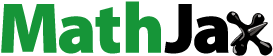
Abstract
Context: The use of Aframomum melegueta K. Schum. (Zingiberaceae) fruit for treatment of diabetes has recently been established in Nigeria. However, compounds responsible for the antidiabetic action have not been identified.
Objective: The present study carried out the bioassay-guided isolation of possible bioactive compounds responsible for the antidiabetic action of A. melegueta fruit.
Materials and methods: The A. melegueta fruit was sequentially extracted using ethyl acetate (EtOAc), ethanol and water, and the most active extract (EtOAc) was subjected to column chromatography on a silica gel column using solvent gradient systems of hexane (HEX):EtOAc and EtOAc:MeOH and the isolation of compounds was guided by α-glycosidase and α-amylase inhibitory activities at various concentrations (30–240 μg/mL).
Results: According to the results, 3 arylalkanes, 6-paradol (1), 6-shogaol (2) and 6-gingerol (3) and a pentacyclic triterpene, oleanolic acid (4) were isolated from A. melegueta fruit. All the compounds exhibited inhibitory effects against α-amylase and α-glucosidase. 6-Gingerol (3) and oleanolic acid (4) showed higher inhibitory activity against α-amylase (IC50: 6-gingerol: 81.78 ± 7.79 μM; oleanolic acid: 91.72 ± 1.63 μM) and α-glucosidase (IC50: 6-gingerol: 21.55 ± 0.45 μM; oleanolic acid: 17.35 ± 0.88 μM) compared to the standard drug, acarbose and other isolated compounds. The kinetics of the enzyme action of the compounds showed a noncompetitive mode of inhibition.
Conclusion: The data of this study suggest that the 6-gingerol (3) and oleanolic acid (4) showed higher α-amylase and α-glucosidase inhibitory action and therefore could be responsible for the antidiabetic activity of A. melegueta fruit.
Introduction
Type 2 diabetes (T2D) is a metabolic disorder characterized by chronic insulin resistance and loss of functional pancreatic β-cells due to relative (rather than absolute) deficiency of insulin (ADA Citation2015). The inability of the pancreatic β-cell to secrete enough insulin disrupts the regulation of hepatic glucose synthesis, muscles glucose uptake and adipose tissue lipolysis. The consequence is postprandial hyperglycemia, which subsequently causes T2D (Gastaldelli Citation2011). This postprandial hyperglycemia must be controlled in order to prevent diabetic complications (Rahati et al. Citation2014). Among many therapeutic approaches to reduce postprandial hyperglycemia in T2D, delaying glucose absorption via inhibition of intestinal carbohydrate-digesting enzymes, such as α-glucosidase and α-amylase, has gained popularity in the recent years (Imam Citation2015). Acarbose and miglitol are the two major available glucosidase inhibitors currently used in the treatment of T2D. However, major drawbacks in using these existing drugs (acarbose and miglitol) are diarrhoea and abdominal pain (Irons & Minze Citation2014). Thus, the search for potent antidiabetic drugs with fewer side effects has been shifted to plant-based natural products (Cragg & Newman Citation2013). The use of plant-derived extracts and/or compounds as an alternative to synthetic drugs is preferred, as they possess fewer side-effects (Taveira et al. Citation2010).
Aframomum melegueta K. Schum. (Zingiberaceae) commonly known as guinea or alligator pepper is abundantly found in central and western parts of Africa (Iwu Citation2014). The fruit, leaf and seeds are used as flavouring agents in various traditional foods and for the treatment of diseases including diabetes and other related disorders in many parts of Africa (Abo et al. Citation2008; Gbolade Citation2009). Previous studies have shown that A. melegueta has hepato-protective (Nwozo & Oyinloye Citation2011), anti-inflammatory (Ilic et al. Citation2014) and antioxidative (Onoja et al. Citation2014) effects at in vitro and in vivo conditions. In a recent study, the diterpenoids isolated from the organic seed extract of A. melegueta showed bactericidal activity in vitro against Escherichia coli, Listeria moncyogenes and Staphylococcus aureus strains (Ngwoke et al. Citation2014). In another study, 6-paradol (1), 6-shogaol (2) and 6-gingerol (3) isolated from the seed have shown anti-inflammatory activity in a rat paw oedema model (Ilic et al. Citation2014). El-Halawany et al. (Citation2014) have also reported that diarylheptanoid, 8-dehydrogingerdione, 6-dehydroparadol, dihydrogingerenone, 6-gingerol, dihydroparadol, 6-paradol and 6-shogaol demonstrated hepato-protective and antioxidative actions in CCl4 induced acute liver injury in rats.
Furthermore, some previous studies have reported that various extracts from A. melegueta have demonstrated inhibitory effects against α-glucosidase and α-amylase actions (Etoundi et al. Citation2010; Adefegha & Oboh Citation2011, Citation2012; Kazeem et al. Citation2012) which has been supported by our recent study (Mohammed et al. Citation2016). The seed and leaf extracts were also reported to demonstrate blood glucose lowering ability in alloxan-induced diabetic rats (Adesokan et al. Citation2010; Mojekwu et al. Citation2011). Additionally, our recent findings have shown that the ethyl acetate (EtOAc) fraction derived from the crude ethanol extract exhibited inhibitory effects against α-glucosidase and α-amylase activities in vitro (Mohammed et al. Citation2016), anti-hyperglycemic and anti-hyperlipidemic actions in experimentally-induced animal model of T2D (Mohammed et al. Citation2015). They have also shown the ability to ameliorate β-cell dysfunction and other major diabetes-related complications in a T2D model of rats (Mohammed et al. Citation2015). However, the compounds responsible for the antidiabetic action remain elusive.
Hence, the present study aimed at carrying out a bioassay-guided isolation of possible bioactive compounds responsible for the antidiabetic action of the A. melegueta fruit and their inhibitory effects on enzymes linked to T2D.
Materials and methods
Materials
Saccharomyces cerevisiae α-glucosidase (E.C. 3.2.1.20), porcine pancreatic α-amylase (E.C. 3.2.1.1), p-nitrophenyl glucopyranoside (pNPG) and p-nitrophenol were obtained from Sigma-Aldrich through Capital Lab Supplies, New Germany, South Africa. Starch, dinitrosalicylic acid (DNS), maltose, absolute ethanol and ethyl acetate were obtained from Merck Chemical Company, Durban, South Africa.
Plant material
The fruit of A. melegueta was freshly collected in December, 2012 from Ibadan, Oyo State, Nigeria and identified and authenticated at the herbarium unit of the Biological Science Department, Ahmadu Bello University, Zaria, Nigeria by Mr. Umar Gallah. A voucher specimen number 1511 was deposited accordingly. The sample was immediately washed and shade-dried for two weeks to constant weight. The dried sample was ground to a fine powder, and stored individually in airtight containers to transport to the University of KwaZulu-Natal, Westville Campus, Durban, South Africa for further investigations.
Preparation of plant extracts
Extraction and fractionation were carried out according to the method reported previously (Ibrahim & Islam Citation2014). The A. melegueta EtOAc fraction was a dark greenish residue and demonstrated the highest in vitro α-glucosidase (IC50: 40.44 ± 5.77 μg/mL) and α-amylase (IC50: 68.69 ± 6.05 μg/mL) inhibitory activity amongst the fractions and thus selected for the isolation of possible bioactive compounds.
Isolation of bioactive compounds from the EtOAc fraction
The EtOAc fraction (15.5 g) of A. melegueta fruit was subjected to column chromatography on a silica gel column using gradient solvent systems of hexane (HEX):EtOAc and EtOAc:MeOH with increments of 10% in each step to obtain 84 fractions. After monitoring with TLC, similar fractions were pooled together to obtain seven major fractions (A: 11–13; B: 14–17; C: 20–25; D: 26–32; E: 33–34; F: 35–47 and G: 48–55) that exhibited considerably higher inhibitory effects against α-amylase and α-glucosidase activities. Compound 1 (210.0 mg), was obtained as an oil from fraction C (2.51 g) after further purification on a column (2.0 cm diameter) using HEX:EtOAc (17:3) as an eluent. Fraction D (990.3 mg) was further purified on a column (1.5 cm in diameter) by eluting with HEX:EtOAc (8:2) to obtain compound 2 (490.0 mg) as an oil. Compound 3 (990.9 mg) was obtained as an oil by the purification of fraction F (4.13 g) on a silica column and eluted with HEX: EtOAc (3:2). Fraction E (100.9 mg) was further purified with a HEX:EtOAc (7:3) solvent system, which yielded compound 4 (60.0 mg). All isolated bioactive compounds demonstrated α-amylase and α-glucosidase inhibitory actions at different scale.
All NMR data, 1H, 13C and 2D experiments were recorded on a Bruker Avance III 400 MHz spectrometer. Samples were acquired with deuterated chloroform (CDCl3). The spectra were referenced according to the deuteriochloroform signal at δH 7.24 (for 1H NMR spectra) and δC 77.0 (for 13C NMR spectra) for CDCl3. The HRESIMS spectra were obtained on a Bruker Micro TOF-QII instrument (Coventry, UK).
α-Amylase (E.C. 3.2.1.1) inhibitory effects
The α-amylase inhibitory effect of the compounds was carried out using a modified method of McCue and Shetty (Citation2004). Briefly, a 250 μL aliquot of compounds at different concentrations (30, 60, 120 and 240 μg/mL) was placed in a test tube and 250 μL of 0.02 M sodium phosphate buffer (pH 6.9) containing α-amylase (2.0 U/mL) solution was added. This solution was pre-incubated at 25 °C for 10 min, after which 250 μL of 1% starch solution in 0.02 M sodium phosphate buffer (pH 6.9) was added at a time interval of 10 s and then further incubated at 25 °C for 10 min. The reaction was terminated after incubation by adding 1 mL of dinitrosalicylic acid (DNS) reagent. The tube was then boiled for 10 min and cooled to room temperature. The reaction mixture was diluted with 5 mL distilled water and the absorbance was measured at 540 nm using a Shimadzu UV mini 1240 spectrophotometer (Shimadzu Corporation, Kyoto, Japan). A control was prepared using the same procedure using distilled water instead of the compound.
The results of α-amylase inhibitory effects of compounds were expressed as a percentage of control (blank) according to the following formula:
α-Glucosidase (E.C. 3.2.1.20) inhibitory effects
The inhibitory effect of the isolated compounds on α-glucosidase activity was determined according to the method described by Kim et al. (Citation2005) using α-glucosidase from Saccharomyces cerevisiae. The 5.0 mM p-nitrophenyl glucopyranoside (pNPG) substrate solution was prepared in 20 mM phosphate buffer, pH 6.9. An aliquot of 500 μL of α-glucosidase (1.0 U/mL) was then pre-incubated with 250 μL of the different concentrations of compounds (30, 60, 120 and 240 μg/mL) for 10 min. Thereafter, 250 μL of 5.0 mM pNPG was dissolved in 20 mM phosphate buffer (pH 6.9) as a substrate to start the reaction. The reaction mixture was incubated at 37 °C for 30 min. The α-glucosidase activity was determined by measuring the yellow coloured p-nitrophenol released from pNPG at 405 nm.
The results of α-glucosidase inhibitory effects of compounds were expressed as a percentage of the control (blank) according to the following formula:
Mechanism of α-amylase and α-glucosidase inhibitions
The isolated compounds were subjected to kinetic experiments to determine the type of inhibition exerted on α-amylase and α-glucosidase. The experiment was conducted according to the protocols mentioned above at a constant concentration of the compounds (30 μg/mL) with a variable concentration of substrate. For the α-glucosidase inhibition assay, 0.313–5.0 mM pNPG and 0.063–1.0% starch was used. The initial rates of reactions were determined from calibration curves constructed using varying concentrations of p-nitrophenol (0.313–5.0 mM) and maltose (0.063–1.0%) for the α-glucosidase and α-amylase inhibition assays, respectively. The initial velocity data obtained were used to construct Lineweaver-Burke plots to determine the KM (Michaelis constant) and vmax (maximum velocity) of the enzyme as well as the Ki (inhibition binding constant as a measure of affinity of the inhibitor to the enzyme) and the type of inhibition for both enzymes.
Statistical analysis
Data are presented as mean ± SD (n = 3). Data were analyzed by using a statistical software package (SPSS for Windows, version 18, IBM Corporation, NY) using Tukey’s-HSD multiple range post hoc test. Values were considered significantly different at p < 0.05.
Results and discussion
The phytochemical analysis of A. melegueta fruit led to the isolation of pure bioactive compounds 1–4 ( and ) which were characterized by 1D (1H and 13C) and 2D NMR and confirmed by comparison with data in the literature as 6-paradol (1) (Ma et al. Citation2004), 6-shogoal (2) and 6-gingerol (3) (Lee et al. Citation2011) and oleanolic acid (4) (Mahato & Kundu Citation1994). Compounds (1–3) are arylalkanes common to the Zingeberaceae. They all have a common 4-hydroxy-3-methoxyphenyl moiety and differ in the side chain due to the biosynthetic conversion of 6-paradol (1) to 6-gingerol (3) by hydroxylation and dehydration to 6-shogoal (2) (). These compounds were previously isolated from A. melegueta seeds and reported to possess a variety of interesting pharmacological properties including hepato-protective and antioxidant activity (El-Halawany et al. Citation2014), as well as anti-inflammatory activity (Dugasani et al. Citation2010; Ilic et al. Citation2014). Furthermore, previous studies on the phytochemistry of A. melegueta indicated that this plant contains monoterpenoids, sesquiterpenoids and diterpenoids (Tane et al. Citation2005). However, in our present study, oleanolic acid (4), a pentacyclic triterpene, was isolated from A. melegueta fruit for the first time, which is not surprising since it is found quite commonly in the plant kingdom. Oleanolic acid (4) is a potent pharmacologically active compound having antioxidant, anti-inflammatory and antidiabetic activity (Pollier & Goossens Citation2012).
Table 1. 1H and 13C NMR data of compounds 1–4 isolated from A. melegueta (more details in the supplementary data file).
The data of the in vitro antidiabetic effect (inhibition of α-amylase and α-glucosidase activity) of the compounds from A. melegueta are presented in and (IC50 values). It was observed that the IC50 values exhibited by oleanolic acid (4) (α-amylase: 91.72 ± 1.63 μM; α-glucosidase: 17.35 ± 0.88 μM) were significantly lower (p < 0.05) than the standard antidiabetic drug, acarbose and other isolated compounds (). According to some previous studies by de Melo et al. (Citation2010), Ali et al. (Citation2002) and Komaki et al. (Citation2003), oleanolic acid (4) was shown to have an inhibitory effect on α-amylase (IC50: 154.89 μM) and α-glucosidase (IC50: 11.16 μM), respectively. This is in line with our present data and our study also additionally showed that oleanolic acid (4) is a potent inhibitor of α-glucosidase rather than α-amylase. It has been also reported that excessive inhibition of α-amylase action causes some undesirable consequences such as diarrhoea, bloating, flatulence, cramping and abdominal pain (Fujisawa et al. Citation2005). Hence, oleanolic acid (4) can be a better alternative to acarbose as an antidiabetic drug.
Figure 2. α-Amylase (A) and α-glucosidase (B) inhibitions (%) of compounds isolated from A. melegueta fruit. Data are presented as mean ± SD of triplicate determinations. a–e Values with different letters presented for a given concentration for each extract are significantly different from each other.

Table 2. IC50 values of bioactive compounds isolated from the A. melegueta fruit in antidiabetic models.
Furthermore, the IC50 values observed with 6-gingerol (3) (α-amylase: 81.78 ± 7.79 μM; α-glucosidase: 21.55 ± 0.45 μM) were significantly lower (p < 0.05) compared to other two arylalkanes (). This shows that the C-5 hydroxyl group of the aliphatic side chain of 6-gingerol (3) contributed to the activity of this compound, however this is subject to further study. Moreover, the isolated arylalkanes (compounds 1–3) have not been tested previously on α-amylase or α-glucosidase inhibitory actions, however, previous studies have shown that 6-shogoal (2) and 6-gingerol (3) play a crucial role in the treatment and control of T2D (Isa et al. Citation2008; Singh et al. Citation2009; Chakraborty et al. Citation2012; Shao et al. Citation2016). The lowest calculated IC50 values of compound 4 (α-amylase: 91.72 ± 1.63 μM; α-glucosidase: 17.35 ± 0.88 μM) and compound 3 (α-amylase: 81.78 ± 7.79 μM; α-glucosidase: 21.55 ± 0.45 μM) confirmed the excellent antidiabetic effects of A. melegueta fruit extract observed in our previous studies (Mohammed et al. Citation2015, Citation2016) which might be attributed to the presence of these compounds. Hence, 6-gingerol (3) and oleanolic acid (4) may be the most suitable antidiabetic agents compared to other isolated compounds. Additionally, a number of previous studies reported that 6-gingerol (3) and oleanolic acid (4) promote insulin secretion from pancreatic β-cells (Hsu et al. Citation2006; Teodoro et al. Citation2008; Lee et al. Citation2015). Therefore, the relatively elevated insulin levels observed in our previous study using the A. melengueta extract (Mohammed et al. Citation2015), could be attributed to the presence of some of these compounds as well.
More importantly, according to our previous data, the IC50 values of the compounds (1–4) were lower compared to that of crude ethanol extract (α-amylase: 0.62 ± 0.01 mg/mL; α-glucosidase: 0.06 ± 0.01 mg/mL), EtOAc fraction (α-amylase: 68.69 ± 6.05 μg/mL; α-glucosidase: 40.44 ± 5.77 μg/mL) (Mohammed et al. Citation2015, Citation2016) as well as other fractions (IC50 values >200 μg/mL) derived from the bioassay-guided analysis. This shows that further purification of the A. melegueta fruit extract leads to an increase α-amylase and α-glucosidase inhibitory effects. Therefore, the data of our present study indicate that the antidiabetic activity of A. melegueta fruit reported earlier (Adesokan et al. Citation2010; Mohammed et al. Citation2015, Citation2016) is attributed to the presence of isolated compounds (1–4).
The kinetic data of the inhibitory mode of α-amylase and α-glucosidase enzymes by the isolated compounds are presented in and . The unchanged KM and lower vmax values denote the noncompetitive inhibition of α-amylase and α-glucosidase action by the compounds 1 and 3 (, ). Additionally, oleanolic acid (4) and 6-shogaol (2) showed a mixed inhibition type for α-amylase action where both the KM and vmax values were altered ( and ). It is therefore suggested that these compounds bind to other site(s), apart from the active site of the enzyme and induced a conformational change in the three-dimensional structure of the enzymes and ultimately slowed down their activities. The equilibrium constant for inhibitor binding (Ki) of the compounds were lower for α-glucosidase than α-amylase, indicating greater stability of the enzyme-substrate complex for α-glucosidase compared to α-amylase enzyme (). This again confirms the potential of these compounds, particularly 6-gingerol (3) and oleanolic acid (4), for the development of novel and better antidiabetic drugs.
Figure 3. Lineweaver-Burke plots for α-amylase (A) and α-glucosidase (B) in the absence and presence of the inhibitors (bioactive compounds).

Table 3. Kinetic analysis of α-amylase and α-glucosidase inhibition by compounds isolated from the fruit of A. melegueta in antidiabetic models.
Conclusions
In conclusion, the results of the present study indicate that 6-gingerol (3) and oleanolic acid (4) isolated from A. melegueta fruit demonstrate higher α-amylase and α-glucosidase inhibitory activity compared to other isolated compounds and could be regarded as possible antidiabetic agents. Therefore, the antidiabetic activity of the fruit of A. melegueta is attributed to the presence of these bioactive ingredients. Further clinical and detailed toxicological studies are required to fully confirm the potency of 6-gingerol (3) and oleanolic acid (4) as possible antidiabetic agents.
Md_Shahidul_Islam_et_al_supplemental_content.zip
Download Zip (1.3 MB)Acknowledgements
This study was supported by a competitive research grant from the Research Office, University of KwaZulu-Natal (UKZN), Durban; an incentive grant for rated researchers and a grant support for women and young researchers from the National Research Foundation (NRF), Pretoria, South Africa. The first author was awarded a PhD study fellowship by the Ahmadu Bello University, Zaria, Nigeria. Authors would like to thank Mr. Olusola Bodede and Mr. Olumuyiwa Ogunlaja for their assistance during the study.
Disclosure statement
We declare that we have no conflict of interest.
Additional information
Funding
References
- Abo KA, Fred-Jaiyesimi AA, Jaiyesimi AEA. 2008. Ethnobotanical studies of medicinal plants used in the management of diabetes mellitus in South Western Nigeria. J Ethnopharmacol. 115:67–71.
- Adefegha SA, Oboh G. 2012. Inhibition of key enzymes linked to type 2 diabetes and sodium nitroprusside-induced lipid peroxidation in rat pancreas by water extractable phytochemicals from some tropical species. Pharm Biol. 50:857–865.
- Adefegha SA, Oboh G. 2011. Water extractable phytochemicals from some Nigerian spices inhibit Fe2+- induced lipid peroxidation in rat’s brain-in vitro. J Food Process Technol. 2:1–6.
- Adesokan AA, Akanji MA, Adewara GS. 2010. Evaluation of hypoglycaemic efficacy of aqueous seed extract of Aframomum melegueta in alloxan-induced diabetic rats. Sierra Leone J Biomed Res. 2:91–94.
- Ali MS, Jahangir M, Hussan SS, Choudhary MI. 2002. Inhibition of alpha-glucosidase by oleanolic acid and its synthetic derivatives. Phytochemistry. 60:295–299.
- American Diabetes Association (ADA). 2015. Report of the expert committee on the diagnosis and classification of diabetes mellitus. Diabetes Care. 38:S8–S16.
- Chakraborty D, Mukherjee A, Sikdar S, Paul A, Ghosh S, Khuda-Bukhsh AR. 2012. [6]-Gingerol isolated from ginger attenuates sodium arsenite induced oxidative stress and plays a corrective role in improving insulin signaling in mice. Toxicol Lett. 210:34–43.
- Cragg GM, Newman DJ. 2013. Natural products: a continuing source of novel drug leads. Biochim Biophys Acta. 1830:3670–3695.
- de Melo CL, Queiroz MGR, Fonseca SGC. 2010. Oleanolic acid, a natural triterpenoid improves blood glucose tolerance in normal mice and ameliorates visceral obesity in mice fed a high-fat diet. Chem Biol Interact. 185:59–65.
- Dugasani S, Pichika MR, Nadarajah VD, Balijepalli MK, Tandra S, Korlakunta JN. 2010. Comparative antioxidant and anti-inflammatory effects of [6]-gingerol, [8]-gingerol, [10]-gingerol and [6]-shogaol. J Ethnopharmacol. 127:515–520.
- El-Halawany AM, El Dine RS, El Sayed NS, Hattori M. 2014. Protective effect of Aframomum melegueta phenolics against CCl4-induced rat hepatocytes damage; role of apoptosis and pro-inflammatory cytokines inhibition. Sci Rep. 4:1–9.
- Etoundi CB, Kuaté D, Ngondi JL, Oben J. 2010. Anti-amylase, anti-lipase and antioxidant effects of aqueous extracts of some Cameroonian spices. J Nat Prod. 3:165–171.
- Fujisawa T, Ikegami H, Inoue K, Kawabata Y, Ogihara T. 2005. Effect of two α-glucosidase inhibitors, voglibose and acarbose, on postprandial hyperglycemia correlates with subjective abdominal symptoms. Metabolism. 54:387–390.
- Gastaldelli A. 2011. Role of beta-cell dysfunction, ectopic fat accumulation and insulin resistance in the pathogenesis of type 2 diabetes mellitus. Diabetes Res Clin Pract. 93:S63–S66.
- Gbolade A. 2009. Inventory of antidiabetic plants in selected districts of Lagos State, Nigeria. J Ethnopharmacol. 121:135–139.
- Hsu JH, Wu YC, Liu IM, Cheng JT. 2006. Release of acetylcholine to raise insulin secretion in Wistar rats by oleanolic acid, one of the active principles contained in Cornus officinalis. Neurosci Lett. 404:112–116.
- Ibrahim MA, Islam MS. 2014. Butanol fraction of Khaya senegalensis root modulates β-cell function and ameliorates diabetes-related biochemical parameters in a type 2 diabetes rat model. J Ethnopharmacol. 154:832–838.
- Ilic NM, Dey M, Poulev AA, Logendra S, Kuhn PE, Raskin I. 2014. Anti-inflammatory activity of grains of paradise (Aframomum melegueta Schum) extract. J Agric Food Chem. 62:10452–10457.
- Imam SK. 2015. Diabetes: a new horizon and approach to management. In: Watson RR, Dokken BB, editors. Glucose Intake and Utilization in Pre-diabetes and Diabetes Implications for Cardiovascular Disease. UK: Elsevier Academic Press. p. 30–44.
- Irons BK, Minze MG. 2014. Drug treatment of type 2 diabetes mellitus in patients for whom metformin is contraindicated. Diabetes Metab Syndr Obes. 7:15–24.
- Isa Y, Miyakawa Y, Yanagisawa M, Goto T, Kang MS, Kawada T, Morimitsu Y, Kubota K, Tsuda T. 2008. 6-Shogaol and 6-gingerol, the pungent of ginger, inhibit TNF-alpha mediated downregulation of adiponectin expression via different mechanisms in 3T3-L1 adipocytes. Biochem Biophys Res Commun. 373:429–434.
- Iwu MM. 2014. Handbook of African Medicinal Plants. 2nd ed. Taylor and Francis Group (NY): CRC Press.
- Kazeem MI, Akanji MA, Hafizur Rahman M, Choudhary MI. 2012. Antiglycation, antioxidant and toxicological potential of polyphenol extracts of alligator pepper, ginger and nutmeg from Nigeria. Asian Pac J Trop Biomed. 2:727–732.
- Kim YM, Jeong YK, Wang MH, Lee WY, Rhee HI. 2005. Inhibitory effect of pine extract on alpha-glucosidase activity and postprandial hyperglycemia. Nutrition. 21:756–761.
- Komaki E, Yamaguchi S, Maru I. 2003. Identification of anti-amylase components from olive leaf extracts. Food Sci Technol Res. 9:35–39.
- Lee C, Park GH, Kim CY, Jang JH. 2011. [6]-Gingerol attenuates β-amyloid-induced oxidative cell death via fortifying cellular antioxidant defense system. Food Chem Toxicol. 49:1261–1269.
- Lee JO, Kim N, Lee HJ, Moon JW, Lee SK, Kim SJ, Park SH, Kim HS. 2015. [6]‐Gingerol affects glucose metabolism by dual regulation via the AMPKα2‐mediated AS160-Rab5 pathway and AMPK‐mediated insulin sensitizing effects. J Cell Biochem. 116:1401–1410.
- Ma J, Jin X, Yang L, Liu ZL. 2004. Diarylheptanoids from the rhizomes of Zingiber officinale. Phytochemistry. 65:1137–1143.
- Mahato SB, Kundu AP. 1994. 13C NMR spectra of pentacyclic triterpenoids-A compilation and some salient features. Phytochemistry. 37:1517–1575.
- McCue P, Shetty K. 2004. Inhibitory effects of rosmarinic acid extracts on porcine pancreatic amylase in vitro. Asian Pac J Clin Nutr. 13:101–106.
- Mohammed A, Koorbanally NA, Islam MS. 2016. Antioxidative activity, phytochemistry, and inhibition of key enzymes linked to type 2 diabetes by various parts of Aframomum melegueta in vitro. Acta Pol Pharm Drug Res. 73:403–417.
- Mohammed A, Koorbanally NA, Islam MS. 2015. Ethyl acetate fraction of Aframomum melegueta fruit ameliorates pancreatic β-cell dysfunction and major diabetes-related parameters in a type 2 diabetes model of rats. J Ethnopharmacol. 175:518–527.
- Mojekwu TO, Yama OE, Ojokuku SA, Oyebadejo SA. 2011. Hypoglyceamic effects of aqueous extract of Aframomum melegueta leaf on alloxan-induced diabetic male albino rats. Pac J Med Sci. 8:28–36.
- Ngwoke KG, Chevallier O, Wirkom VK, Stevenson P, Elliott CT, Situ C. 2014. In vitro bactericidal activity of diterpenoids isolated from Aframomum melegueta K. Schum against strains of Esceherichia coli, Listeria monocytogenes and Staphylococcus aureus. J Ethnopharmacol. 153:1147–1154.
- Nwozo SO, Oyinloye BE. 2011. Hepatoprotective effect of aqueous extract of Aframomum melegueta on ethanol-induced toxicity in rats. Acta Biochim Pol. 58:355–358.
- Onoja SO, Omeh YN, Ezeja MI, Chukwu MN. 2014. Evaluation of the in vitro and in vivo antioxidant potentials of Aframomum melegueta Methanolic Seed Extract. J Trop Med. 2014: http://dx.doi.org/10.1155/2014/159343.
- Pollier J, Goossens A. 2012. Oleanolic acid. Phytochemistry. 77:10–15.
- Rahati S, Shahraki M, Arjomand G, Shahraki T. 2014. Food pattern, lifestyle and diabetes mellitus. Int J High Risk Behav Addict. 3:e8725
- Shao Y, Yu Y, Li C, Yu J, Zong R, Pei C. 2016. Synergistic effect of quercetin and 6-gingerol treatment in streptozotocin induced type 2 diabetic rats and poloxamer P-407 induced hyperlipidemia. RSC Adv. 6:12235–12242.
- Singh AB, Singh N, Maurya R, Srivastava AK. 2009. Anti-hyperglycaemic, lipid lowering and antioxidant properties of [6]-gingerol in db/db mice. Int J Med Sci. 1:536–544.
- Tane P, Tatsimo SD, Ayimele GA, Connolly JD. 2005. Bioactive metabolites from Aframomum species. 11th NAPRECA Symposium Book of Proceedings; Antananarivo, Madagascar. p. 214–223.
- Taveira M, Silva LR, Vale-Silva LA, Pinto E, Valentão P, Ferreres F, Guedes P, Andrade PB. 2010. Lycopersicon esculentum seeds: an industrial byproduct as an antimicrobial agent. J Agric Food Chem. 58:9529–9536.
- Teodoro T, Zhang L, Alexander T, Yue J, Vranic M, Volchuk A. 2008. Oleanolic acid enhances insulin secretion in pancreatic beta-cells. FEBS Lett. 582:1375–1380.