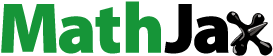
Abstract
Context: Alchornea laxiflora (Benth.) Pax. & Hoffman (Euphorbiaceae) root decoctions are traditionally used in the treatment of malaria and pain in Nigeria.
Objective: To assess the antimalarial, antiplasmodial and analgesic potentials of root extract and fractions against malarial infections and chemically-induced pains.
Material and methods: The root extract and fractions of Alchornea laxiflora were investigated for antimalarial activity against Plasmodium berghei infection in mice, antiplasmodial activity against chloroquine sensitive (Pf 3D7) and resistant (Pf INDO) strains of Plasmodium falciparum using SYBR green assay method and analgesic activity against experimentally-induced pain models. Acute toxicity study of the extract, cytotoxic activity against HeLa cells and GCMS analysis of the active fraction were carried out.
Results: The root extract (75–225 mg/kg, p.o.) with LD50 of 748.33 mg/kg exerted significant (p < 0.05–0.001) antimalarial activity against P. berghei infection in suppressive, prophylactive and curative tests. The root extract and fractions also exerted moderate activity against chloroquine sensitive (Pf 3D7) and resistant (Pf INDO) strains of P. falciparum with the ethyl acetate fraction exerting the highest activity with IC50 value of 38.44 ± 0.89 μg/mL (Pf 3D7) and 40.17 ± 0.78 μg/mL (Pf INDO). The crude extract was not cytotoxic to HeLa cells with LC50 value >100 μg/mL. The crude extract and ethyl acetate fraction exerted significant (p < 0.05–0.001) analgesic activity in all pain models used.
Discussion and conclusions: These results suggest that the root extract/fractions of A. laxiflora possess antimalarial, antiplasmodial and analgesic potentials and these justify its use in ethnomedicine to treat malaria and pain.
Introduction
Alchornea laxiflora (Benth.) Pax. & Hoffman (Euphorbiaceae) is a deciduous shrub, about 6–10 m high. It grows in most areas of Africa including Nigeria, DR Congo, Ethiopia and throughout East Africa to Zimbabwe (Burkill Citation1994). Alchornea laxiflora is called ‘Opoto and Nwariwa’ respectively among the Yoruba and Ibibio tribes in Nigeria. The root decoctions are used by the Ibibios to treat malaria while leaf infusion is used in folklore medicine by the Yorubas as malarial remedy (Adeloye et al. Citation2005). The leave decoctions are usually used to treat inflammation and infectious diseases (Ogundipe et al. Citation2001). Oladunmoye and Kehinde (Citation2011) reported the use of A. laxiflora among the Yoruba tribe of southwestern Nigeria for the treatment of poliomyelitis, and measles. Biological activities reported on the leaf include; antioxidant (Farombi et al. Citation2003; Oloyede et al. Citation2010), antibacterial and antifungal activities against Staphylococcus aureus, Escherichia coli, Bacillus subtilis, Pseudomonas aeruginosa, and fungal species (Oloyede et al. Citation2010; Akinpelu et al. Citation2015), hepatoprotective activity (Oloyede et al. Citation2011), antianaemic activity (Oladiji et al. Citation2014), antitoxicity, anticonvulsant and sedative effects (Esosa et al. Citation2013). The leaf extract of Alchornea laxiflora has been reported to contain alkaloids, saponins, tannins, phlobatannins, flavonoids and cardiac glycosides, among others (Oloyede et al. Citation2010; Oladiji et al. Citation2014). Flavonoids such as quercetin, quercetrin, rutin, taxifolin and quercetin 3, 4-diacetate (Ogundipe et al. Citation2001; Adeloye et al. Citation2005; Oloyede et al. Citation2011), quercetin-3-O-I2-d-glucopyranoside and quercetin 3, 7, 3′, 4′-tetrasulphate (Oloyede et al. Citation2011) have been isolated from the ethyl acetate leaf fraction of the plant. Terpenoid compounds were reported to be present in the root extract of A. laxiflora (Farombi et al. Citation2003).
Scientific information regarding the antiplasmodial activity of A. laxiflora root is not available. In this investigation, we report the analgesic, in vivo antimalarial and in vitro antiplasmodial activities of the ethanol root extract and fractions of Alchornea laxiflora against Plasmodium berghei and chloroquine sensitive and resistant strains of Plasmodium falciparum to confirm the folkloric claim of its usefulness in the treatment of malaria traditionally.
Materials and methods
Drugs
Chloroquine diphosphate and artemisinin used in this study were from Sigma-Aldrich, Darmstadt, Germany.
Animals
The animals (Swiss albino mice) of either sex were used for these experiments. Four-week-old mice (16–20 g) were used for malaria study, while 2-month-old mice (25–28 g) were used in the analgesic study. The animals were housed in standard cages and were maintained on a standard pelleted feed (Guinea Feed) and water ad libitum. Permission and approval for animal studies were obtained from the College of Health Sciences Animal Ethics Committee, University of Uyo.
Parasite
A chloroquine sensitive strain of Plasmodium berghei (ANKA) was obtained from the National Institute of Medical Research (NIMR), Yaba Lagos, Nigeria and was maintained by sub-passage in mice while Plasmodium falciparum strains Pf 3D7 and Pf INDO were obtained from the International Center for Genetic Engineering and Biotechnology, New Delhi, India.
Collection of plant materials
The fresh roots of Alchornea laxiflora were collected in August 2015 from a farmland in Nung Oku in Uruan LGA, Akwa Ibom State, Nigeria. The roots were identified and authenticated as Alchornea laxiflora by Dr. Margaret Bassey, a taxonomist in the Department of Botany and Ecological studies, University of Uyo, Uyo. Nigeria. Herbarium Specimen (FPHUU 536) was deposited at the Faculty of Pharmacy Herbarium, University of Uyo, Uyo.
Extraction
The plant parts (root) were cut into smaller pieces, washed and air-dried on laboratory table for 2 weeks. The dried roots were pulverized using a pestle and mortar. The powdered root was macerated in 95% ethanol for 72 h. The liquid ethanol extract obtained by filtration was evaporated to dryness in a rotary evaporator 40 °C. The crude ethanol extract (20 g) was further partitioned successively with 2 L each of petroleum ether, dichloromethane, ethyl acetate and butanol to give the corresponding fractions of these solvents. The extract/fractions were stored in a refrigerator at 4 °C until used for experiment reported in this study.
Phytochemical screening
Phytochemical screening of the crude root extract was carried out by employing standard procedures and tests (Trease & Evans Citation1989; Sofowora Citation1993), to reveal the presence of chemical constituents such as alkaloids, flavonoids, tannins, terpenes, saponins, anthraquinones, reducing sugars, cardiac glycosides and phlobatannins.
Determination of acute toxicity in mice
This was done by determining the median lethal dose (LD50) of the extract using the method of Lorke’s (Citation1983). This involved intraperitoneal (i.p.) administration of different doses of the extract (100–1000 mg/kg) to groups of five mice each. The animals were observed for manifestation of physical signs of toxicity such as writhing, decreased motor activity, decreased body/limb tone, decreased respiration and death. The number of deaths in each group within 24 h was recorded.
Parasite inoculation
Each mouse used in the experiment was inoculated i.p. with 0.2 mL of infected blood containing about 1 × 107 P. berghei parasitized erythrocytes. The inoculum consisted of 5 × 107 P. berghei erythrocytes per mL. This was prepared by determining both the percentage parasitaemia and the erythrocytes count of the donor mouse and diluting the blood with isotonic saline in proportions indicated by both determinations (Odetola & Basir Citation1980).
Drug administration
The drugs (chloroquine and pyrimethamine) and extract used in the antimalarial study were orally administered with the aid of a stainless metallic feeding cannula.
Evaluation of in vivo antimalarial activity of ethanol crude root extract of Alchornea laxiflora
Evaluation of suppressive activity of the extract (four-day test)
Evaluation of the schizontocidal activity of the extract/fractions and chloroquine against early Plasmodium berghei infection in mice was done according to method described previously by Knight and Peters (Citation1980). Forty-eight mice were infected with the parasite on the first day and randomly divided into eight groups of six mice each. The mice in group 1 were administered with the 75 mg/kg, the group 2, 150 mg/kg and group 3, 225 mg/kg of crude extract, while groups 4, 5 and 6 were respectively administered with 150 mg/kg of dichloromethane, ethyl acetate and n-butanol fractions. Group 7 was administered 5 mg/kg of chloroquine (positive control), and 10 mL/kg of distilled water to group 8 (negative control) for four consecutive days (D0–D3) between 8 am and 9 am. On the fifth day (D4), thin blood film was made from tail blood of each mouse and stained with Giemsa stain to reveal parasitized erythrocytes out of 500 in a random field of the microscope. The average percentage suppression of parasitaemia was calculated in comparison with the controls as follows:
The mean survival time (MST) of the mice was monitored in the different groups for 30 days.
Evaluation of prophylactic or repository activities of extract
The repository activity of the extract/fractions and pyrimethamine was assessed by using the method described by Peters (Citation1965). Forty-eight mice were randomly divided into eight groups of six mice each. Groups 1–3 were administered with 75, 150 and 225 mg/kg/day of the extract, respectively. Groups 4, 5 and 6 were respectively administered with 150 mg/kg of dichloromethane, ethyl acetate or n-butanol. Groups 7 and 8 were administered with 1.2 mg/kg/day of pyrimethamine (positive control), and 10 mL/kg of distilled water (negative control), respectively. Administration of the extract/drug continued for three consecutive days (D0–D2). On the fourth day (D3), the mice were inoculated with P. berghei berghei. The parasitaemia level was assessed by blood smears 72 h later. The MST of the mice in each treatment group was determined over a period of 30 days.
Evaluation of curative activities of extract (Rane’s test)
Evaluation of the schizontocidal activity of the extract/fractions, and chloroquine in established infection was done according to the method of Ryley and Peters (Citation1970). P. berghei was injected i.p. into 48 mice on the first day (D0). Seventy-two hours later (D3), infections were confirmed in the infected mice from Giemsa stained thin blood film made from tail blood of the mice and the parasitaemia level of each mouse determined. The mice were then divided randomly into eight groups of six mice each. Specific doses of the extract, 75, 150 and 225 mg/kg were orally administered respectively to mice in groups 1–3. Groups 4, 5 and 6 were respectively administered with 150 mg/kg of dichloromethane, ethyl acetate or n-butanol fractions. 5 mg/kg/day of chloroquine was administered to the group 7 (positive control) and group 8 was given 10 mL/kg of distilled water (negative control). The extract/fractions and drugs were administered once daily for five days. Giemsa stained thin smears were prepared from tail blood samples collected on each day of treatment to monitor parasitaemia level. The MST of the mice in each treatment group was determined over a period of 29 days (D0–D28).
Evaluation of in vitro antiplasmodial activity
In vitro cultivation of plasmodium falciparum
CQ-sensitive strain 3D7 and CQ-resistant strain INDO of Plasmodium falciparum used in this study were in vitro blood stage cultures to test the antimalarial efficacy of the crude root extract and fractions. The culture was maintained at the Malaria Research Laboratory, International Center for Genetic Engineering and Biotechnology, New Delhi, India. Plasmodium falciparum culture was maintained according to the method described by Trager and Jensen (Citation1976) with slight modifications. Plasmodium falciparum (3D7) cultures were maintained in fresh O + ve human erythrocytes suspended at 4% haematocrit in RPMI 1640 (Sigma, Darmstadt, Germany) containing 0.2% sodium bicarbonate, 0.5% albumax, 45 μg/L hypoxanthine and 50 μg/L gentamicin and incubated at 37 °C under a gas mixture of 5% O2, 5% CO2 and 90% N2. Daily, infected erythrocytes were transferred into fresh complete medium to propagate the culture. For Plasmodium falciparum (INDO strain) in culture medium, albumax was replaced by 10% pooled human serum.
Drug dilutions
Dimethyl sulphoxide (DMSO) was used to prepare the stock solutions of each plant extract and fraction as well as artemisinin, while water (Milli-Q grade) was used in the case of CQ stock solution. Culture medium was used to dilute the stock solutions to their required concentrations except CQ. The final solution of each stock was constituted to contain nontoxic concentration of DMSO (0.4%), which was found to be harmless to the parasite. Drugs, test plant extracts and fractions were then placed in 96-well flat bottom tissue culture grade plates.
In vitro antiplasmodial assays
The crude root extract and fractions of this plant were evaluated for their antiplasmodial activity against 3D7 and INDO strains of Plasmodium falciparum. For drug screening, SYBR green I-based fluorescence assay was set up as described previously (Smilkstein et al. Citation2004). Sorbitol synchronized parasites were incubated under normal culture conditions at 2% haematocrit and 1% parasitaemia in the absence or presence of increasing concentrations of plant extract and fractions. CQ and artemisinin were used as positive controls, while 0.4% DMSO was used as the negative control. After 48 h of incubation, 100 μL of SYBR Green I solution (0.2 μL of 10,000 × SYBR Green I (Invitrogen)/mL) in lysis buffer[Tris (20 mM; pH 7.5), EDTA (5 mM), saponin (0.008%, w/v) and Triton X-100 (0.08%, v/v)] was added to each well and mixed twice gently with multi-channel pipette and incubated in dark at 37 °C for 1 h. Fluorescence was measured with a Victor fluorescence multi-well plate reader (Perkin Elmer, Waltham, MA) with excitation and emission wavelength bands centred at 485 and 530 nm, respectively. The fluorescence counts were plotted against the drug concentration and the 50% inhibitory concentration (IC50) was determined by analysis of dose–response curves and IC50 estimator. Results were validated microscopically by examination of Giemsa stained smears of extract treated parasite cultures.
Cytotoxic activity on HeLa cells using MTT assay
The cytotoxic effects of extract and fractions on host cells were assessed by functional assay as described (Mosmann Citation1983) using HeLa cells cultured in RPMI containing 10% foetal bovine serum, 0.21% sodium bicarbonate (Sigma, Darmstadt, Germany) and 50 μg/mL gentamycin (complete medium). Briefly, cells (104 cells/200 μL/well) were seeded into 96-well flat-bottom tissue culture plates in complete medium. Drug solutions were added after 24 h of seeding and incubated for 48 h in a humidified atmosphere at 37 °C and 5% CO2. DMSO (as positive inhibitor) was added at 10%. A stock solution (20 μL) of MTT (5 mg/mL in 1 × phosphate buffered saline) was added to each well, gently mixed and incubated for another 4 h. After spinning the plate at 1500 rpm for 5 min, supernatant was removed and 100 μL of DMSO (stop agent) was added. Formation of formazon was read on a microtiter plate reader (Versa max tunable multi-well plate reader) at 570 nm. The 50% cytotoxic concentration (IC50) of drug was determined by analysis of dose–response curves and IC50 estimator.
Evaluation of analgesic potential of the extract
Acetic acid induced writhing in mice
Intraperitoneal injection of 3% acetic acid was used to induce writhings (abdominal constrictions consisting of the contraction of abdominal muscles together with the stretching of hindlimbs) according to the procedure described by Santos et al. (Citation1994), Correa et al. (Citation1996) and Nwafor et al. (Citation2010). The animals were divided into eight groups of six mice each. Group 1 served as negative control and received 10 mL/kg of normal saline, while groups 2, 3 and 4 were pretreated with 75, 150 and 225 mg/kg doses of A. laxiflora root extract i.p., and groups 5, 6 and 7 were respectively administered 150 mg/kg of dichloromethane, ethyl acetate and n-butanol fractions. Group 8 received 100 mg/kg of acetyl salicylic acid (ASA). After 30 min, 0.2 mL of 2% acetic acid was administered i.p. The number of writhing movements was counted for 30 min. Antinociception (analgesia) was expressed as the reduction of the number of abdominal constrictions between control animals and mice pretreated with extracts.
Formalin-induced hind paw licking in mice
The procedure adopted was similar to that described by Hunskaar and Hole (Citation1987), Gorski et al., (Citation1993) and Okokon and Nwafor (Citation2010). The animals were injected with 20 μL of 2.5% formalin solution (0.9% formaldehyde) made up in phosphate buffer solution (PBS concentration: NaCl 137 mM, KCl 2.7 mM and phosphate buffer, 10 mM) under the surface of the right hind paw. The amount of time spent licking the injected paw was timed and considered as indication of pain. Adult albino mice (20–25 g) of either sex randomized into eight groups of six mice each were used for the experiment. The mice were fasted for 24 h before used but allowed access to water. The animals in group 1 (negative control) received 10 mL/kg of normal saline, groups 2–4 received 75, 150 and 225 mg/kg doses of the extract respectively, while groups 5, 6 and 7 were respectively treated with dichloromethane, ethyl acetate and n-butanol fractions. Group 8 received 100 mg/kg of ASA 30 min before being challenged with buffered formalin. The responses were measured for 5 min (first phase) and 15–30 min (second phase) after formalin injection.
Thermally induced pain in mice
The effect of extract and fractions on hot plate induced pain was investigated in adult mice. The hot plate was used to measure the response latencies according to the method of Vaz et al. (Citation1996) and Okokon and Nwafor (Citation2010). In these experiments, the hot plate was maintained at 45 ± 1 °C, each animal was placed into a glass beaker of 50 cm diameter on the heated surface, and the time(s) between placement and shaking or licking of the paws or jumping was recorded as the index of response latency. An automatic 30 s cutoff was used to prevent tissue damage. The animals were randomly divided into eight groups of six mice each and fasted for 24 h but allowed access to water. Group 1 animal served as negative control and received 10 mL/kg of normal saline. Groups 2, 3 and 4 were pretreated i.p. with 75, 150 and 225 mg/kg doses of A. laxiflora root extract respectively, while groups 5, 6 and 7 were respectively treated with 150 mg/kg of dichloromethane, ethyl acetate and n-butanol fractions. Mice in group 8 received 100 mg/kg of ASA i.p., 30 min prior to the placement on the hot plate.
Gas chromatography–mass spectrometry analysis
Quantitative and qualitative data were determined by GC and GC–MS, respectively. The fraction was injected onto a Shimadzu GC-17A system (Kyoto, Japan), equipped with an AOC-20i autosampler and a split/splitless injector. The column used was an DB-5 (Optima-5), 30 m, 0.25 mm i.d., 0.25 μm df, coated with 5% diphenyl–95% polydimethylsiloxane. The oven temperature operated was programed as follows: 50 °C, held for 1 min, rising at 3 °C/min to 250 °C, held for 5 min, rising at 2 °C/min to 280 °C, held for 3 min. The injection temperature and volume were 250 °C and 1.0 μL, respectively. Injection mode, split; split ratio was 30:1. Carrier gas was nitrogen set at 30 cm/s linear velocity and inlet pressure of 99.8 kPa. Other operating parameters used included: detector temperature, 280 °C; hydrogen flow rate, 50 mL/min; air flow rate, 400 mL/min; make-up (H2/air), flow rate, 50 mL/min and sampling rate, 40 ms. Data were acquired by means of GC solution software (Shimadzu, Kyoto, Japan). Agilent 6890N GC was interfaced with a VG Analytical 70-250s double-focusing mass spectrometer. Helium was used as the carrier gas. The MS operating conditions were: ionization voltage 70 eV, ion source 250 °C. The GC was fitted with a 30 m × 0.32 mm fused capillary silica column coated with DB-5. The GC operating parameters were identical with those of GC analysis described above.
Identification of the compounds
The identification of compounds present in the active fraction of the plants’ extract was based on direct comparison of the retention times and mass spectral data with those for standard compounds, and by computer matching with the Wiley and Nist Libraries (Adams Citation2001; Setzer et al. Citation2007).
Statistical analysis
Data obtained from this work were analysed statistically using ANOVA (One-way) followed by a post test (Tukey–Kramer’s multiple comparison test). Differences between means were considered significant at 1% and 5% level of significance, that is p ≤ 0.01 and 0.05.
Results
Phytochemical screening
Phytochemical screening of the crude root extract revealed the presence of chemical constituents such as alkaloids, flavonoids, tannins, terpenes, saponins and cardiac glycosides.
Determination of median lethal dose
The LD50 was calculated to be 748.33 mg/kg. The physical signs of toxicity included excitation, paw licking, increased respiratory rate, decreased motor activity, gasping and coma which was followed by death.
Effect on suppressive activity of ethanol root extract/fraction of Alchornea laxiflora
The extract showed a dose-dependent chemosuppressive effect on the parasitaemia. These effects were statistically significant relative to the control (p < 0.05–0.001). The chemoinhibitory ranged from 25.87 to 44.05% (). Dichloromethane fraction had the highest activity with chemosuppression of 65.73%. The crude extract exhibited a MST range of 12.75 ± 0.47 to 18.25 ± 1.03 days, while DCM fraction had MST of 18.75 ± 0.62 days. However, the effect of the extract was weak compared to that of the standard drug, chloroquine, with a chemosuppression of 74.12% and MST of 24.25 ± 1.25 days ().
Table 1. Suppressive activities of root extract of Alchornea laxiflora (four-day test).
Effect on repository activity of ethanol root extract/fractions of Alchornea laxiflora
The ethanol root extract of Alchornea laxiflora showed a dose-dependent chemosuppressive effect of 25.92–42.59% on the parasitaemia and MST range of 10.50 ± 0.50 to 18.50 ± 1.25 days during prophylactic studies. These effects were statistically significant relative to the control (p < 0.05–0.001). DCM fraction had the highest activity with chemosuppression of 55.55% and MST range of 19.25 ± 1.18 days. However, these effects were weak compared to that of the standard drug, pyrimethamine, with chemosuppression of 78.70% ().
Table 2. Repository/prophylactic activity of ethanol root extract of Alchornea laxiflora on Plasmodium berghei infection in mice.
Antiplasmodial effect of ethanol root extract of Alchornea laxiflora on established infection
The extract showed a dose-dependent schizonticidal effect on the parasitaemia. There were reductions in the percentage parasitaemia of the extract/fraction and chloroquine-treated groups compared to that of the control in which prominent increases were recorded. These reductions were statistically significant relative to the control (p < 0.0–0.001) (). The chemosuppression range of the extract treated groups was 50.0–76.52% on day 7. The crude extract also showed a significant (p < 0.05–0.001), dose-dependent MST (13.00 ± 0.40 to 19.00 ± 0.57 days) on established infection, and the MST value of dichloromethane fraction treated group was 19.25 ± 0.47 days. The highest dose of the extract as well as dichloromethane fraction (225 mg/kg) produced a chemosuppressive effects that were comparable to that of the standard drug, chloroquine ().
Table 3. Antiplasmodial activity of root extract of Alchornea laxiflora (curative test).
In vitro antiplasmodial and cytotoxic activities
The results of the in vitro studies show that the root extract and fractions exerted antiplasmodial activity against chloroquine sensitive Pf 3D7 and resistant Pf INDO strains of P. falciparum (). The ethyl acetate fraction was found to exhibit moderate activity against both strains of P. falciparum with IC50 value of 38.44 ± 0.89 μg/mL (Pf 3D7) and 40.17 ± 0.78 μg/mL (Pf INDO). The potency order was ethyl acetate > crude extract > dichloromethane > petroleum ether. The crude extract and fractions were not cytotoxic to HeLa cell lines tested with TC50 values >100 μg/mL.
Table 4. In vitro antiplasmodial activities of crude root extract and fractions of A. laxiflora.
Effect of ethanol root extract of A. laxiflora on acetic acid-induced writhing in mice
The administration of A. laxiflora extract (75, 150 and 225 mg/kg) demonstrated a dose-dependent reduction in acetic acid-induced writhing in mice. The reductions were statistically significant (p < 0.05–0.001) relative to control during the first 20 min of the experiment. The dichloromethane and ethyl acetate fractions exerted activities that were comparable to that of the standard drug, ASA ().
Effect of ethanol root extract of A. laxiflora on formalin induced paw licking in mice
The administration of A. laxiflora extract (75, 150 and 225 mg/kg) demonstrated a dose-dependent reduction in formalin-induced hind paw licking in mice. The reductions were statistically significant (p < 0.05–0.001) relative to control and were persistent from 10 to 25 min of the experiment. The ethyl acetate fraction exerted the highest activity which was comparable to that of the standard drug, ASA, 100 mg/kg. The effect of the crude extract was diminished in the last 5 min of the 30 min duration of the experiment ().
Table 5. Effect of Alchornea laxiflora root extract on acetic acid induced writhing in mice.
Effect of ethanol root extract of A. laxiflora on thermally-induced pain in mice
The extract (75, 150 and 225 mg/kg) exhibited a dose-dependent effect on thermally-induced pain in mice. These inhibitions were statistically significant (p < 0.05–0.001) relative to the control. The ethyl acetate fraction exerted the highest activity which was weak compared to that of the standard drug, ASA (100 mg/kg) ().
Table 6. Effect of Alchornea laxiflora root extract on formalin hind paw licking in mice.
GCMS analysis
The GCMS analysis of the ethyl acetate fraction of A. laxiflora root revealed the presence of 43 bioactive compounds with major and minor ones as represented in .
Table 7. Effect of Alchornea laxiflora root extract on hot plate test.
Table 8. GCMS profile of ethyl acetate fraction of Alchornea laxiflora root.
Discussion
The root of A. laxiflora is used in Ibibio folkloric medicine as malaria remedy and this work was designed to confirm and authentic its antiplasmodial potential in order to provide scientific basis for its usage as antimalarial plant.
In this work, LD50 was determined to be 748.33 mg/kg, portraying the extract to be slightly toxic (Homburger Citation1989) and the doses (75, 150 and 300 mg/kg) employed in this study were relatively safe.
The antiplasmodial activity of root extract and fractions of Alchornea laxiflora was investigated for antimalarial activity against rodent malaria parasite, P. berghei, infection in mice using standard in vivo models. It was found that the extract significantly reduced the parasitaemia in prophylactic, suppressive and curative models in a dose-dependent fashion and the dichloromethane and ethyl acetate fractions were found to demonstrate considerable activities confirming the antimalarial potential of this extract. The extract and fractions also prolonged the MST of the mice suggesting that they were able to offer certain degree of protection to the mice. This activity could have resulted from plasmodicidal or plasmodistatic activity of the extract and fractions. These results validate the use of the root decoctions as malarial remedy.
Further work was carried out to evaluate the activities of the extract and fractions against human malaria parasite, P. falciparum. The in vitro antiplasmodial study on the root extract and fractions of A. laxiflora carried out against chloroquine sensitive (Pf 3D7) and resistant (Pf INDO) strains of human malaria parasite, P. falciparum, further showed that the root extract and fractions possess moderate antiplasmodial activity against the parasites. Ethyl acetate fraction was found to exert the most pronounced activity probably suggesting the localization of the active compounds in this fraction. The root extract and fractions were observed to be active also against the chloroquine resistant strain (Pf INDO). This suggests that the root extract could be effective in the treatment of resistant malaria infection. However, dichloromethane fraction was outstandingly more active during the in vivo testing as compared to in vitro activity. This suggests the involvement of immunostimulating activity which may be due to the phytochemical constituents in this fraction. Tannins, squalene and fatty acids such as linoleic acids have been reported to possess immune-stimulating properties (Kolodziej et al. Citation2001; Chakrabarti et al. Citation2012; Kumaradevan et al. Citation2015). These compounds are found to be present in this fraction and extract may be responsible for the suggested immunostimulatory effect.
The phytochemical screening and GCMS analysis of the crude extract and ethyl acetate fraction have revealed the present of some pharmacologically active compounds such as flavonoids, alkaloids, terpenes, triterpenes like squalene, tannins, phenolics and polyunsaturated fatty acids (PUFAs) among others. These compounds are likely to be responsible for the observed activities of the extract and fractions. Some secondary metabolites of plants such as alkaloids, flavonoids and triterpenoids have been reported previously to have antiplasmodial properties (Kirby et al. Citation1989; Philipson & Wright Citation1991; Christensen & Kharazmi Citation2001).
Polyunsaturated fatty acids such as hexadecanoic acid, methyl ester, 9,12-octadecadienoic acid methyl ester (linoleic acid), 9,12,15-octadecatrienoic acid, methyl ester (linoleic acid), 9-octadecenoic acid (Z)-2-hydroxyethyl ester and eicosanoic acid, 2-(acetyloxy)-1-[(acetyloxy)methyl]ethyl ester have been found in the active antiplasmodial fraction. These PUFAs above have been implicated in antiplasmodial activity and this activity has been reported to increase with the degree of unsaturation (Kumaratilake et al. Citation1992; Krugliak et al. Citation1995; Suksamrarn et al. Citation2005; Attioua et al. Citation2007; Melariri et al. Citation2011, Citation2012). Flavonoids such as quercetin, quercetrin, rutin, taxifolin and quercetin 3,4-diacetate have also been isolated from ethyl acetate leaf fraction of A. laxiflora (Ogundipe et al. Citation2001; Adeloye et al. Citation2005). These compounds are likely to be present in the root probably in varying quantity. Quercetin and its derivatives as well as rutin have been shown to possess significant antiplasmodial activity against chloroquine sensitive and resistant strains of P. falciparum (Attioua et al. Citation2011; Ganesh et al. Citation2012; Ezenyi et al. Citation2014). Rutin has been reported to possess IC50 of 3.53 ± 13.34 μM against 3D7 and 15.00 μM against K1 (Attioua et al. Citation2011). Farombi et al. (Citation2003) reported the presence of terpenoids compounds in the root and GCMS analysis of ethyl acetate fraction further revealed the presence of squalene, a triterpene and active antioxidant compound (Kohno et al. Citation1995). These compounds mentioned above to be present in the extract and active fraction maybe responsible for the observed antiplasmodial activities. Antioxidant property of quercetin has been suggested to be responsible for its antiplasmodial activity (Cimanga et al. Citation2009; Ganesh et al. Citation2012), as elevated free radical levels are common features of malaria disease and are implicated in severe malaria complications. This could be one of the mechanisms of action of this extract. Other mechanisms of antiplasmodial activity have been proposed for flavonoids besides the antioxidant activity. Flavonoids are known to exert antiplasmodial activity by chelating with nucleic acid base pairing of the parasite (Lui et al. Citation1992), thereby producing plasmodicidal effect. Other modes of action include modulation of host immunity to tackle disease and inhibition of plasmodial enoyl-ACP reductase (FAB I enzyme) – a key regulator of type II fatty synthases (FAS-II) in P. falciparum (Kirmizibekmez et al. Citation2004; Teffo et al. Citation2010). Flavonoids may also bind parasite’s serinethreonine kinase with high affinity and affect its development (Ferreira et al. Citation2010). The root extract may be acting through one of these mechanisms.
These compounds (flavonoids) present in this plant extract and in particular, ethyl acetate fraction may in part have contributed to the plasmodicidal activity of this extract/fraction and therefore explained the mechanism of antiplasmodial effect of the extract.
Phytochemical compounds such as terpenes and their derivatives as well as alkaloids which have been found to be present in this plant, have been reported previously to contribute to antiplasmodial activity of many plants (Philipson & Wright Citation1991; Christensen & Kharazmi Citation2001). These could have also contributed to the antiplasmodial activity of this extract.
The root extract and fractions were found to possess analgesic activity against acetic acid-induced writhing, formalin induced hind paw licking and thermal induced pains with the ethyl acetate and chloroform fractions exhibiting prominent activity.
Acetic acid causes inflammatory pain by inducing capillary permeability (Amico-Roxas et al. Citation1984; Nwafor et al. Citation2007), and in part through local peritoneal receptors from peritoneal fluid concentration of PGE2 and PGF2α (Deraedt et al. Citation1980; Bentley et al. Citation1983). The acetic acid-induced abdominal writhing is a visceral pain model in which the processor releases arachidonic acid via cyclooxygenase, and prostaglandin biosynthesis plays a role in the nociceptive mechanism (Franzotti et al. Citation2002). It is used to distinguish between central and peripheral pain. These results suggested that the extract may be exerting its action partly through the lipoxygenase and/or cyclooxygenase system.
The organic acid has also been suggested to induce the release of endogenous mediators indirectly, which stimulates the nociceptive neurons that are sensitive to NSAIDs and narcotics (Adzu et al. Citation2003). The inhibition of acetic acid-induced writhing by the extract at all the doses suggests antinociceptive effect which might have resulted from the inhibition of the synthesis of arachidonic acid metabolites.
Formalin-induced pains involve two different types which are in phases; neurogenic and inflammatory pains (Vaz et al. Citation1996, Citation1997) and measure both centrally and peripherally mediated activities that are characteristics of biphasic pain response. The first phase (0–5 min), named neurogenic phase resulted from chemical stimulation that provoked the release of bradykinin and substance P while the second and late phase initiated after 15–30 min of formalin injection resulted in the release of inflammatory mediators such as histamine and prostaglandin (Wibool et al. Citation2008; Yi et al. Citation2008). The injection of formalin has been reported to cause an immediate and intense increase in the spontaneous activity of C fibre afferent and evokes a distinct quantifiable behaviour indicative of pain demonstrated in paw licking by the animals (Heapy et al. Citation1987). The first phase of formalin-induced hind paw licking is selective for centrally acting analgesics such as morphine (Berken et al. Citation1991), while the late phase of formalin-induced hind paw licking is peripherally mediated. Analgesic (nociceptive) receptors mediate both the neurogenic and non-neurogenic pains (Lembeck & Holzer Citation1979). The extract ability to inhibit both phases of formalin-induced paw licking suggests its central and peripheral activities as well as its ability to inhibit bradykinins, substance P, histamine and prostaglandins which are mediators in these pains.
The study also shows that the extract significantly delayed the reaction time of thermally-induced (hot plate) test. This model is selective for centrally acting analgesics and indicates narcotic involvement (Turner Citation1995) with opioid receptors. This finding further confirms the central analgesic action of the extract.
Some terpenes, flavonoids and polyphenolic compounds have also been revealed by GCMS analysis to be present in the plant extract. Flavonoids are known anti-inflammatory compounds acting through inhibition of the cyclo-oxygenase pathway (Liang et al. Citation1999). Some flavonoids are reported to block both the cyclooxygenase and lipoxygenase pathways of the arachidonate cascade at relatively high concentrations, while at lower concentrations they only block lipoxygenase pathway (Carlo Di et al. Citation1999). Some flavonoids exert their antinociception via opioid receptor activation activity (Suh et al. Citation1996; Rajendran et al. Citation2000; Otuki et al. Citation2005). Flavonoids also exhibit inhibitory effects against phospholipase A2 and phospholipase C (Middleton et al. Citation2000), and cyclooxygenase and/or lipoxygenase pathways (Robak et al. Citation1998). One of these mechanisms could likely be the mode of analgesic action of these extract and fractions.
Triterpenes have also been implicated in analgesic activity of plants (Liu Citation1995; Krogh et al. Citation1999; Tapondjou et al. Citation2003; Maia et al. Citation2006). Ursolic acid is a selective inhibitor of cyclooxygenase-2 (Ringbom et al. Citation1998). Oleanolic acid is known to exert its analgesic action through an opioid mechanism, and possibly, a modulatory influence on vanilloiod receptors (Maia et al. Citation2006). Squalene, a triterpene and strong antioxidant found in this plant may have contributed to the observed analgesic effect.
Moreso, capsaicin and dihydrocapsaicin, alkaloids, have been revealed to be present in the ethyl acetate fraction. Capsaicin is efficacious in neuropathic pain and its analgesic activity has been reported by Jolayemi and Ojewole (Citation2014) to be effective in inhibiting acetic acid induced writhing and hot plate induced pain. Capsaicin, a transient receptor potential vanilloid 1 (TRPV1) agonist, and its metabolites are natural ligands for TRPV1 receptors, which are ion channel-type receptors found on sensory neurons. Capsaicin displays analgesic activity, potentially by inducing desensitization of TRPV1 receptors after activation (Smith & Brooks Citation2014).
The above extract has been reported to exhibit analgesic activity. The presence of these compounds (polyphenolics, flavonoids and triterpenes) in this plant may have accounted for these activities and may in part explain the mechanisms of its actions in this study.
Conclusions
The results obtained in this study indicated that the root of Alchornea laxiflora plant possesses significant antimalarial and antiplasmodial activities against chloroquine sensitive and resistant strains of P. falciparum and also has analgesic action. These findings justify and confirm the usage of this plant in the treatment of malaria and related symptoms. Further studies on the extract and ethyl acetate fraction are necessary to isolate, characterize and identify the active compound which could serve as a useful agent against resistant malaria infections.
Disclosure statement
Dr Jude Okokon is grateful to International Center for Genetic Engineering and Biotechnology (ICGEB) for the financial support for Postdoctoral fellowship and ICGEB, Delhi, India for providing research facilities.
Additional information
Funding
References
- Adams RP. 2001. Identification of essential oils by gas chromatography quadrupole mass spectrometry. Carol Stream (IL): Allured Publishing Corporation.
- Adeloye AO, Aderogba MA, Idowu TO, Obuotor EM, Ogundaini AO. 2005. Investigation of the antioxidant activity of Alchornea laxiflora (Benth) and its constituents. J Food Technol. 3:365–369.
- Adzu B, Amos S, Kapu SD, Gamaniel KS. 2003. Anti-inflammatory and anti-nociceptive effects of Sphaeranthus senegalensis. J Ethnopharmacol. 84:169–173.
- Akinpelu DA, Abioye EO, Aiyegoro OA, Akinpelu OF, Okoh AI. 2015. Evaluation of antibacterial and antifungal properties of Alchornea laxiflora (Benth.) Pax. & Hoffman. Evid Based Complement Alternat Med. doi: 10.1155/2015/684839.
- Amico-Roxas M, Caruso A, Trombadore S, Scifo R, Scapagnime U. 1984. Gangliosides antinociceptive effects in rodents. Arch Int Pharmacodyn Ther. 272:103–117.
- Attioua B, Lagnikab L, Yeoc D, Antheaumed C, Kaisere M, Wenigerf B, Lobsteinf A, Vonthron-Sénécheauf C. 2011. In vitro antiplasmodial and antileishmanial activities of flavonoids from Anogeissus leiocarpus (Combretaceae). Int J Pharmaceut Rev Res. 11:1–6.
- Attioua B, Weniger B, Chabert P. 2007. Antiplasmodial activity of constituents isolated from Croton lobatus. Pharm Biol. 45:263–266.
- Bentley GA, Newton SH, Starr J. 1983. Studies on the antinociceptive action of alpha-agonist drugs and their interactions with opioid mechanisms. Br J Pharmacol. 79:125–134.
- Berken T, Ostunes L, Lermioglu F, Ozer A. 1991. Antiinflammatory, analgesic, and antipyretic effects of an aqueous extract of Erythraea centaurium. Planta Med. 57:34–37.
- Burkill HM. 1994. The useful plants of West Tropical Africa. Volume 2: Families E-I. 2nd ed. kew, Richmond, UK: Royal Botanical Gardens.
- Carlo Di G, Mascolo N, Izzo AA, Capasso F. 1999. Flavonoids: old and new aspects of a class of natural therapeutic drugs. Life Sci. 65:337–353.
- Chakrabarti R, Srivastava PK, Kundu K, Khare RS, Shanta B. 2012. Evaluation of immunostimulatory and growth promoting effect of seed fractions of Achyranthes aspera in common carp Cyprinus carpio and identification of active constituents. Fish Shellfish Immunol. 32:839–843.
- Christensen SB, Kharazmi A. 2001. Antimalarial natural products. Isolation, characterization and biological properties. In: Tringali C, editor. Bioactive compounds from natural sources: isolation, characterization and biological properties. London: Taylor & Francis; p. 379–432.
- Cimanga RK, Tona GL, Kambu OK, Mesia GK, Muyembe JJ, Apers S. 2009. Antimalarial, antiamoebic and cytotoxic activities of some extracts and isolated constituents from the leaves of Morinda morindoides (Baker) Milne-Redh. (Rubiaceae). Rec Progr Med Plants. 25:225–242.
- Correa CR, Kyle DJ, Chakravarty S, Calixto JB. 1996. Antinociceptive profile or the pseudopeptide β2 bradykinin receptors antagonist NPC 18688 in mice. Br J Pharmacol. 117:552–556.
- Deraedt R, Jougney S, Falhout M. 1980. Release of prostaglandin E and F in an algogenic reaction and its inhibition. Eur J Pharm. 51:17–24.
- Esosa US, Omage K, Erifeta O, Georgina JS, Spencer NC. 2013. Possible reversal of sodium arsenate-induced liver toxicity by hexane leaf extract of Alchornea laxiflora. Asian J Med Sci. 5:3–8.
- Ezenyi IC, Salawu OA, Kulkarni R, Emeje M. 2014. Antiplasmodial activity-aided isolation and identification of quercetin-4′-methyl ether in Chromolaena odorata leaf fraction with high activity against chloroquine-resistant Plasmodium falciparum. Parasitol Res. 113:4415–4422.
- Farombi EO, Ogundipe OO, Uhunwagho ES, Adeyanju MA, Moody JO. 2003. Antioxidant properties of extracts from Alchornea laxiflora (Benth) Pax and Hoffman. Phytother Res. 17:713–716.
- Ferreira JF, Luthria DL, Sasaki T, Heyerick A. 2010. Flavonoids from Artemisia annua L. as antioxidants and their potential synergism with artemisinin against malaria and cancer. Molecules. 15:3135–3170.
- Franzotti EM, Santos CVF, Rodrigues HMSL, Mourao RHV, Andrade MR, Antoniolli AR. 2002. Anti-inflammatory, analgesic activity and acute toxicity of Sida cordifolia L. J Ethnopharmacol. 72:273–278.
- Ganesh D, Fuehrer H, Starzengrüber P, Swoboda P, Khan WA, Reismann JA, Mueller MS, Chiba P, Noedl H. 2012. Antiplasmodial activity of flavonol quercetin and its analogues in Plasmodium falciparum: evidence from clinical isolates in Bangladesh and standardized parasite clones. Parasitol Res. 110:2289–2295.
- Gorski F, Correa CR, Filhe VC, Yunes RA, Calixto JB. 1993. Potent antinociceptive activity of a hydroalcoholic extract of Phyllanthus corcovadensis. J Pharm Pharmacol. 45:1046–1049.
- Heapy CG, Jamieson A, Russell NJW. 1987. Afferent C-fiber and A-delta activity in models of inflammation. Br J Pharmacol. 90:164.
- Homburger F. 1989. In vivo testing in the study of toxicity and safety evaluation. In: Marquis JK, editor. A guide to general toxicology. 2nd ed. New York: Karger.
- Hunskaar S, Hole K. 1987. The formalin test in mice: dissociation between inflammatory and non-inflammatory pain. Pain. 30:103–114.
- Jolayemi AT, Ojewole JA. 2014. Analgesic effects of Capsicum frutescens Linn. (Solanaceae) fruit aqueous extract in mice. Global Adv Res J Med Med Sci. 3:325–330.
- Kirby GC, O’Neill MJ, Phillipson JD, Warhurst DC. 1989. In vitro studies on the mode of action of quassinoids with activity against chloroquine-resistant Plasmodium falciparum. Biochem Pharmacol. 38:4367–4374.
- Kirmizibekmez H, Calis I, Perozzo R, Brun R, Donmez AA, Linden A, Ruedi P, Tasdemir D. 2004. Inhibiting activities of the secondary metabolites of Phlomis brunneogaleata against parasitic protozoa and plasmodial enoyl-ACP reductase, a crucial enzyme in fatty acid biosynthesis. Planta Med. 70:711.
- Knight DJ, Peters W. 1980. The antimalarial activity of N-benzyloxydihydrotriazines. I. The activity of clociguanil (BRL 50216) against rodent malaria, and studies on its mode of action. Ann Trop Med Parasitol. 74:393–404.
- Kohno Y, Egawa Y, Itoh S, Nagaoka S, Takahashi M, Mukai K. 1995. Kinetic study of quenching reaction of singlet oxygen and scavenging reaction of free radical by squalene in n-butanol. Biochim Biophys Acta. 1256:52–56.
- Kolodziej H, Kayser O, Kiderlen AF, Ito H, Hatano T, Yoshida T, Foo LY. 2001. Antileishmanial activity of hydrolyzable tannins and their modulatory effects on nitric oxide and tumour necrosis factor-alpha release in macrophages in vitro. Planta Med. 67:825–832.
- Krogh R, Kroth R, Berti C, Madereira AO, Souza MM, Cechinel-Filho V, Delle-Monache F, Yunes RA. 1999. Isolation and identification of compounds with antinociceptive action from Ipomoea pes-caprae (L.) R. Br. Pharmazie. 54:464–466.
- Krugliak M, Deharo E, Shalmiev G, Sauvain M, Moretti C, Ginsburg H. 1995. Antimalarial effects of C18 fatty acids on Plasmodium falciparum in culture and on Plasmodium vinckei petteri and Plasmodium yoelii nigeriensis in vivo. Expl Parasitol. 81:97–105.
- Kumaradevan G, Damodaran R, Mani P, Dineshkumar G, Jayaseelan T. 2015. Phytochemical screening and GC–MS analysis of bioactive components of ethanol leaves extract of Clerodendrum phlomidis (L.). Am J Biol Pharm Res. 2:142–148.
- Kumaratilake LM, Robinson BS, Ferrante A, Poulos A. 1992. Antimalarial properties of n-3 and n-6 polyunsaturated fatty acids: in vitro effects on Plasmodium falciparum and in vivo effects on P. berghei. J Clin Investig. 89:961–967.
- Lembeck F, Holzer P. 1979. Substance P as neurogenic mediator of antidromic, vasodilatation and neurogenic plasma extravasation. Naunyn-Schmiedeberg’s Arch Pharm. 310:175–183.
- Liang YC, Huang YT, Tsau SH, Lin-Shiau SY, Chen CF, Lin JK. 1999. Suppression of inducible cyclo-oxygenase and inducible nitric acid synthase by apigenia and related flavonoid in mouse macrophages. Carcinogenesis. 20:1945–1952.
- Liu J. 1995. Pharmacology of oleanolic acid and ursolic acid. J Ethnopharmacol. 49:57–68.
- Lorke D. 1983. A new approach to practical acute toxicity testing. Arch Toxicol. 54:275–286.
- Lui KC, Yang SC, Roberts MF. 1992. Antimalarial activity of Artemisia annua flavonoids from whole plants and cell cultures. Plants Cell. II:637–640.
- Maia JL, Lima-Junior RC, David JP, David JM, Santos FA, Rao VS. 2006. Oleanolic acid, a pentacyclic triterpene attenuates the mustard oil-induced colonic nociception in mice. Biol Pharm Bull. 29:82–85.
- Melariri P, Campbell W, Etusim P, Smith P. 2011. Antiplasmodial properties and bioassay-guided fractionation of ethyl acetate extracts from Carica papaya leaves. J Parasitol Res. doi: 10.1155/2011/10495.
- Melariri P, Campbell W, Etusim P, Smith P. 2012. In vitro and in vivo antimalarial activity of linolenic and linoleic acids and their methyl esters. Adv Stud Biol. 4:333–349.
- Middleton E Jr, Kandaswami C, Theoharides TC. 2000. The effects of plant flavonoids on mammalian cells: implications for inflammation, heart disease, and cancer. Pharmacol Rev. 52:673–751.
- Mosmann T. 1983. Rapid colorimetric assay for cellular growth and survival: application to proliferation and cytotoxicity assays. J Immunol Methods. 65:55–63.
- Nwafor PA, Jacks TW, Ekanem AU. 2007. Analgesic and anti-inflammatory effects of methanol extract of Pausinystalia mecroceras stem bark in rodents. J Pharmacol. 3:86–90.
- Nwafor PA, Nwajiobi N, Uko IE, Obot JS. 2010. Analgesic and anti-inflammatory activities of an ethanol extract of Smilax krausiana leaf in mice. Afr J Biomed Res. 13:141–148.
- Odetola A, Basir O. 1980. Evaluation of antimalarial properties of some Nigerian Medicinal Plants. In: Sofowora A, editor. Proceeding of African Bioscience Network, Federal Ministry of Science and Technology, Nigerian Society of Pharmacology and Drug Research and Production Unit. Ife: University of Ife organized Workshop; p. 275–283.
- Ogundipe OO, Moody JO, Houghton PJ, Odelola HA. 2001. Bioactive chemical constituents from Alchornea laxiflora (Benth) pax and Hoffman. J Ethnopharmacol. 74:275–280.
- Okokon JE, Nwafor PA. 2010. Antiinflammatory, analgesic and antipyretic activities of ethanolic root extract of Croton zambesicus. Pak J Pharmaceut Sci. 23:383–390.
- Oladiji AT, Olatunde A, Oloyede HO. 2014. Evaluation of anti-anaemic potential of aqueous extract of Alchornea laxiflora (Benth) leaf in iron deficient rats. Acad Arena. 6:2–29.
- Oladunmoye MK, Kehinde FY. 2011. Ethnobotanical survey of medicinal plants used in treating viral infections among Yoruba tribe of South Western Nigeria. Afr J Microbiol Res. 5:2991–3004.
- Oloyede GK, Onocha PA, Adaramoye OA, Thonda SE. 2011. Hepatoprotective activity and flavonoids of Alchornea laxiflora leaf extract. Res J Phytochem. 5:190.
- Oloyede GK, Onocha PA, Soyinka J, Oguntokun O, Thonda E. 2010. Phytochemical screening, antimicrobial and antioxidant activities of four Nigerian medicinal plants. Ann Biol Res. 1:114–120.
- Otuki MF, Ferreira J, Lima FV, Meyre-Silva C, Malheiros Â, Muller LA, Cani GS, Santos ARS, Yunes RA, Calixto JB. 2005. Antinociceptive properties of mixture of α-amyrin and β-amyrin triterpenes: evidence for participation of protein kinase C and protein kinase A pathways. J Pharmacol Exp Ther. 313:310–318.
- Peters W. 1965. Drug resistance in Plasmodium berghei Vincke and Lips, 1948. I. Chloroquine resistance. Exp Parasitol. 17:80–89.
- Philipson JD, Wright CW. 1991. Antiprotozoal compounds from plants sources. Planta Med. 57:553–559.
- Rajendran NN, Thirugnanasambandam P, Viswanathan S, Parvathavarthini S, Ramaswamy S. 2000. Antinociceptive pattern of flavone and its mechanism as tested by formalin assay. Indian J Exp Biol. 38:182–185.
- Ringbom T, Segura L, Noreen Y, Perera P, Bohlin L. 1998. Ursolic acid from Plantago major, a selective inhibitor of cyclooxygenase-2 catalysed prostaglandin biosynthesis. J Nat Prod. 61:1212–1215.
- Robak J, Shridi F, Wolbis M, Krolikowska M. 1998. Screening of the influence of flavonoids on lipoxygenase and cyclooxygenase activity, as well as on nonenzymic lipid oxidation. Polish J Pharmacol Pharm. 40:451–458.
- Ryley JF, Peters W. 1970. The antimalarial activity of some quinolone esters. Ann Trop Med Parasitol. 84:209–222.
- Santos AR, Cechinel Filho V, Niero R, Viana AM, Moreno FN, Campos MM, Yunes RA, Calixto JB. 1994. Analgesic effects of callus culture from selected species of Phyllanthus. J Pharm Pharmacol. 46:755–759.
- Setzer WN, Stokes SL, Penton AF, Takaku S, Haber WA, Hansell E, Caffrey CR, McKerrow JH. 2007. Cruzain Inhibitory activity of leaf essential oils of neotropical lauraceae and essential oil components. Nat Prod Commun. 2:1203–1210.
- Smilkstein M, Sriwilaijaroen N, Kelly JX, Wilairat P, Riscoe M. 2004. Simple and inexpensive fluorescence-based technique for high-throughput antimalarial drug screening. Antimicrob Agents Chemother. 48:1803–1806.
- Smith H, Brooks JR. 2014. Capsaicin-based therapies for pain control. Prog Drug Res. 68:129–146.
- Sofowora A. 1993. Medicinal plants and traditional medicine in Africa. 2nd ed. Ibadan, Nigeria: Spectrum Books Limited.
- Suh HW, Song DK, Son KH, Wie MB, Lee KH, Jung KY, Do JC, Kim YH. 1996. Antinociceptive mechanisms of dipsacus saponin C administered intracerebroventricularly in the mouse. Gen Pharmacol. 27:1167–1172.
- Suksamrarn A, Buaprom M, Udtip S, Nuntawong N, Haritakun R, Kanokmedhakul S. 2005. Antimycobacterial and antiplasmodial unsaturated carboxylic acid from the twigs of Scleropyrum wallichianum. Chem Pharm Bull. 53:1327–1329.
- Tapondjou LA, Lontsi D, Sondengam BL, Choi J, Lee KT, Jung HJ, Park HJ. 2003. In vivo anti-nociceptive and anti-inflammatory effect of the two triterpenes, ursolic acid and 23-hydroxyursolic acid, from Cussonia bancoensis. Arch Pharm Res. 26:143–146.
- Teffo LS, Aderogba MA, Eloff JN. 2010. Antibacterial and antioxidant activities of four kaempferol methyl ethers isolated from Dodonaea viscosa Jacq. Var. angustifolia leaf extracts. South Afr J Bot. 76:25–29.
- Trager W, Jensen JB. 1976. Human malaria parasites in continuous culture. Science. 193:673–675.
- Trease GE, Evans WC. 1989. Pharmacognosy. 13th ed. London: Bailliere Tindall; p. 683–684.
- Turner RA. 1995. Screening methods in pharmacology. Vol. 1. New York: Academic Press; p. 85–106.
- Vaz ZR, Cechinel V, Yunes RA, Calixto JB. 1996. Antinociceptive action of 2-(4-bromobenzoyl)-3-methyl-4-6-dimethoxy bezofuran, a novel xanthoxyline derivative of chemical and thermal models of nociception in mice. J Pharm Exp Ther. 278:304–312.
- Vaz ZR, Risley EA, Calixto JB. 1997. Analgesic effect of the herbal medicine catuama in thermal and chemical models of nociception in mice. Phytother Res. 11:101–106.
- Wibool R, Sae WC, Reanmongkol W, Wongnawaa M. 2008. Antinociceptive activity of the methanolic extract of Kaempferia galanga Linn. in experimental animals. J Ethnopharmacol. 118:225–230.
- Yi YL, Zong HA, Lu ZM, Xu HY, Zhang XM, Dou WF, Xu ZH. 2008. Analgesic and anti-inflammatory effects of the dry matter of culture broth of Termitomyces albuminosus and its extracts. J Ethnopharmacol. 120:432–436.