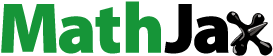
Abstract
Context: Buchenavia tetraphylla (Aubl.) RA Howard (Combretaceae: Combretoideae) is an ethnomedicinal plant with reported antifungal action.
Objective: This study evaluates the antimicrobial activity of B. tetraphylla leaf extracts against clinical isolates of Candida albicans. The morphological alterations, combinatory effects with fluconazole and the cytotoxicity of the active extract were analyzed.
Materials and methods: Extracts were obtained using different solvents (hexane: BTHE; chloroform: BTCE; ethyl acetate: BTEE; and methanol: BTME). Antimicrobial activity was determined by the broth microdilution method using nine strains of C. albicans isolated from vaginal secretions and one standard strain (UFPEDA 1007).
Results: All extracts showed anti-C. albicans activity, including against the azole-resistant strains. The MIC values ranged from 156 to 2500 μg/mL for the BTHE; 156 to 1250 μg/mL for the BTCE; 625 to 1250 μg/mL for the BTME and 625 μg/mL to 2500 μg/mL for the BTEE. BTME showed the best anti-C. albicans activity. This extract demonstrated additive/synergistic interactions with fluconazole. Scanning electron microscopy analysis suggested that the BTME interferes with the cell division and development of C. albicans. BTME showed IC50 values of 981 and 3935 μg/mL, against J774 macrophages and human erythrocytes, respectively. This extract also enhanced the production of nitric oxide by J774 macrophages.
Discussion and conclusion: Buchenavia tetraphylla methanolic extract (BTME) is a great source of antimicrobial compounds that are able to enhance the action of fluconazole against different C. albicans strains; this action seems related to inhibition of cell division.
Introduction
Candida albicans is a polymorphic fungus recognized as one of the most common fungal pathogens, responsible for a range of diseases (Mayer et al. Citation2013). This yeast is usually found in the normal microbiota of several distinct anatomical sites such as skin, mouth and genitourinary and digestive systems (Pfaller & Diekema Citation2010; Gulati & Nobile Citation2016). However, some adverse environmental conditions such as pH shift, nutritional deficiency, use of antibiotics or variations in the immune status of the host, can enable this microorganism to cause infections, which range from superficial dermal and mucosal cases to more severe systemic infections (candidemia and invasive candidiasis, frequently associated with patients with compromised immunity). Several factors, related to both host and pathogen, are recognized as crucial for infection development; for example, C. albicans possesses a range of virulence factors, associated to adherence, filamentation, biofilm formation and secretion of proteases. Additionally, antibiotic and immunosuppressive therapies, diseases (human immunodeficiency virus, diabetes), and aging are also factors that favour C. albicans infections (Gow & Hube Citation2012; Mayer et al. Citation2013; Fadda et al. Citation2015).
The treatment of C. albicans infection is based on the use of systemic antifungals (fluconazole or itraconazole) and topical nystatin (Pappas et al. Citation2015). An increase in the resistance of C. albicans to antifungals has been observed in recent decades, which makes this yeast a serious concern for the global health system. Indeed, the development of new and effective approaches to treat fungal infections remains one of the major challenges for modern medicine (Sardi et al. Citation2013).
Traditional medicinal plants from underexploited environments, such as the Caatinga (semi-arid region), an exclusively Brazilian biome, have been the subject of various studies in the search for new antimicrobial compounds, due to their exceptional activity against microorganisms (Oliveira et al. Citation2012; Silva et al. Citation2014). Buchenavia tetraphylla (Aubl.) RA Howard (Combretaceae: Combretoideae) is a Neotropical plant, distributed from Cuba (Caribbean) to Brazil (South America) (Weaver Citation1991). In Brazil, this plant is popularly known as ‘Tanimbuca’ and is listed as an ethnomedicinal plant used by the traditional communities of northeastern Brazil (Agra et al. Citation2007, Citation2008). Our group has shown that extracts and fractions of B. tetraphylla leaves have a broad-spectrum of antimicrobial activities, inhibiting both Gram-positive and Gram-negative bacteria and fungi (including C. albicans) (Oliveira et al. Citation2012). Additionally, a recent study has shown that B. tomentosa had a potential antifungal effect against different Candida species (Teodoro et al. Citation2015). In this context, this study aimed to evaluate the antimicrobial activity of B. tetraphylla extracts alone or in combination with fluconazole against clinical isolates of C. albicans isolated from vaginal secretions, and to analyze the ultrastructural changes and the combinatory effect with fluconazole induced by the active extract.
Materials and methods
Plant material: collection, identification and extraction
Leaves of B. tetraphylla were collected in the Parque Nacional do Catimbau (PARNA do Catimbau, Pernambuco, Brazil) in November of 2013. The taxonomic identification was performed by Dr Alexandre Gomes da Silva, at the Herbarium of the Instituto Agronômico de Pernambuco (IPA), where the voucher specimen (Number 84.104) was deposited. Leaves were dried using an incubator at 45 °C for 2 to 3 days. The dried material was ground into powder form using a grinder followed by a Waring blender.
For extraction, dried leaves (25 g) were mixed with hexane (100 mL) in a shaker at 125 rpm for 72 h at 25 °C. Then, the extract was filtered and the supernatant was concentrated in a rotary vacuum. The residue was suspended in 100 mL of chloroform. The extraction procedure was repeated and the residue was resuspended in 100 mL of ethyl acetate. Finally, the residue was subjected to methanol extraction. All extract samples were diluted in 100% dimethyl sulfoxide and stored at 4 °C prior to use.
Phytochemical analysis
The extracts from B. tetraphylla were submitted to thin-layer chromatography (TLC) assays in order to perform a qualitative detection of their functional compounds. The extracts were loaded in silica gel F254 + 366 plates (20 × 20 cm; <AQ5>), and developed in a solvent system: A (toluene/dioxan/acetic acid, 180:45:5, v/v) and B (hexane/diethyl ether/formic acid, 130:80:20, v/v). Bands were revealed under UV light (254/365 nm), using ceric sulphate and ferric chloride. The bands were identified by their Rf values and compared with the standards used.
Antimicrobial assays
Candida albicans strains
A total of 10 C. albicans strains were used in this study. The standard strain (UFPEDA1007) was obtained from the Microbial Collection of the Departamento de Antibióticos, Universidade Federal de Pernambuco (UFPEDA). The clinical strains were isolated from vaginal secretions at different clinical laboratories in Recife (Pernambuco, Brazil) and kindly provided by them between September and December 2012.
The antibiotic-susceptibility profile of each isolate was performed according to the CLSI recommendations (CLSI Citation2011) using the disc-diffusion assay on Sabouraud dextrose agar (SDA). In brief, colonies from overnight cultures of C. albicans were suspended in sterile saline water equivalent to a 0.5 McFarland standard. The suspension (100 μL) was spread over an SDA plate and the antibiotic disc was applied aseptically onto the surface. Afterwards, the plates were incubated at 30 °C for a period of 48 h and then each inhibition diameter zone (IDZ) was measured and interpreted as shown in . The antibiotics used were amphotericin B, fluconazole, itraconazole and ketoconazole.
Table 1. Antibiotic-resistance profile of C. albicans clinical strains isolated from vaginal secretion samples.
The multiple antibiotic resistance (MAR) index was calculated as previously described by Krumperman (Citation1983) using the formula MAR = x/y, where ‘x’ is the number of antibiotics to which the isolate demonstrated resistance; and ‘y’ is the total number of antibiotics tested.
Determination of minimal inhibitory concentration (MIC) and minimum fungicidal concentration (MFC)
The minimal inhibitory concentration (MIC) was determined by the microdilution method (CLSI Citation2011). Twofold serial dilution of each extract was prepared in Sabouraud broth and 10 μL of yeast suspension (approximately 1.5 × 108 CFU/mL) were added. The microplates were incubated at 30 °C. After 48 h, 50 μL of resazurin solution (0.01%) were added to each well. The plates were re-incubated for 2 h at 30 °C, and any colour changes from purple to pink was recorded as microbial growth. The lowest concentration at which no colour change occurred was taken as the MIC. Afterwards, cultures were seeded in SDA plates and incubated for 48 h at 30 °C to determine the minimum fungicidal concentration (MFC).
Combinatory effects of B. tetraphylla methanolic extract and fluconazole
The combinatory effects of the BTME and fluconazole were evaluated against five C. albicans strains (F03, F08, F11, F22, 1007). Both samples were added to microplates containing SAB and a twofold serial dilution was prepared (5000 to 98 μg/mL for BTME and 100 to 0.2 μg/mL for fluconazole). The antimicrobial action was evaluated as described for MIC, and after 48 h, the drug/extract interaction was assessed algebraically by determining the Fractional Inhibitory Concentration indices (ΣFIC), according to the following equation:
MICE or MICF: the MIC extract or MIC fluconazole; MICF+D: the MICE when in combination with fluconazole; MICD+F: the MIC of fluconazole when in combination with extract; Data interpretation: ΣFIC ≤0.5: synergism (syn); 0.5 < ΣFIC ≤1: addition (add); 1 < ΣFIC < 4: non-interaction (non); ΣFIC ≥4: antagonism (ant) (Vuuren & Viljoen Citation2011).
Scanning electron microscopy (SEM)
To evaluate the morphological changes induced by the BTME extract, a sample of 100 μL of a diluted overnight culture of C. albicans (approximately 1.5 × 108 CFU/mL) was added to 1 mL of SAB and mixed with the BTME (MIC). After incubation (12 h at 30 °C) the cells were treated as described by da Silva et al. (Citation2013) and imaged with a Quanta 200 F scanning electron microscope (FEI company).
Determination of nitric oxide production and cell viability in macrophages
J774 macrophages (1 × 105 cells/mL) were seeded in a 96-wells plate for 24 h at 37 °C and 5% CO2 and then treated with the BTME (156–2500 μg/mL) for another 24 h. After the treatment, the supernatant was used to determine nitric oxide (NO) production, and the adherent cells were used for viability assays. For NO determination, the supernatant (100 μL) from each well was mixed with 100 μL of Griess reagent in a 96-wells plate. After incubation for 15 min at room temperature, the optical density was determined at 540 nm with a microplate reader (Benchmark plus, Bio-Rad, Hercules, CA). The nitrite content (μM/106 cells) was quantified by extrapolation from sodium nitrite standard curve in each experiment. Cell viability was evaluated using the MTT assay, which measures the metabolic conversion of the 3-(4,5-dimethylthiazol-2-yl)-2,5-diphenyltetrazolium bromide (MTT) salt by mitochondria of viable cells, by the use of coloured formazan dye. At the end of the incubation, the medium was removed and an MTT solution (5000 μg/mL in RPMI) was added to the cells that were further incubated for 3 h. Afterwards, the medium was removed and the intracellular formazan product was dissolved in DMSO. Optical density (OD) was measured at 595 nm. Cell viability was calculated in comparison to the OD obtained by untreated cells.
Haemolytic assay
Blood (5–10 mL) samples were obtained from healthy volunteers by venipuncture and placed in heparinized tubes, after written informed consent was obtained. Human erythrocytes were isolated by centrifugation (1500 rpm for 10 min) and washed three times with phosphate-buffered saline (PBS; pH 7.4). Each test tube received 1.1 mL of erythrocyte suspension (1%) and 0.4 mL of the various extracts at different concentrations (156–2500 μg/mL). Cells incubated in PBS or in a solution of saponin from Quillaja sp. (0.0025%) were used as negative and positive controls for haemolytic activity, respectively. After 60 min of incubation, cells were centrifuged and the absorbance of supernatant was read at 540 nm. The haemolytic activity was expressed by the following formula:
Ab = solvent absorbance, As = sample absorbance; Ac =saponin absorbance.
Statistical analysis
All experiments were performed in quadruplicate with at least two independent experiments. Results are expressed as mean ± standard deviation (SD). Statistical analyses were performed by Student's t test. All analyses were carried out using the Statistica 8.0 software. Differences were considered significant at p < 0.05. The correlation indices were calculated using the Pearson coefficient (ρ).
Results
Phytochemical analysis
The phytochemical profile of extracts from leaves of B. tetraphylla is shown in . The results showed that all extracts have at least one class of compound reported as an antimicrobial agent. The hexane extract (BTHE) presented hydrocarbons, glycosides, sugar and terpenes. Flavonoids, terpenes and tannins were detected in the ethyl acetate extract (BTEE), while only terpenes and sugar were found in the chloroform extract (BTCE). Finally, flavonoids and tannins were detected in the methanol extract (BTME).
Table 2. Phytochemical analysis of B. tetraphylla leaves extracts.
Antibiotic susceptibility of C. albicans strains
All clinical isolates of C. albicans tested in this study had their antibiotic-susceptibility profile analyzed (). According to the disc-diffusion assay, two strains (F03 and F23) were susceptible to all tested antifungal agents; another three isolates (F14, F23 and F27) were susceptible to amphotericin B and ketoconazole and showed intermediate susceptibility to fluconazole and itraconazole; the strain F01 was only resistant to amphotericin B; finally, the strains F02, F08 and F11 showed resistance to fluconazole with cross-resistance to itraconazole (MAR index of 0.5). Fluconazole resistance of each strain was confirmed by MIC determination as recommend by CLSI (CLSI Citation2011). Strains F03, F08 and 1007 were inhibited by fluconazole at 3.125 μg/mL, while strains F11 and F22 were inhibited by the same drug at 6.25 μg/mL.
Anti-C. albicans action of B. tetraphylla extracts
The inhibitory action of B. tetraphylla extracts was evaluated against 10 C. albicans strains (). The extracts showed anti-C. albicans activity against all tested strains, including the azole-resistant strains (F02, F08 and F11). The MIC values ranged from 156 to 2500 μg/mL for the BTHE; 156 to 1250 μg/mL for the BTCE; 625 to 1250 μg/mL for the BTME and 625 μg/mL to 2500 μg/mL for the BTEE. The BTME and BTCE showed the best antimicrobial potential with no significant difference between their average MFC values (p > 0.05). A moderate negative correlation was observed between the MAR indexes of all strains and MIC (ρ = −0.39) or MFC (ρ = −0.33) values of the BTME, indicating this extract was slightly more active against the resistant strains. Moderate and weak positive correlations were found between the MAR indexes and MIC (ρ = 0.51) or MFC (ρ = 0.14) values of the BTCE. Additionally, the MFC/MIC ratios ranged from 2 to 4 for the BTME, thus it was a fungicidal agent against all tested strains. Both fungicidal and fungistatic effects were observed for the BTCE (MFC/MIC ratios ranged from 2 to 8), but fungicidal action was more prominent (for nine strains). Since the BTME showed the strongest activity it was chosen for further biological activity assays.
Table 3. The anti-Candida albicans activity of extracts from B. tetraphylla leaves.
Combinatory effects of the BTME and fluconazole
The combinatory effects of the BTME and fluconazole are shown in . The extract had the ability to increase the action of fluconazole in most strains through additive (20% of strains; ΣFIC =0.75) or synergetic (60% of strains; ΣFIC values of 0.375 and 0.5) effects. Non-interaction was observed against one strain (ΣFIC =2).
Morphological changes induced by the BTME
The morphological alterations induced by the BTME in the fluconazole-resistant F08 strain were evaluated using scanning electron microscopy. This strain was chosen because it presents a high degree of cell surface hydrophobicity in vitro (data not shown), a property related to the increase of C. albicans adherence and virulence (Blanco et al., Citation2009; Silva-Dias et al. Citation2015). After 12 h of cultivation, untreated samples () showed a predominance of budding blastoconidial cells with a normal elliptical shape, smooth surface and formation of hyphal structures exhibiting a homogeneous, elongated shape without constrictions (). BTME-treated cells () showed intense deposition of flocculated material on the cell surface, more elongated blastoconidial morphology with several scars (). However, no filamentation could be observed and hyphal structures were not seen in treated samples. Cells presenting depressions on the cell surface could also be observed (). No ruptured cells or alterations on the yeast cell surface were detected.
Figure 2. Effects of BTME on C. albicans. (a–c) Control cells; (d–f) cells treated with BTME at MIC for 12 h. (a–c) Ultrastructural aspects of untreated C. albicans culture at low (a) and high magnification (b–c) showing the presence of single and budding yeast cells with evident fragile blastoconidial septum (white arrow). (h) True hyphal structures could be also observed. Treated C. albicans culture at (d) low and (e–f) high magnifications. Note in (e) the presence of elongated cells (white asterisk) with multiple scars (white arrow). (f) Cells presenting surface depressions are indicated in white arrow.

Effects of the BTME on cell viability and NO production
The treatment of J774 macrophages with different concentrations of the BTME resulted in significant production of NO at concentrations from 312.5 to 2500 μg/mL (p > 0.05), in a dose-dependent manner (). Regarding cytotoxicity, the BTME extract showed IC50 values of 981 μg/mL against J774 macrophages and 3935 μg/mL against erythrocytes.
Discussion
C. albicans is the most prevalent pathogen associated with vulvovaginal candidiasis, and it is estimated that it affects approximately 75% of women at least once during their lifetime. This pathology represents significant health issues for women of childbearing age, as well as diabetic and immuno-deficient patients. Vulvovaginal candidiasis is associated with considerable morbidity, increased healthcare costs, distress, pain and sexual dysfunction (Cassone Citation2014). C. albicans strains isolated from vaginal secretions have shown increased resistance towards antifungal agents, especially fluconazole. It is important to highlight that the breakpoint of in vitro susceptibility for strains isolated from vaginal secretions has been suggested to be 1 μg/mL instead of 8 μg/mL, this is due to the specific pharmacokinetic characteristics of the vagina (Sobel et al. Citation2003; Marchaim et al. Citation2012). In this context, this work evaluated the antifungal action of extracts from leaves of B. tetraphylla against fluconazole-resistant and fluconazole-sensitive strains of C. albicans isolated from human vaginal secretions.
It has been reported that B. tetraphylla leaves are a source of compounds with anti-C. albicans activity (Oliveira et al. Citation2012). Furthermore, Teodoro et al. (Citation2015) found remarkable anti-Candida activity of another member of the Buchenavia genus, B. tomentosa. All the extracts were also effective against the clinical isolates tested. Furthermore, the extraction method used in this present paper increased the antifungal potential of this plant, as the average MIC values of the standard strain were up to 40 times lower than those reported by Oliveira et al. (Citation2012). Among the extracts, the BTME and BTCE showed the best activity and no significant differences were observed between their MIC or MFC values. The BTME is more effective against the fluconazole-resistant strains than the BTCE, as confirmed by correlation analysis (the Pearson coefficient for their MIC values was −0.032; and 0.076 for their MFC values). Two mammalian cell types were used to determine the cytotoxic potential of each extract: human erythrocytes and mice macrophages. Both models are widely used to assess the toxicity of plant-derived products (Oliveira et al. Citation2012; Santos Aliança et al. Citation2014; Sant’Anna da Silva et al. Citation2016). In the cytotoxicity assays, the BTME showed IC50 values of 981 and 3935 μg/mL against J774 macrophages and human erythrocytes, respectively. These values are higher than the average MIC of the BTME (625 μg/mL). Therefore, it was decided to evaluate the morphological changes induced by the BTME and its effects when in combination with fluconazole.
Fluconazole is recommended as the first choice for treating pathologies caused by C. albicans, mainly due to its high efficiency and good pharmacokinetic properties (Pappas et al. Citation2015). However, the appearance of fluconazole resistance represents an enormous limitation for its use in the treatment of candidiasis (Morschhäuser Citation2016). Consequently, combination therapy has been indicated as a useful alternative to manage Candida infections (Cui et al. Citation2015). Measurement of the combinatory effects of natural products and antimicrobial agents has been taken as a new strategy in the battle against drug resistance (Hemaiswarya et al. Citation2008; Wagner & Ulrich-Merzenich Citation2009; Cui et al. Citation2015) and a range of plant-derived products have been used to increase or restore the action of some antifungal agents such as fluconazole (Khan & Ahmad Citation2012; Avijgan et al. Citation2014; da Silva et al. Citation2014). The BTME was able to increase fluconazole activity against the five resistant strains by addictive and synergetic effects. Compounds from both flavonoid and tannin classes (same classes detected in the BTME) are reported as enhancers of fluconazole activity (Endo et al. Citation2010, da Silva et al. Citation2014).
Depending on environmental conditions, C. albicans can assume three different morphotypes during its development: blastoconidial, pseudohyphal and hyphal forms. Furthermore, C. albicans easily develops into a biofilm on surfaces, which when compared to planktonic cells, displays stronger resistance to a wide variety of antifungal agents (Staniszewska et al. Citation2012; Ma et al. Citation2015). Different from the control cells, morphological observation showed a predominance of single, isolated blastoconidia over the budding cells and no true hyphal development. These results suggest that the BTME substantially affected the morphogenetic transition of C. albicans, which is crucial to its virulence (Staniszewska et al. Citation2012). The ability to produce hyphae (and them switch between yeast and filamentous forms) is of extreme importance to the pathogenesis of candidiasis and is considered the central virulence attribute (Berman & Sudbery Citation2002; Dalle et al. Citation2010; Mayer et al. Citation2013). The yeast-to-hyphae transition is related to tissue damage and invasion and the host inflammatory response, as well as mediating Staphylococcus aureus co-infection (Peters et al. Citation2014; Schlecht et al. Citation2015). The ability of the BTME to inhibit hyphal formation can be considered an attractive characteristic for anti-C. albicans therapy, especially during biofilm formation (Jacobsen et al. Citation2012).
Finally, the BTME induced the significant production of NO from macrophages. NO is a reactive oxygen nitrogen species which is recognized as a marker of macrophage activation, playing an essential role in antimicrobial defence. Specifically, NO suppression is a mechanism used by C. albicans to evade the immune system response, thus macrophage function modulation is a potential aim for antifungal therapy (Collette et al. Citation2014).
In conclusion, this study demonstrated that B. tetraphylla is a great source of antimicrobial compounds able to enhance the action of fluconazole against different C. albicans strains. The methanol extract induced significant morphological changes in treated cells and enhanced NO production in vitro. Therefore, it is presented as a promising source of antifungal agent(s) for the treatment of C. albicans. The identification, molecular and in vivo studies of the active substance(s) will be areas of important future research aimed at finding alternative, new strategies for controlling C. albicans infections using natural products from the Caatinga.
Acknowledgements
The authors are grateful to the following Brazilian agencies: the Conselho Nacional de Desenvolvimento Científico e Tecnológico (CNPq), the Coordenação de Aperfeiçoamento de Pessoal de Nível Superior (CAPES) and the Fundação de Amparo à Ciência e Tecnologia do Estado de Pernambuco (CAPES).
Disclosure statement
The authors confirm that there is no conflict of interest in this article content.
Additional information
Funding
References
- Agra MF, Baracho GS, Silva KN, Basílio IJLD, Coelho VPM. 2007. Medicinal and poisonous diversity of the flora of Cariri Paraibano, Brazil. J Ethnopharmacol. 111:383–395.
- Agra MF, Silva KN, Basílio IJLD Freitas PF, Barbosa-Filho JM. 2008. Survey of medicinal plants used in the region northeast of Brazil. Braz J Pharmacog. 18:472–508.
- Avijgan M, Mahboubi M, Nasab MM, Nia EA, Yousefi H. 2014. Synergistic activity between Echinophora platyloba DC ethanolic extract and azole drugs against clinical isolates of Candida albicans from women suffering chronic recurrent vaginitis. J Mycol Med. 24:112–116.
- Berman J, Sudbery PE. 2002. Candida albicans: a molecular revolution built on lessons from budding yeast. Nat Rev Genet. 3:918–932.
- Blanco MT, Sacristán B, Lucio L, Blanco J, Pérez-Giraldo C, Gómez-García AC. 2009. Cell surface hydrophobicity as an indicator of other virulence factors in Candida albicans. Rev Iberoam Micol. 27:195–199.
- Cassone A. 2014. Vulvovaginal Candida albicans infections: pathogenesis, immunity and vaccine prospects. BJOG. 122:785–794.
- Clinical and Laboratory Standards Institute (CLSI). 2011. Performance standards for antimicrobial susceptibility testing (21st edn) Wayne: M100-S21. Clin Lab Std Inst. 30.
- Collette JR, Zhou H, Lorenz MC. 2014. Candida albicans suppresses nitric oxide generation from macrophages via a secreted molecule. PLoS One. 9:e96203.
- Cui J, Ren B, Tong Y, Dai H, Zhang L. 2015. Synergistic combinations of antifungals and anti-virulence agents to fight against Candida albicans. Virulence. 6:362–371.
- da Silva CR, de Andrade Neto JB, de Sousa Campos R, Figueiredo NS, Sampaio LS, Magalhães HI, Cavalcanti BC, Gaspar DM, de Andrade GM, Lima IS, et al. 2014. Synergistic effect of the flavonoid catechin, quercetin, or epigallocatechin gallate with fluconazole induces apoptosis in Candida tropicalis resistant to fluconazole. Antimicrob Agents Chemother. 58:1468–1478.
- Dalle F, Wächtler B, L'Ollivier C, Holland G, Bannert N, Wilson D, Labruère C, Bonnin A, Hube B. 2010. Cellular interactions of Candida albicans with human oral epithelial cells and enterocytes. Cell Microbiol. 12:248–271.
- Endo EH, Cortez DAG, Ueda-Nakamura T, Nakamura CV, Dias Filho BP. 2010. Potent antifungal activity of extracts and pure compound isolated from pomegranate peels and synergism with fluconazole against Candida albicans. Res Microbiol. 161:534–540.
- Fadda ME, Podda GS, Pisano MB, Deplano M, Cosentino S. 2015. Prevalence of Candida species in different hospital wards and their susceptibility to antifungal agents: results of a three-year survey. J Prev Med Hyg. 49:69–74.
- Gow NA, Hube B. 2012. Importance of the Candida albicans cell wall during commensalism and infection. Curr Opin Microbiol. 15:406–412.
- Gulati M, Nobile CJ. 2016. Candida albicans biofilms: development, regulation and molecular mechanisms. Microbes Infect. 16:1–30.
- Hemaiswarya S, Kruthiventi AK, Doble M. 2008. Synergism between natural products and antibiotics against infectious diseases. Phytomedicine. 15:639–652.
- Jacobsen ID, Wilson D, Wächtler B, Brunke S, Naglik JR, Hube B. 2012. Candida albicans dimorphism as a therapeutic target. Expert Rev Anti Infect Ther. 1:85–93.
- Khan MAS, Ahmad I. 2012. Antibiofilm activity of certain phytocompounds and their synergy with fluconazole against Candida albicans biofilms. J Antimicrob Chemother. 67:618–621.
- Krumperman PH. 1983. Multiple antibiotic resistance indexing of Escherichia coli to identify high-risk sources of fecal contamination of foods. Appl Environ Microbiol. 46:165–170.
- Ma C, Du F, Yan L, He G, He J, Wang C, Rao G, Jiang Y, Xu G. 2015. Potent activities of roemerine against Candida albicans and the underlying mechanisms. Molecules. 20:17913–17928.
- Marchaim D, Lemanek L, Bheemreddy S, Kaye KS, Sobel JD. 2012. Fluconazole-resistant Candida albicans vulvovaginitis. Obstet Gynecol. 120:1407–1414.
- Mayer FL, Wilson D, Hube B. 2013. Candida albicans pathogenicity mechanisms. Virulence. 4:119–128.
- Morschhäuser J. 2016. The development of fluconazole resistance in Candida albicans - an example of microevolution of a fungal pathogen. J Microbiol. 54:192–201.
- Oliveira YLC, Silva LCN, Silva AG, Macedo AJ, Araújo JM, Correia MTS, Silva MV. 2012. Antimicrobial activity and phytochemical screening of Buchenavia tetraphylla (Aubl.) R. A. Howard (Combretaceae: Combretoideae). Sci World J. 2012:1–6.
- Pappas PG, Kauffman CA, Andes DR, Clancy CJ, Marr KA, Ostrosky-Zeichner L, Zaoutis TE. 2015. Clinical practice guideline for the management of candidiasis: 2016 update by the Infectious Diseases Society of America. Clin Infect Dis. 62:e1–50.
- Peters BM, Palmer GE, Nash AK, Lilly EA, Fidel PL, Noverr MC. 2014. Fungal morphogenetic pathways are required for the hallmark inflammatory response during Candida albicans vaginitis. Infect Immun. 82:532–543.
- Pfaller MA, Diekema DJ. 2010. Epidemiology of invasive mycoses in North America. Crit Rev Microbiol. 36:1–53.
- Sant’Anna da Silva AP, Nascimento da Silva LCN, Martins da Fonseca CS, de Araújo JM, Correia MT, Cavalcanti MS, Lima VL. 2016. Antimicrobial activity and phytochemical analysis of organic extracts from Cleome spinosa Jaqc. Front Microbiol. 7:963.
- Santos Aliança AS, Anjos KF, Vasconcelos Reis TN, Higino TM, Brelaz-de-Castro MC, Bianco ÉM, Figueiredo RC. 2014. The in vitro biological activity of the Brazilian brown seaweed Dictyota mertensii against Leishmania amazonensis. Molecules. 19:14052–14065.
- Sardi JCO, Scorzoni L, Bernardi T, Fusco-Almeida AM, Giannini MM. 2013. Candida species: current epidemiology, pathogenicity, biofilm formation, natural antifungal products and new therapeutic options. J Med Microbiol. 62:10–24.
- Schlecht LM, Peters BM, Krom BP, Freiberg JA, Hänsch GM, Filler SG, Shirtliff ME. 2015. Systemic Staphylococcus aureus infection mediated by Candida albicans hyphal invasion of mucosal tissue. Microbiology (Reading, Engl.). 161:168–181.
- Silva LCN, Sandes JM, Paiva MM, Araújo JM, Figueiredo RCBQD, da Silva MV, Correia MTDS. 2013. Anti-Staphylococcus aureus action of three Caatinga fruits evaluated by electron microscopy. Nat Prod Res. 27:1492–1496.
- Silva LN, Trentin DDS, Zimmer KR, Treter J, Brandelli CLC, Frasson AP, Macedo AJ. 2014. Anti-infective effects of Brazilian Caatinga plants against pathogenic bacterial biofilm formation. Pharm Biol. 53:464–468.
- Silva-Dias A, Miranda IM, Branco J, Monteiro-Soares M, Pina-Vaz C, Rodrigues AG. 2015. Adhesion, biofilm formation, cell surface hydrophobicity, and antifungal planktonic susceptibility: relationship among Candida spp. Front Microbiol. 6:1–8.
- Sobel JD, Zervos M, Reed BD, Hooton T, Soper D, Nyirjesy P, Panzer H. 2003. Fluconazole susceptibility of vaginal isolates obtained from women with complicated Candida vaginitis: clinical implications. Antimicrob Agents Chemother. 47:34–38.
- Staniszewska M, Bondaryk M, Siennicka K, Kurzatkowski W. 2012. Ultrastructure of Candida albicans pleomorphic forms: phase-contrast microscopy, scanning and transmission electron microscopy. Pol J Microbiol. 61:129–135.
- Teodoro GR, Brighenti FL, Delbem ACB, Delbem ÁCB, Khouri S, Gontijo AVL, Koga-Ito CY. 2015. Antifungal activity of extracts and isolated compounds from Buchenavia tomentosa on Candida albicans and non-albicans. Future Microbiol. 10:917–927.
- Vuuren SV, Viljoen A. 2011. Plant-based antimicrobial studies methods and approaches to study the interaction between natural products. Planta Med. 77:1168–1182.
- Wagner H, Ulrich-Merzenich G. 2009. Synergy research: approaching a new generation of phytopharmaceuticals. Phytomedicine. 16:97–110.
- Weaver PL. 1991. Buchenavia tetraphylla (Vahl.) Eichler: Granadillo. New Orleans (LA): Department of Agriculture, Southern Forest Experiment Station.