediators in the SO-treated group. NF-κB is known for its unique role in coordinating immune and inflammatory responses (O'Sullivan et al. Citation2007). Studies have indicated that the NF-κB expression changing is likely to influence the production of pro-inflammatory cytokines (Adewoyin et al. Citation2015). The results of this study showed that the inhibited production of NF-κB and pSTAT1 also help to explain the decreased production of pro-inflammatory cytokines and mediators in the RAW 264.7 cells in the SO treatment group.
Moreover, the in vivo experiment also showed the significant anti-inflammatory effects of SO extract. By using carrageenan-induced paw oedema rat model, Vilela et al. studied the anti-inflammatory and antipyretic effects of S. oleraceus. Their results indicated the extract of S. oleraceus administered at 300 mg/kg p.o. had a stronger anti-inflammatory effect on rats. And in the present study, we analyzed the anti-inflammatory effects of SO using mouse ear oedema model. The results showed that the SO extract induced favourable anti-inflammatory effects (decreasing xylene-induced mouse ear oedema) through oral administration of the 250 mg/kg concentration. It functioned as well as the acetylsalicylic acid (100 mg/kg) and had less effect on the weights of the mice.
Li et al. (Citation2008) showed that villosol, ferulaic acid, β-sitosterol, ursolic acid, rutin and β-daucosterin are the main bioactive components in S. oleraceus. In recent years, large quantities of flavonoids had been isolated from the S. oleraceus, together with other types of active principles including triterpenes, iridoids, sterols, coumarins and organic acids (Li et al. Citation2008; Yoo et al. Citation2014; Hu et al. Citation2015; Hassan et al. Citation2016; Liu et al. Citation2016). In our study, rutin (a kind of flavonoids) and β-sitosterol (a kind of sterols) are the major components of S. oleraceus. Rutin modulates multiple biological functions with anticancer, antiviral, antibacterial and anti-inflammatory activities due to its appreciable free radical-scavenging and antioxidant capacities (Hosseinzadeh & Nassiri-Asl Citation2014). As a potent anticancer nutraceutical, β-sitosterol interferes with multiple cell signalling pathways, including cell cycle, apoptosis, proliferation, metastasis and inflammation (Bin Sayeed & Ameen Citation2015).
Conclusions
In conclusion, the results of this study indicated that SO extract exerted anti-inflammatory effects in vitro and in vivo. By inhibiting the expression of TLR-4, NF-κB and pSTAT1, the SO extract decreased the production of pro-inflammatory cytokines and mediators in RAW 264.7 cells stimulated by LPS. The extract also inhibited xylene-induced mouse ear oedema. The anti-inflammatory components in SO extract may include villosol, ferulaic acid, β-sitosterol, ursolic acid and rutin, and these extract will be further developed as novel pharmacological agents against inflammatory diseases.
Acknowledgements
The authors are thankful to the Desert Plants & Ecological Civilization Research Center of Northwest University for providing the research plant material and the Plant Identification Report.
Disclosure statement
The authors declare no conflict of interest.
Additional information
Funding
References
- Adewoyin M, Mohsin SM, Arulselvan P, Hussein MZ, Fakurazi S. 2015. Enhanced anti-inflammatory potential of cinnamate-zinc layered hydroxide in lipopolysaccharide-stimulated RAW 264.7 macrophages. Drug Design Devel Ther. 9:2475–2484.
- Akira S. 2003. Mammalian Toll-like receptors. Curr Opin Immunol.15:5–11.
- Angus DC, Linde-Zwirble WT, Lidicker J, Clermont G, Carcillo J, Pinsky MR. 2001. Epidemiology of severe sepsis in the United States: analysis of incidence, outcome, and associated costs of care. Crit Care Med. 29:1303–1310.
- Beutler B, Cerami A. 1988. The common mediator of shock, cachexia, and tumor necrosis. Adv Immunol. 42:213–231.
- Bin Sayeed MS, Ameen SS. 2015. beta-Sitosterol: a promising but orphan nutraceutical to fight against cancer. Nutr Cancer. 67:1214–1220.
- Bogdan C. 2001. Nitric oxide and the immune response. Nat Immunol. 2:907–916.
- Cardoso Vilela F, Soncini R, Giusti-Paiva A. 2009 . Anxiolytic-like effect of Sonchus oleraceus L. in mice. J Ethnopharmacol. 124:325–327.
- Doufas AG, Tian L, Padrez KA, Suwanprathes P, Cardell JA, Maecker HT, Panousis P. 2013. Experimental pain and opioid analgesia in volunteers at high risk for obstructive sleep apnea. PloS One. 8:e54807.
- Ferrucci L, Ble A, Bandinelli S, Lauretani F, Suthers K, Guralnik JM. 2004. A flame burning within. Aging Clin Exp Res. 16:240–243.
- Funk CD. 2001. Prostaglandins and leukotrienes: advances in eicosanoid biology. Science. 294:1871–1875.
- Hassan EM, Matloub AA, Aboutabl ME, Ibrahim NA, Mohamed SM. 2016. Assessment of anti-inflammatory, antinociceptive, immunomodulatory, and antioxidant activities of Cajanus cajan L. seeds cultivated in Egypt and its phytochemical composition. Pharm Biol. 54:1380–1391.
- Han YF, Zhang Q, Gao K, Jia ZJ. 2005. New sesquiterpenes from Sonchus transcaspicus. Planta Med. 71:543–547.
- Hu N, Kong L, Qian A, Meng Q, Li C, Yu X, Chen H, Du X, Li X. 2015. HMGB1 Silencing potentiates the anti-inflammatory effects of sodium ferulate in ox-LDL-stimulated vascular smooth muscle cells. Cell Biochem Biophys. 72:297–304.
- Lee KW, Bode AM, Dong Z. 2011. Molecular targets of phytochemicals for cancer prevention. Nat Rev Cancer. 11:211–218.
- Li N. 2006. Studies on chemical constituents and anti-inflammation effect and extract and purifying technique of total saponins of white Patrinia [dissertation]. Chengdu: University of TCM.
- Li N, Zhao B, Yu YF, Dong XP. 2008. Studies on anti-inflammation chemical constitutes of Patrinia villosa. J Chinese Med Mater. 31:51–53.
- Liu B, Piao X, Guo L, Liu S, Chai F, Gao L. 2016 . Ursolic acid protects against ulcerative colitis via anti-inflammatory and antioxidant effects in mice. Mol Med Rep. 13:4779–4785.
- Livak KJ, Schmittgen TD. 2001. Analysis of relative gene expression data using real-time quantitative PCR and the 2(-Delta Delta C(T)) method. Methods. 25:402–408.
- McDowell A, Thompson S, Stark M, Ou ZQ, Gould KS. 2011. Antioxidant activity of puha (Sonchus oleraceus L.) as assessed by the cellular antioxidant activity (CAA) assay. Phytother Res PTR. 25:1876–1882.
- Medvedev AE, Piao W, Shoenfelt J, Rhee SH, Chen H, Basu S, Wahl LM, Fenton MJ, Vogel SN. 2007. Role of TLR4 tyrosine phosphorylation in signal transduction and endotoxin tolerance. J Biol Chem. 282:16042–16053.
- Nathan C. 2002. Points of control in inflammation. Nature. 420:846–852.
- Hosseinzadeh H, Nassiri-Asl M. 2014. Review of the protective effects of rutin on the metabolic function as an important dietary flavonoid. J Endocrinol Invest. 37:783–788.
- O'Sullivan B, Thompson A, Thomas R. 2007. NF-kappa B as a therapeutic target in autoimmune disease. Expert Opin Ther Targets. 11:111–122.
- Ou ZQ, Rades T, McDowell A. 2015 . Anti-ageing effects of Sonchus oleraceus L. (pūhā) leaf extracts on H2O2-induced cell senescence. Molecules. 20:4548–4564.
- Raetz CR, Whitfield C. 2002. Lipopolysaccharide endotoxins. Annu Rev Biochem. 71:635–700.
- Saraiva RA, Araruna MK, Oliveira RC, Menezes KD, Leite GO, Kerntopf MR, Costa JG, Rocha JB, Tome AR, Campos AR, et al. 2011. Topical anti-inflammatory effect of Caryocar coriaceum Wittm. (Caryocaraceae) fruit pulp fixed oil on mice ear edema induced by different irritant agents. J Ethnopharmacol. 136:504–510.
- Vasto S, Candore G, Balistreri CR, Caruso M, Colonna-Romano G, Grimaldi MP, Listi F, Nuzzo D, Lio D, Caruso C. 2007. Inflammatory networks in ageing, age-related diseases and longevity. Mech Ageing Dev. 128:83–91.
- Vilela FC, Bitencourt AD, Cabral LD, Franqui LS, Soncini R, Giusti-Paiva A. 2010. Anti-inflammatory and antipyretic effects of Sonchus oleraceus in rats. J Ethnopharmacol. 127:737–741.
- Vilela FC, de Mesquita Padilha M, Dos Santos ESL, Alves-da-Silva G, Giusti-Paiva A. 2009. Evaluation of the antinociceptive activity of extracts of Sonchus oleraceus L. in mice. J Ethnopharmacol. 124:306–310.
- Volynskii Iu D, Berdikian S, Pastushenko VP, Nikitaev NS, Nagiev AA. 1976. Utilization of the thermodilution method for study and assessment of rapid changes in central hemodynamics under clinical conditions. Kardiologiia. 16:94–98.
- Woods JA, Vieira VJ, Keylock KT. 2009. Exercise, inflammation, and innate immunity. Immunol Allergy Clin North Am. 29:381–393.
- Xia DZ, Yu XF, Zhu ZY, Zou ZD. 2011. Antioxidant and antibacterial activity of six edible wild plants (Sonchus spp.) in China. Nat Prod Res. 25:1893–1901.
- Yayeh T, Jung KH, Jeong HY, Park JH, Song YB, Kwak YS, Kang HS, Cho JY, Kim SK, et al. 2012. Korean red ginseng saponin fraction downregulates proinflammatory mediators in LPS stimulated RAW264.7 cells and protects mice against endotoxic shock. J Ginseng Res. 36:263–269.
- Yin J, Kwon GJ, Wang MH. 2007. The antioxidant and cytotoxic activities of Sonchus oleraceus L. extracts. Nutr Res Pract. 1:189–194.
- Yoo H, Ku SK, Baek YD, Bae JS. 2014. Anti-inflammatory effects of rutin on HMGB1-induced inflammatory responses in vitro and in vivo. Inflamm Res. 63:197–206.
- Zhang JM, An J. 2007. Cytokines, inflammation, and pain. Int Anesthesiol Clin. 45:27–37.