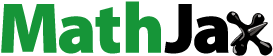
Abstract
Context: Previous studies have shown that Spirulina platensis Gomont (Phormidiaceae) (SP) extract has beneficial effects on many disease conditions. The putative protective effects of SP were investigated in diabetic rats.
Objective: The current study investigates the antioxidant effects of SP in diabetic Wistar rats.
Materials and methods: Alloxan monohydrate (150 mg/kg body weight) was intraperitoneally administrated to induce diabetes. An aqueous suspension of SP powder in distillate water (10% w/v) was administrated orally by gavage (1 mL/day) for 50 days. Histopathological, biochemical and antioxidant analyses were performed. Glycemia, liver function and HOMA-IR were assessed using Spinreact and ELISA kits.
Results: SP exhibited high-antioxidant activity. The IC50 values of the SP aqueous extract were 70.40 and 45.69 mg/L compared to those of the standard antioxidant BHT, which were 27.97 and 19.77 mg/L, for the DPPH and ABTS tests, respectively. The diabetic animals showed a significant increase in glycaemia (from 4.05 to 4.28 g/L) and thiobarbituric acid reactive substances (50.17 mmol/g protein) levels. Treatment with SP significantly reduced glycaemia by 79% and liver function markers [glutamate pyruvate transaminase (GPT), glutamate oxaloacetate transaminase (GOT) and alkaline phosphatase (Alk-p)]) by 25, 36 and 20%, respectively, compared to that of the controls. There was a significant increase in superoxide dismutase (48%), total antioxidant status (43%), glutathione peroxidase (37%) and glutathione reductase (16%) in the diabetic rats treated with SP.
Discussion and conclusion: These results showed that SP has high antioxidant activity, free radical scavenging, antihyperglycemic and hepatoprotective effects in diabetes.
Introduction
In the past decade, the number of diabetes mellitus cases has gradually increased. This disease is the third leading cause of death worldwide, and the prevalence of diabetes for all age groups was estimated to be 2.8% in 2000 and is predicted to be 4.4% by 2030 (Wild et al. Citation2004). Diabetes mellitus is a multifactorial disease characterized by hyperglycaemia and increased basal metabolic rate (Bos & Agyemang Citation2013). In these conditions, the body does not produce sufficient insulin, a hormone produced by the β pancreatic cells. Insulin enables cells to absorb glucose and convert it into energy (Pankaj & Varma Citation2013). High blood glucose levels damage the cell membranes and generate reactive oxygen species (ROS) (Ha & Kim Citation1999). Despite the intensive effort to control this disease by pharmaceutical methods, antidiabetic drugs are still largely restricted due to their adverse effects and their expense (Zhang & Moller Citation2000; Oliveira et al. Citation2005; Abdel-Daim & Halawa Citation2014). Metformin is an antihyperglycemic drug used in the regulation of diabetes mellitus. To avoid the harmful side effects of chemical drugs, researchers have investigated natural products such as extracts from Haematoccus pluviaris Gomont (Haematococcaceae) and Spirulina platensis Gomont (Phormidiaceae) (SP), that possess antidiabetic effects and contribute to the nutrient requirements, stimulate the endocrine system and intermediate nutrient metabolism (Khan et al. Citation2005; Thormar Citation2012).
SP is a blue-green algae belonging to the cyanobacteria family that is rich in bioactive compounds, such as proteins, lipids, carbohydrates, trace elements (zinc, magnesium, manganese, selenium), pigments (phycocyanin, β-carotene), riboflavin, tocopherol and α-linoleic acid (Göksan & Kılıc¸ Citation2009; Yang & Zhang Citation2009; Yusuf et al. Citation2016). SP and its extracts are widely used as nutrients for human and animal consumption, natural dyes in food and cosmetics and nutraceutical and food additives for pharmaceutical industries (Zheng et al. Citation2013). Multiple studies worldwide have reported that Spirulina species can regulate diabetic processes, such as hypercholesterolemic activity, and have antioxidative effects and radical scavenging properties which provide significant multiorgan protection and ameliorate the effect of many drugs and chemicals-induced toxic assaults in laboratory animals (Pankaj & Varma Citation2013; Abdel-Daim Citation2014; Ibrahim & Abdel-Daim Citation2c015; Abdel-Daim et al. Citation2016). The major objective of this study was to determine the effects of SP on metabolic abnormalities, oxidative stress and histological changes in diabetic rats treated with alloxan.
Materials and methods
Phytochemical screening of SP
Determination of total polyphenols and flavonoids
An aqueous extract of SP (AESP) was prepared in 2014 as described by Chu et al. (Citation2010). Total polyphenols of AESP were measured using the Folin–Ciocalteu method (Singleton et al. Citation1999); gallic acid was used as a standard for the calibration curve. The flavonoids of AESP were measured using the AlCl3 method (Lamaison & Carnet Citation1990). Quercetin was used as a standard for the calibration curve.
Determination of phycobiliproteins and total carotenoids
SP powder (1 g) was suspended in 100 mL of sodium-phosphate buffer (0.1 M, pH 7.0, 1 mM sodium azide). The suspension was disrupted by sonication at 50 Hz for 60 s followed by freezing at −20 °C and thawing at room temperature (25 °C) in the dark. Extraction of the phycobiliproteins was performed by centrifuging the pretreated SP cell suspension at 10,000 g for 30 min at 4 °C. The dark-blue phycobiliprotein supernatant was collected, and the pellet was discarded. The absorbance of the phycocyanines (C-PC), allophycocyanines (APC) and phycoerythrines (PE) of the phycobiliprotein solution was measured using a SPECTRONIC® 20 GENESYS™ UV-Vis spectrophotometer (Virginia, USA) at wavelengths of 620, 652 and 562 nm, respectively (Anamika et al. Citation2005).
For determination of the concentrations of C-PC, APC and PE, the following EquationEquations (1–3) were used (Bennett & Bogorad Citation1973):(1)
(1)
(2)
(2)
(3)
(3)
Total carotenoids were also determined spectrophotometrically at 470 nm using the same UV/Vis spectrophotometer. The concentrations of chlorophyl a (Ca) and chlorophyl b (Cb) were determined at 653 and 666 nm, respectively. Total carotenoids and Ca and Cb levels were calculated based on EquationEquations (4–6) (Lichtenthaler & Wellburn Citation1985).(4)
(4)
(5)
(5)
(6)
(6)
Determination of the antiradical and antioxidant activity and the IC50
The 2,2-diphenyl-1-picrylhydrazyl (DPPH) antiradical test was carried out as described by Burits & Bucar (Citation2000). Butylated hydroxyl toluene (BHT) at 100 μg/mL was used as a positive control. The antiradical activity of the tested samples was calculated using the following equation (EquationEquation 7(7)
(7) ):
(7)
(7)
A.s and A.st are the absorbance of the tested samples and the DPPH, respectively.
The antioxidant activity assay is based on the ability of different fractions to scavenge 2, 2′-azino-bis (ethylbenzothiazoline-6-sulfonic acid: ABTS. +) (ABTS), a radical cation, compared to that of the standard (BHT). The antioxidative activity of the tested samples was calculated using the following equation (EquationEquation 8(8)
(8) ) (Re et al. Citation1999):
(8)
(8)
At and Ac are the absorbance of the tested samples and ABTS+, respectively.
The extract concentration resulting in 50% inhibition (IC50) of DPPH, ABTS and the standard BHT was calculated from the graph plotting the inhibition percentages against the extract concentration. DPPH, ABTS and BHT were purchased from Sigma Aldrich GmbH, Sternheim, Germany.
Animals
Adult male rats (Wistar strain; age: 10–13 weeks; weight: 180–200 g) were obtained from the Pasteur Institute of Algiers (Algeria). The rats were housed in polypropylene cages and maintained on a standard pellet diet (National Office for Food Livestock, Algiers, Algeria) with access to water ad libitum under standard conditions of temperature (24 ± 2 °C) and relative humidity (60–70%) with a 12 h light/dark cycle. All of the experimental procedures were approved by the Algerian Institutional Animal Care Committee, which belongs to the National Administration of Algerian Higher Education and Scientific Research (Algiers).
Diabetes induction
Freshly prepared alloxan monohydrate [150 mg/kg body weight (b.w.)] purchased from Sigma-Aldrich Co. (USA) was intraperitoneally administrated to overnight-fasted rats (Kameswara Rao et al. Citation1999). Fructose was added to the drinking water to prevent hypoglycemic crisis. The same volume of sodium chloride (0.9% solution) was injected into the control rats. Fasting blood sugar (FBS) of the animals was measured after 72 h. Animals with an FBS level ≥2 g/L were considered diabetic.
Experimental design
All of the animals were randomized and divided into six groups, with eight rats in each group, as described in . We distinguished four major steps, which were as follows: day 0 to day 10 (week of adaptation), day 10 to day 20 (diabetes induction by alloxan), day 20 to day 60 (treatment with SP and metformin), and day 60 to day 70 (therapy arrest).
Table 1. Experimental design.
The SP powder was obtained from the Tractebel Consult Office in association with the University Center of Biotechnology Algae, Tchad in 2013. It was formulated as a standard quality spray-dried product that was part of a bulk production composed primarily of proteins (63.05%), carbohydrates (19%), lipids (4.48%), fibers (3%) and ash (3%). The SP and metformin (Met) powders were suspended in tap water and were administrated orally to each animal using a gavage needle once daily (1 mL).
Blood collection and biochemical analysis
The animals fasted for 12 to 14 h. Then, blood samples were collected from the retro-orbital plexus every 10 days. The blood was centrifuged at 3500 rpm for 15 min at room temperature (25 °C), and the plasma was stored in dry tubes at −20 °C.
Glucose and liver function tests [glutamate pyruvate transaminase (GPT) and glutamate oxaloacetate transaminase (GOT) and alkaline phosphatase (Alk-p)] were performed using SPINREACT diagnostic kits (UAA Ctra, Santa Coloma 7 E 17176 SantEsteve de BAS (GI), Spain) by the automated Random Access Clinical Analyzer PICTUS 200-DIATRON based on the colorimetric method.
To determine whether SP could stimulate the release of insulin, we measured serum insulin levels after the arrest of treatment with SP for 10 days (at day 70) using an ELISA kit (Boehringer Manheim Diagnostic, Mannheim, Germany). Because abnormalities in insulin activity are poorly detected by a single determination of glucose or insulin levels (Laakso Citation1993; American Diabetes Association Citation1998), insulin resistance was evaluated by the homeostasis model assessment estimate of insulin resistance (HOMA-IR) (Matthews et al. Citation1985; Haffner et al. Citation2002) as follows (EquationEquation 9(9)
(9) ):
(9)
(9)
The antioxidant enzyme activities were determined using commercial kits (Randox Laboratories Antrim, UK) for superoxide dismutase (SOD), glutathione peroxidase (GPx), and glutathione reductase (GRx) and total antioxidant status (TAS). The serum TBARS levels were measured as described by Quintanilha et al. (Citation1982).
Organo-somatic index (OSI) and body weight gain (BWG)
Each animal was weighted every 10 days and was sacrificed after 70 days of treatment, and the organs were removed and weighed.(10)
(10)
(11)
(11)
Histopathological analysis
The pancreas and liver were fixed in 10% formaldehyde. After they were embedded in paraffin, they were cut into 3 μm sections and stained with haematoxylin and eosin (H & E) (Gomeri Citation1950). The sections were examined under a light microscope (Leica, Leica Store Miami, Coral Gables, FL) equipped with a camera system (Canon, Tokyo, Japan).
Statistics and data processing
The results are expressed as the mean ± standard deviation (SD). The statistical analyses of the data were conducted using Microsoft Excel software (Microsoft 1 Excel 2010). The statistical significance between the means was analyzed using a Student Test of ANOVA (t-test) from Excel version 2010 (Microsoft Corporation, USA).
Results
Phytochemical screening
Spirulina showed 5.54 ± 0.41 mg EAG/g extract of total polyphenols and 1.82 ± 0.05 mg EQ/g extract of total flavonoids. Phenolic compounds act as scavengers of free radicals; they play a major role in antioxidant activity and in stabilizing lipid oxidation as reported by many studies (Gezer et al. Citation2006; Turkoglu et al. Citation2007).
In this study, we also evaluated the major antioxidant composition of phycobiliproteins and carotenoids from SP. The values obtained for C-PC, PE and APC were 16.54 ± 0.12, 1.34 ± 0.08 and 2.06 ± 0.11%, respectively. The total carotenoid was 3.80 ± 0.20 mg/L.
Free radical scavenging is the general mechanism for antioxidants that inhibit lipid peroxidation in a relatively short time. SP showed high antioxidant activity as determined by the DPPH and ABTS tests. The ABTS test showed higher antioxidant activity than the DPPH test (70.19 and 64.99%, respectively) compared to that of BHT (77.07 and 73.15%, respectively) ().
Table 2. Antioxidant activities of S. platensis extract water.
Relative BWG and organ weight
There was a significant decrease (p < 0.001) in the BWG in groups 2 and 4 (−16.92; −16.83 g, respectively) compared to that of the controls (+55.78 g) as shown in . There was a smaller (p < 0.001) weight gain in G5 (+30.13 g) compared to that of the controls, while a similar BWG was observed in G3 (42.42 g) for the rats treated with SP. Notably, SP treatment outperformed the Met treatment in alloxan-treated rats (G5 vs. G4). Similarly, the relative organ weight was monitored. We noticed a significant increase (p < 0.001) in the OSI (liver, left kidney) in groups 2 and 4 compared to that of the untreated group. However, the diabetic group treated with SP did not show any substantial changes.
Table 3. Effects of S. platensis on body weight, body weight gain, and relative weight liver and left kidney [% body weight].
Biochemical parameters
Blood glucose level (BGL)
The antihyperglycemic effect of SP in the diabetic rats induced by alloxan injections was indicated by an improvement in the fasting BGL, an important parameter for monitoring diabetes in addition to the plasma insulin levels as shown in and , respectively.
Figure 1. Effect of S. platensis administration on blood glucose level. NC: Normal Control; DC: Diabetic Control; SPC: SP control; D + Met: Diabetic rats treated with metformin; D + SP: Diabetic rats treated with SP; MetC: Metformin Control. Each value represents mean ± SE (n = 8). ‡p < 0.001, compared with group 1 values, ***p < 0.001, compared with group 2 values, (c) p < 0.001, compared the same group before and after therapy arrest (60th and 70th day) values.

Figure 2. Effect of S. platensis administration on blood insulin level and HOMA-IR. NC: Normal Control; DC: Diabetic Control; SPC: SP control; D + Met: Diabetic rats treated with metformin; D + SP: Diabetic rats treated with SP; Met C: Metformin Control. Each value represents mean ± SE (n = 8). ‡p < 0.001, compared with group 1 values, *p < 0.05; **p < 0.01, ***p < 0.001 compared with group 2 values at the end of experiment.

Hyperglycaemia induced by alloxan ranged from 4.05 to 4.28 g/L. SP treatment (G5) resulted in a significant 56% reduction (p < 0.001) in BGL, which varied from 4.28 to 1.87 g/L in the 50 days of therapy. The BGL of G4, varying from 4.26 to 2.70 g/L, decreased significantly (p < 0.001) by 36%. The BGL assessments were performed every 10 days over the 50 days of treatments. Ten days later, after stopping the treatment, the BGL was monitored again. In this case, the BGL of the diabetic-treated rats (G5) did not increase but continued to decrease by 23%. However, the diabetic rats in G4, which were treated with Met, showed a non-significant increase (7%) ().
Serum insulin levels decreased significantly (p < 0.001) to 7.65 μU/mL in the diabetic group compared to those of the normal control (22.12 μU/mL). However, SP and Met administration increased the levels (p < 0.001) to near normal values compared to those of G2 [41% (G5) against 37% (G4)] during the 70 days. In the normal treated rats, there was a slight increase compared to the controls in the insulin levels after treatment with SP. Thus, SP has potent antihyperglycemic activity. Furthermore, this alga enhanced insulin levels in diabetic rats.
Oxidative stress enzymes and liver function profile
The effect of SP on serum oxidative stress and hepatic function is shown in . Diabetes induced by alloxan decreased the oxidative stress parameters very significantly (p < 0.001) in comparison to those of the controls after 70 days. Administration of SP and Met significantly increased TAS (43%; p < 0.01 and 17%; p < 0.05), GRx (16%; p < 0.01 and 3%; p < 0.01), SOD (48%; p < 0.001and 25%; p < 0.01) and GPx (37%; p < 0.001 and 22%; p < 0.01) in groups 4 and 5, respectively. In contrast, the serum TBARS levels increased significantly (p < 0.001) in G2 compared to those G1. Administration of SP decreased the TBARS levels by 37% in G5, but no significant changes in the serum TBARS levels (5%) were observed in G4. The G2 rats showed a very significant (p < 0.001) increase in AST, ALT, and Alk-p after injection of alloxan. However, SP decreased these effects (p < 0.001) by 25, 36 and 20%, respectively.
Table 4. Effect of S. platensis on serum oxidative stress and liver function levels.
Histopathology
Histopathological analyses of the pancreas () and liver () are shown in . Normal rats (G1) had no structural changes in the pancreas. The pancreases of the diabetic rats (G2) revealed cell necrobiosis and a reduction in islet size. Marine SP-treated normal rats (G3) had large endocrine cells with granular cytoplasm, high eosinophil levels and clear vesicular nuclei, which indicate nucleoprotein synthesis (clumping of chromatin). Group 4 had small islets of Langerhans and a reduced number of cells with condensed nuclei with a partial restoration of the damage. However, after treatment with marine SP (G5), the diabetic rats showed a moderately size islets of Langerhans with active nuclei in the cells. This was likely due to an increase in the β-cells, leading to increased insulin production and secretion. Groups 1 and 3 showed normal liver parenchyma, while the liver sections of G2 showed primary degeneration of hepatocytes. Hypertrophy, hepatocyte necrosis and inflammatory infiltrates around the dilated centrilobular veins were observed. The liver sections obtained from the diabetic rats treated with metformin (G4) showed hypertrophy of hepatocytes and inflammatory infiltrates around the central veins and portal spaces, while the liver tissues of the diabetic rats treated with SP (G5) showed hepatocyte restoration with some hepatosteatosis.
Discussion
Extensive studies have assessed the therapeutic benefits of SP on various disease conditions including diabetes, cardiovascular diseases and inflammatory diseases (Iyer et al. Citation2008; Juarez-Oropeza et al. Citation2009; Al-Dhabi Citation2013). SP is a unicellular cyanobacterium that has attracted attention for its high phytonutrient value; it has been consumed as a food for centuries and is currently used as nutraceutical food supplement worldwide (Deng & Chow Citation2010; Abdel-Daim et al. Citation2013).
Alloxan, a β-cytotoxin, induces ‘chemical diabetes’ in animal species by damaging the insulin-secreting cells of the pancreas and alters serum biochemical parameters. The major aim of this study was to assess the antidiabetic roles of SP.
The induction of diabetes by alloxan is associated with a progressive loss of body weight, which could be due to increased muscle wasting or excess breakdown of proteins in the tissues (Chatterjea & Shinde Citation2002). In contrast, oral administration of SP to diabetic rats for 50 days resulted in a significant (p < 0.001) increase in BWG, suggesting that SP substantially improved their general health status and metabolic mechanisms by effective glycaemic control or a reversal of gluconeogenesis (Voltarelli & de Mello Citation2008; Muthuraman et al. Citation2009; Abdel-Daim Citation2014; Yusuf et al. Citation2016).
The diabetic rats in Group 2 showed a considerable relative decrease in liver weight compared to that of the kidney. However, Yadav et al. (Citation2005) and Sophia and Manoharan (Citation2007) attributed the significant reduction in the liver weight of the diabetic animals to enhanced catabolic processes, such as glycogenolysis, lipolysis and proteolysis. The renal hypertrophy or increase in the kidney weight observed in the DC group may be due to increased glucose utilization and the subsequent enhancement in glycogen synthesis, lipogenesis and protein synthesis (Poonam et al. Citation2008; Vallon & Thomson Citation2012).
Glycaemia and insulin
The injection of alloxan induces diabetes within 3 days (> 4 g/L). Oral administration of SP (10% w/v) restored the BGL to the normal range after 50 days. In this study, SP had a more potent effect than that of Met against diabetes as noted in groups 4 and 5. These results corroborate reports in the literature regarding diabetic rats treated with 10 mg/kg b.w. for 30 days and 25, 50 or 100 mg/kg p.o. of SP for 5 or 10 days after the alloxan injection (Muthuraman et al. Citation2009; Joventino et al. Citation2012). The antihyperglycemic effect of SP is believed to be due to either the presence of potent antioxidant bioactive molecules (β-carotene, phycocyanin and others), which increase the insulin secretion from the islet β-cells or promotion of blood glucose transport to the peripheral tissues (El-Baz et al. Citation2013). This antidiabetic effect could also be due to the action of peptides and polypeptides generated by the digestion of the SP proteins (Mani et al. Citation2000). Antioxidant effects of SP supplementation might be due to its high protein, essential amino acids, essential fatty acids, minerals, vitamins, carotenoids and other antioxidant active constituents, which promote growth and maintain health (Sanchez et al. Citation2003; Babadzhanov et al. Citation2004; Mata et al. Citation2010; Alvarenga et al. Citation2011; Abdel-Daim Citation2014).
In the last 10 days of the experiment, a significant reduction was observed (p < 0.001) in the BGL in Group 5. The strong antioxidant activity of the alga might also contribute to this effect by providing protection against the cytotoxic effects of the free radicals that are generated by the alloxan or diabetes itself (Gallo et al. Citation2005; Wadood et al. Citation2007).
Serum insulin levels decrease during diabetes. In the present study, we found that SP reversed the diabetic effects of glycemia and insulinemia. Similar results were reported by Muthuraman et al. (Citation2009). The active fraction may exert antihyperglycemic effects in diabetic rats by increasing the pancreatic secretion of insulin from the existing β-cells. Bansal et al. (Citation1981) reported that the increase in plasma insulin may be attributed to the conversion of proinsulin to insulin, possibly by pancreatic cathepsin B and/or its secretion.
Oxidative stress
The increased TAS, SOD, GRx and GPx activities in the blood may be responsible for the inhibitory effect of SP upon alloxan-induced oxidative stress and the reduced lipid peroxidation level. These results were noted by Abdel-Daim et al. (Citation2015). According to Shyam et al. (Citation2007), supplementation with SP did not cause any significant change in the plasma total antioxidant status, although a trend toward higher values was evident, with the exception of the TBARS. Treatment with SP offered protection through attenuation of lipid peroxidation and decreased production of free-radical derivatives, as evident from the decreased levels of serum MDA and normalization of GSH and SOD levels (Abdel-Daim Citation2014).
This abnormal metabolism leads to an increased generation of ROS (Rajasekaran et al. Citation2006). The diabetogenic effect of alloxan is due to excess production of ROS. Excess ROS result in toxicity in the pancreatic cells, which reduces the synthesis and the release of insulin, and affects various organs, such as the liver, kidney, and the hematopoietic system (Sakurai et al. Citation2001; Sabu et al. Citation2002).
Diabetics and experimental animal models exhibit high oxidative stress due to persistent and chronic hyperglycaemia as well as hyperlipidaemia, which blocks the antioxidative defence system and thus promotes de novo free radical generation (Kamalakannan & Prince Citation2006; Vijayaraj et al. Citation2013).
Plant cell defences against the damaging effects of oxidative stress involve both enzymatic and non-enzymatic components. The enzymatic factors may directly scavenge ROS or, by producing a non-enzymatic antioxidant (as mentioned previously), may play an important role in the cellular response to oxidative stress by reducing certain ROS individually or in synergy (Candan & Tarhan Citation2003; Chen et al. Citation2008; Vo et al. Citation2015). SP supplementation enhanced all altered serum biochemical parameters and antioxidant biomarkers (Abdelkhalek et al. Citation2014).
Liver function and histology
SP might play an important role in prevention and treatment of hepatic, renal and neurological disorders, especially those mediated by oxidative stress (Lu et al. Citation2010; Gad et al. Citation2011; Bhattacharyya & Mehta Citation2012; Abdel-Daim et al. Citation2016). In this study, alloxan injection was harmful and had a negative on the hepatic tissues, which was accompanied by an increase in GOT, GPT and Alk-p enzymes. GOT and GPT are cytosolic marker enzymes, reflecting hepatocellular necrosis (Setorki et al. Citation2010; Urmila et al. Citation2012). In contrast, the administration of SP had beneficial effects on hepatic balance and decreased liver function parameters significantly (p < 0.001) by 25, 36 and 20% for GOT, GPT and Alk-p, respectively, compared to those of DC 10 days after therapy arrest. SP dietary supplementation reduced the serum hepatic biomarkers and offered a good protection and maintained the structural integrity of hepatocellular membrane (Abdel-Daim Citation2014; Abdelkhalek et al. Citation2014). This may be due to the antioxidant activity of Spirulina phycobiliproteins (phycocyanins and allophycocyanins) or phenolic compounds (Nuhu Citation2013; Abdel-Daim et al. Citation2016), whereas the DPPH assay demonstrated that SP has free radical-scavenging activity. Therefore, this alga acts as an anti-hepatotoxicity agent (Abd El-Baky et al. Citation2009; Thomas & Kim Citation2011; Abdel-Daim Citation2014). A histopathological examination revealed that the SP supplement significantly improved the histological architecture of the islets of Langerhans and the liver of diabetic animals. Makhlouf and Makhlouf (Citation2012), Tobon-Velasco et al. (Citation2013) and Abdel-Daim et al. (Citation2015) reported that the SP supplement had free radical scavenging activity and reduced various indicators of toxicity such as tissue damage in rats. In vitro and in vivo studies have shown that the antioxidant components produced by SP prevent or delay oxidative damage by reducing the accumulation of ROS (Zhang et al. Citation2011) through the activation of the antioxidant enzyme systems of catalase, SOD, and GPx (Thaakur & Jyothi Citation2007; Abdel-Daim Citation2014). In accordance with our results, it was proven that the antioxidant properties of SP contributed to its beneficial effect in treating various pathological conditions.
Conclusion
Spirulina extract effectively alleviated the abnormal biochemical parameters, especially glycaemia and insulinemia. It may be used as a potent phytomedicine for diabetes alone or in combination with other treatments. Spirulina also reversed damage to the liver and the oxidative stress observed in diabetic animals. Furthermore, even if after therapy stopped, the SP efficacy maintained all of the studied parameters in a normal range, especially glycaemia. Other investigations are needed to elucidate the exact mechanism of action of the SP or its extract.
Disclosure statement
The authors declare no conflicts of interest.
Additional information
Funding
References
- Abd El-Baky HH, El Baz FK, El-Baroty GS. 2009. Production of phenolic compounds from Spirulina maxima microalgae and its protective effects. Afr J Biotechnol. 8:7059–7067.
- Abdel-Daim M, El-Bialy BE, Rahman HG, Radi AM, Hefny HA, Hassan AM. 2016. Antagonistic effects of Spirulina platensis against sub-acute deltamethrin toxicity in mice: biochemical and histopathological studies. Biomed Pharmacother. 77:79–85.
- Abdel-Daim M, Halawa S. 2014. Synergistic hepatocardioprotective and antioxidant effects of myrrh and ascorbic acid against diazinon-induced toxicity in rabbits. Int Res J Humanit Eng Pharm Sci. 1:1–7.
- Abdel-Daim MM. 2014. Pharmacodynamic interaction of Spirulina platensis with erythromycin in Egyptian Baladi bucks (Capra hircus). Small Rumin Res. 120:234–241.
- Abdel-Daim MM, Abuzead SMM, Halawa SM. 2013. Protective role of Spirulina platensis against acute deltamethrin-induced toxicity in rats. PLoS ONE. 8:e72991.
- Abdel-Daim MM, Farouk SM, Madkour FF, Azab SS. 2015. Anti-inflammatory and immunomodulatory effects of Spirulina platensis in comparison to Dunaliella salina in acetic acid-induced rat experimental colitis. Immunopharm Immunot .37:126–139.
- Abdelkhalek NK, Ghazy EW, Abdel-Daim MM. 2014. Pharmacodynamic interaction of Spirulina platensis and deltamethrin in freshwater fish Nile tilapia, Oreochromis niloticus: impact on lipid peroxidation and oxidative stress. Environ Sci Pollut Res Int. 22:3023–3031.
- Al-Dhabi NA. 2013. Heavy metal analysis in commercial Spirulina products for human consumption. Saudi J Biol Sci. 20:383–388.
- Alvarenga RR, Rodrigues PB, Cantarelli VS, Zangeronimo MG, Júnior JWS, Silva LR, Santos LM, Pereira LJ. 2011. Energy values and chemical composition of Spirulina (Spirulina platensis) evaluated with broilers. Rev Bras Zootec. 40:992–996.
- American Diabetes Association. 1998. Consensus Development Conference on Insulin Resistance (ADACDCIR). Diabetes Care. 21:310–314.
- Anamika P, Sandhya M, Richa P, Ghosh PK. 2005. Purification and characterization of C-Phycocyanin from cyanobacterial species of marine and freshwater habitat. Protein Express Purif. 40:248–255.
- Babadzhanov AS, Abdusamatova N, Yusupova FM, Faizullaeva N, Mezhlumyan LG, Malikova MK. 2004. Chemical composition of Spirulina platensis cultivated in Uzbekistan. Chem Nat Compd. 40:276–279.
- Bansal R, Ahmad N, Kidwai JR. 1981. Effect of oral administration of Eugenia jambolana seeds and chlorpropamide on blood glucose level and pancreatic cathepsin B in rats. Indian J Biochem Biophys. 18:377–381.
- Bennett A, Bogorad L. 1973. Complementary chromatic adaptation in a filamentous blue-green alga. J Cell Biol. 58:419–435.
- Bhattacharyya S, Mehta P. 2012. The hepatoprotective potential of Spirulina and vitamin C supplemention in cisplatin toxicity. Food Funct. 3:164–169.
- Bos M, Agyemang C. 2013. Prevalence and complications of diabetes mellitus in Northern Africa, a systematic review. BMC Public Health. 13:1–7.
- Burits M, Bucar F. 2000. Antioxidant activity of Nigella sativa essential oil. Phytother Res. 14:323–328.
- Candan N, Tarhan L. 2003. Relationship among chlorophyll-carotenoid concentration, antioxidant enzyme activities and lipid peroxidation levels by Mg2+deficiency in the Mentha pulegium leaves. Plant Physiol Biochem. 41:35–40.
- Chatterjea MN, Shinde R. 2002. Textbook of Medical Biochemistry. New Delhi, India: Jaypee Brothers Medical Publishers.
- Chen TF, Zheng WJ, Wong YS, Yang F. 2008. Selenium-induced changes in activities of antioxidant enzymes and content of photosynthetic pigments in Spirulina platensis. J Integr Plant Biol. 50:40–48.
- Chu WL, Lim YW, Ammu KRAK, Lim PE. 2010. Protective effect of aqueous extract from Spirulina platensis against cell death induced by free radicals. BMC Complement Altern Med. 10:1–8.
- Deng R, Chow TJ. 2010. Hypolipidemic, antioxidant, and antiinflammatory activities of microalgae Spirulina. Cardiovasc Ther. 28:e33–e45.
- El-Baz F, Aly HF, El-Sayed AB, Mohamed AA. 2013. Role of Spirulina platensis in the control of glycemia in DM2 rats. Int J Sci Eng Res. 4:1731–1740.
- Gad AS, Khadrawy YA, El-Nekeety AA, Mohamed SR, Hassan NS, Abdel-Wahhab MA. 2011. Antioxidant activity and hepatoprotective effects of whey protein and Spirulina in rats. Nutrition. 27:582–589.
- Gallo A, Ceolotto G, Pinton P, Iori E, Murphy E, Rutter AG, Rizzuto R, Semplicini A, Avogaro A. 2005. Metformin prevents glucose-induced protein kinase C-β2 activation in human umbilical vein endothelial cells through an antioxidant mechanism. Diabetes. 54:1123–1131.
- Gezer K, Duru E, Kivrak Turkaglu A, Mercan N, Turkoglu H, Gukan S. 2006. Free radical scavenging capacity and antimicrobial activity of wild edible mushroom from Turkey. Afr J Biotechnol. 5:1924–1928.
- Göksan T, Kılıc¸ C. 2009. Growth and biochemical composition of Spirulina platensis Geitler in summer period under the conditions of C¸anakkale, Turkey. Asian J Chem. 21:4947–4950.
- Gomeri G. 1950. Aldehyde-fuchsin, a new staining for elastic tissue. Am J Pathol. 17:395–406.
- Ha H, Kim KH. 1999. Pathogenesis of diabetic nephropathy: the role of oxidative stress and protein kinase C. Diabetes Res Clin Pract. 45:147–151.
- Haffner SM, Greenberg AS, Weston WM, Chen H, Williams K, Freed MI. 2002. Effect of rosiglitazone treatment on nontraditional markers of cardiovascular disease in patients with type 2 diabetes mellitus. Circulation. 106:679–684.
- Ibrahim A, Abdel-Daim M. 2015. Modulating effects of Spirulina platensis against tilmicosin-induced cardiotoxicity in mice. Cell J (Yakhteh). 17:137–144.
- Iyer UM, Dhruv SA, Mani IU. 2008. Spirulina and its therapeutic implications as a food product. In: Gershwin ME, Belay A, editors. Spirulina in human nutrition and health. Boca Raton: Taylor & Francis Group; New York: CRC Press, p. 51–70.
- Juarez-Oropeza MA, Mascher D, Torres-Duran PV, Farias JM, Paredes- Carbajal MC. 2009. Effects of dietary Spiurlina on vascular reactivity. J Med Food. 12:15–20.
- Joventino IP, Alves HGR, Neves LC, Pinheiro-Joventino F, Leal LKAM Neves SA, Ferreira FV, Brito GAC, Viana GB. 2012. The microalga Spirulina platensis presents anti-inflammatory action as well as hypoglycemic and hypolipidemic properties in diabetic rats. J Complement Integr Med. 9:1553–3840.
- Kamalakannan N, Prince PSM. 2006. Antihyperglycaemic and antioxidant effect of rutin, a polyphenolic flavonoid, in streptozotocin-induced diabetic Wistar rats. Basic Clin Pharmacol Toxicol. 98:97–103.
- Kameswara Rao B, Kesavulu MM, Giri R, AppaRao C. 1999. Antidiabetic and hypolipidemic effects of Momordica cymbalaria Hook. fruit powder in alloxan-diabetic rats. J Ethnopharmacol. 67:103–109.
- Khan Z, Bhadouria P, Bisen P. 2005. Nutritional and therapeutic potential of Spirulina. Curr Pharm Biotechnol. 6:373–379.
- Laakso M. 1993. How good a marker is insulin level for insulin resistance? Am J Epidemiol. 137:959–965.
- Lamaison JLC, Carnet A. 1990. Teneurs en principaux flavonoids des fleurs de Crataegeus monogyna Jacq et de Crataegeus laevigata (Poiret D. C) en function de la vegetation. Pharm Acta Helv. 65:315–320.
- Lichtenthaler H, Wellburn A. 1985. Determination of total carotenoids and chlorophylls A and B of leaf in different solvents. Biochem Soc Trans. 11:591–592.
- Lu J, Ren DF, Wang JZ, Sanada H, Egashira Y. 2010. Protection by dietary Spirulina platensis against D-galactosamine-and acetaminophen-induced liver injuries . Br J Nutr. 103:1573–1576.
- Makhlouf R, Makhlouf I. 2012. Evaluation of the effect of Spirulina against gamma irradiation-induced oxidative stress and tissue injury in rats. Int J Appl Sci Eng Res. 1:152–164.
- Mani UV, Desai S, lyer UM. 2000. Studies on long term effect of Spirulina supplementation on serum lipid profile and glycated proteins in NIDDM patients. J Nutraceut. 2:25–32.
- Mata TM, Martins AA, Caetano NS. 2010. Microalgae for biodiesel production and other applications: a review. Renew Sust Energy Rev. 14:217–232.
- Matthews DR, Hosker JP, Rudenski AS, Naylor BA, Treacher DF, Turner RC. 1985. Homeostasis model assessment: insulin resistance and beta-cell function from fasting plasma glucose and insulin concentrations in man. Diabetologia. 28:412–419.
- Muthuraman P, Senthilkumar R, Srikumar K. 2009. Alterations in beta-islets of Langerhans in alloxan-induced diabetic rats by marine Spirulina platensis. J Enzyme Inhib Med Chem. 24:1253–1256.
- Nuhu AA. 2013. Spirulina (Arthrospira): an important source of nutritional and medicinal compounds. J Mar Biol. 2013:1–8.
- Oliveira ACP, Endringer DC, Amorim LAS, Brandao MGL, Coelho MM. 2005. Effect of the extracts and fractions of Baccharis trimera and Syzygium cumini on glycaemia of diabetic and non-diabetic mice. J Ethnopharmacology. 102:465–469.
- Quintanilha AT, Packer L, Davies JM, Racanelli TL, Davies KJ. 1982. Membrane effects of vitamin E deficiency: bioenergetic and surface charge density studies of skeletal muscle and liver mitochondria. Ann N Y Acad Sci. 393:32–47.
- Pankaj PP, Varma MC. 2013. Potential role of Spirulina platensis in maintaining blood parameters in alloxan-induced diabetic mice. Int J Pharm Pharm Sci. 5:450–456.
- Poonam S, Prachi A, Murali YK, Tandon V. 2008. Antidiabetic activity of 50% ethanolic extract of Ricinus communis and its purified fractions. Food Chem Toxicol. 46:3458–3466.
- Rajasekaran S, Kasiappan R, Karuran S, Sorimutha S. 2006. Beneficial effects of Aloe vera leaf gel extract on lipid profile status in rats with streptozotocin diabetes. Clin Exp Pharmacol Physiol. 33:232–237.
- Re R, Pellegrini N, Proteggente A, Pannala A, Yang M, Rice-Evans C. 1999. Antioxidant activity applying an improved ABTS radical cation decolorization assay. Free Radic Biol Med. 26:1231–1237.
- Sabu MC, Smitha K, Kuttan R. 2002. Anti-diabetic activity of green tea polyphenols and their role in reducing oxidative stress in experimental diabetes. J Ethnopharmacol. 83:109–116.
- Sakurai K, Katoh M, Someno K, Fujimoto Y. 2001. Apoptosis and mitochondrial damage in INS-1 cells treated with alloxan. Biol Pharm Bull. 24:876–882.
- Sanchez M, Bernal-Castillo J, Rozo C, Rodriguez I. 2003. Spirulina (Arthrospira): an edible microorganism. A Review Univ Sci. 8:7–24.
- Setorki M, Asgary S, Eidi A, Haeri Rohani A, Khazaei M. 2010. Acute effects of vinegar intake on some biochemical risk factors of atherosclerosis in hypercholesterolemic rabbits. Lipids Health Dis. 28:1–10.
- Shyam R, Singh SN, Vats P, Singh VK, Pharma D, Bajaj R, Singh SB, Banerjee PK. 2007. Wheat grass supplementation decreases oxidative stress in healthy subjects: a comparative study with Spirulina. J Altern Complement Med. 13:789–791.
- Singleton VL, Orthofer R, Lamuela-Raventos RM. 1999. Analysis of total phenols and other oxidation substrates and antioxidants by means of Folin-Ciocalteu reagent. Methods Enzymol. 299:152–178.
- Sophia D, Manoharan S. 2007. Hypolipidemic activities of Ficus racemosa Linn. bark in alloxan induced diabetic rats . Afr J Tradit Complement Altern Med. 4:279–288.
- Thaakur SR, Jyothi B. 2007. Effect of Spirulina maxima on the haloperidol induced tardive dyskinesia and oxidative stress in rats. J Neural Transm (Vienna). 114:1217–1225.
- Thormar H. 2012. Patented non-antibiotic agents as animal feed additives. Recent Pat Food Nutr Agric. 4:155–168.
- Tobon-Velasco JC, Palafox-Sanchez V, Mendieta L, García E, Santamaría A, Chamorro-Cevallos G, Limón ID. 2013. Antioxidant effect of Spirulina (Arthrospira) maxima in a neurotoxic model caused by 6-OHDA in the rat striatum. J Neural Transm. 120:1179e–11189.
- Thomas NV, Kim SK. 2011. Potential pharmacological applications of polyphenolic derivates from marine brown algae. Environ Toxicol Pharmacol. 32:325–335.
- Turkoglu A, Duru ME, Mercan N, Kivrak I, Gezer K. 2007. Antioxidant and antimicrobial activities of Laetiporus sulphureus (Bull.). Murill Food Chem. 101:267–273.
- Urmila J, Anish ZJ, Pravin K, Bisen PS, Prasad GBKS. 2012. Alleviation of metabolic abnormalities induced by excessive fructose administration in Wistar rats by Spirulina maxima. Indian J Med Res. 135:422–428.
- Vallon V, Thomson SC. 2012. Renal function in diabetic disease models: the tubular system in the pathophysiology of the diabetic kidney. Annu Rev Physiol. 74:1–27.
- Vijayaraj P, Muthukumar K, Sabarirajan J, Nachiappan V. 2013. Antihyperlipidemic activity of Cassia auriculata flowers in Triton WR 1339 induced hyperlipidemic rats. Exp Toxicol Pathol. 65:135–141.
- Vo TS, Ngo DH, Kim SK. 2015. Nutritional and pharmaceutical properties of microalgal Spirulina. In: Kim SK, editors. Handbook of Marine Microalgae. Biotechnology Advances. Elsevier Science, Academic Press, p. 299–308.
- Voltarelli FA, de Mello MAR. 2008. Spirulina enhanced the skeletal muscle protein in growing rats. Eur J Nutr 47:393–400.
- Wadood N, Nisar M, Rashid A, Wadood A, Nawab G, Khan A. 2007. Effect of a compound recipe (medicinal plants) on serum insulin levels of alloxan-induced diabetic rabbits. J Ayub Med Coll Abbottabad. 19:32–38.
- Wild S, Bchir MB, Roglic G, Green A, Sicree R, King H. 2004. Global prevalence of diabetes: Estimates for the year 2000 and projections for 2030. Diabetes Care. 27:1047–1053.
- Yadav UC, Moorthy K, Baquer NZ. 2005. Combined treatment of sodium orthovanadate and Momordica charantia fruit extract prevents alterations in lipid profile and lipogenic enzymes in alloxan diabetic rats. Mol Cell Biochem. 268:111–120.
- Yang L, Zhang LM. 2009. Chemical structural and chain conformational characterization of some bioactive polysaccharides isolated from natural sources. Carbohyd Polym. 76:349–361.
- Yusuf MS, Hassan MA, Abdel-Daim MM, El Nabtiti AS, Ahmed AM, Moawed SA, El-Sayed AK, Cui H. 2016. Value added by Spirulina platensis in two different diets on growth performance, gut microbiota, and meat quality of Japanese quails. Vet World. 9:1287–1293.
- Zhang BB, Moller DE. 2000. New approaches in the treatment of type 2 diabetes. Curr Opin Chem Biol. 4:461–467.
- Zhang H, Chen T, Jiang J, Wong YS, Yang F, Zheng W. 2011. Selenium-containing allophycocyanin purified from selenium-enriched Spirulina platensis attenuates AAPH-induced oxidative stress in human erythrocytes through inhibition of ROS generation. J Agric Food Chem. 59:8683–8690.
- Zheng J, Inoguchi T, Sasaki S, Maeda Y, McCarty MF, Fujii M, Ikeda N, Kobayashi K, Sonoda N, Takayanagi R. 2013. Phycocyanin and phycocyanobilin from Spirulina platensis protect against diabetic nephropathy by inhibiting oxidative stress. Am J Physiol Regul Integr Comp Physiol. 304:110–120.