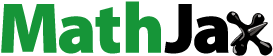
Abstract
Context: Extensive research on Rhus (Anacardiaceae) shows their antioxidant potential, which warrants further evaluation of its other species.
Objective: To perform a comparative antioxidant assay on extracts of R. retinorrhoea and R. tripartita, including sakuranetin quantification by a validated HPTLC method.
Materials and methods: In vitro antioxidant assay was performed on chloroform and ethanol extracts of R. retinorrhoea Steud. ex Oliv. (RRCE and RREE) and R. tripartita (Ucria) Grande (RTCE and RTEE) by DPPH radical scavenging (at 31.25, 62.5, 125, 250 and 500 μg/mL concentrations) and β-carotene-linoleic acid bleaching methods at 500 μg/mL concentration. Densitometric HPTLC method was developed and validated using toluene: ethyl acetate: methanol (8:2:0.2; v/v/v) as mobile phase, executed on glass-backed silica gel F254 plate and scanned at 292 nm.
Results: Antioxidant activity of Rhus extracts tested by the two methods (DPPH/BCB) was found in order of RTEE > RREE > RTCE > RRCE with IC50 118.67/256.26, 315.75/82.35, 827.92/380.0 and 443.69/292.75, respectively. Scanning of the HPTLC plate provided an intense peak of sakuranetin at Rf = 0.59. The estimated sakuranetin content in the dry weight of the extracts was highest in RREE (27.95 μg/mg) followed by RRCE (25.22 μg/mg), RTEE (0.487 μg/mg) and RTCE (0.0 μg/mg). Presence of sakuranetin in RREE, RRCE and RTEE supported the highest antioxidant property of the two Rhus species. Nonetheless, low sakuratenin in R. tripartita indicated the presence of other bioactive constituents responsible for synergistic antioxidant activity.
Conclusion: The developed HPTLC method therefore guarantees its application in quality control of commercialized herbal drugs and formulations containing sakuranetin.
Introduction
Exploring novel and effective naturally derived antioxidants of nutritional values for human health has been a major goal for herbalists. The genus, Rhus (Anacardiaceae) commonly known as ‘sumac’ is comprised of 250 flowering plant species distributed in the temperate and tropical regions, worldwide (USDA Citation2007). Traditionally, several species of Rhus have been used for their medicinal purposes by indigenous cultures (Van Wyk & Wink Citation2004). Of these, Rhus glabra L. (smooth sumac) (Anacardiaceae) has been used in the treatment of bacterial infection like syphilis, gonorrhea, dysentery, and gangrene by North American people (Erichsen-Brown Citation1989). Other species, Rhus coriaria L. (tanner’s sumac) is commonly used as spice and wound healing herb in the Mediterranean and Middle East regions (Sezik et al. Citation1991). Moreover, maximum research dedicated to Rhus extracts has shown their antioxidant potential that warrants for further evaluations of its other species and commercialization.
The ethanol extract of Rhus verniciflua Stokes was reported to exhibit strong antioxidant properties against reactive oxygen species wherein its aqueous fraction protected against thymocyte apoptosis, attributed to antioxidant flavonoid contents (butein, quercitin, fustin and sulfuretin) (Lee et al. Citation2001). In addition, fustin and sulfuretin were found in Rhus copallina, Rhus typhina (Young Citation1976) and R. glabra (Keppler Citation1957; Yasue & Kato Citation1957) which had suggested their potential antioxidant properties. The ethanol extract of R. verniciflua wood was found superior to BHA and α-tocopherol in its antioxidative action (Park et al. Citation2002). The alcoholic extract of fruits and leaves of R. coriaria is used in stabilization of peanut oil due to its high antioxidant properties (Ozcan Citation2003a, Citation2003b). Rhus tripartita is mainly found in North Africa (Tackholm Citation1974) and widely used in food and traditional medicine (Wu et al. Citation2013). It has been extensively used in the treatment of several ailments including diarrhea, inflammatory diseases, diabetes, sexual disease, fever, pain and various cancers (Lev & Amar Citation2002; Giancarlo et al. Citation2006; Kossah et al. Citation2010; Lee et al. Citation2010). Furthermore, several flavonoid phytoconstituents with mild antimalarial activity have been also reported in Rhus retinorrhoea (Ahmed et al. Citation2001).
Flavonoids are the phenolic compounds of plant origin, and exhibit antioxidant, antimicrobial, antiallergenic, antiviral, anti-inflammatory and photoreceptor activities. The antioxidant property of flavonoid is attributed to its ability to reduce the formation of free radicals and promotion of free radical scavenging (Pier-Giorgio Citation2000). Of these, sakuranetin [(+/−) 7-O-methylnaringenin] is an important flavanone () (Jung et al. Citation2005) that has been identified in several other genera like, Oryza sativa L. (Grasses) (Rakwal et al. Citation1996), Boesenbergia pandurata (Roxb.) Schltr. (Zingiberaceae) (Tuchinda et al. Citation2002), Eriodictyon californicum (Hook. & Arn.) Torr. (Hydrophylloideae) (Liu et al. Citation1992) and different Piper species (Orjala et al. Citation1994; Moreira et al. Citation2000). Sakuranetin has been found to possess antioxidant (Soarse et al. Citation2005), chemopreventive (Charles et al. Citation2014), antinoceptive, and anti-inflammatory properties (Zhang et al. Citation2006; Cruz et al. Citation2016).
Based on this background information indicating promising antioxidative potential of Rhus extracts with few applied examples, we argue that this genus may offer a promising natural source of commercial antioxidants. Therefore, we performed in this study a comparative antioxidant assay of chloroform and ethanol extracts of aerial parts of Rhus retinorrhoea () and Rhus tripartita () along with the estimation of sakuranetin by a validated HPTLC method.
Materials and methods
Plant material
The aerial parts of R. retinorrhoea (Voucher number-15371) were collected in March 2009 and R. tripartita (Voucher number-15807) were collected in March, 2012 from northern part of Saudi Arabia. These samples were authenticated by Dr. Mohammed Yusuf (Field taxonomist, Pharmacognosy Department) and specimens were deposited at the college herbarium.
Plant material extraction by using ultrasonic method
The aerial parts of R. retinorrhoea (RR) and R. tripartita (RT) were dried in air, pulverized and passed through a 0.75 mm sieve. The extraction process was carried out in an ultrasonic cleaner Transsonic-460/H (ELMA, Germany). The powdered material of RR (5.0 g) and RT (5.0 g) were extracted by ultrasonication (frequency 20 kHz, power 100 W) using ethanol (95%) and chloroform, separately. The chloroform and ethanol extracts (CE and EE, respectively) were centrifuged at 5000 rpm for 20 min, filtered through Whatman filter paper No. 1. Therefore, the obtained extracts (CE and EE) of the two Rhus species (RT and RR) were concentrated and dried under reduced pressure using rotary evaporator (R-210, BUCHI). The estimated yields (w/w) of RRCE, RREE, RTCE and RTEE were 4.52, 8.75, 3.86 and 5.62%, respectively.
Apparatus and reagents
Sakuranetin (Biomarker; purity: ≥95%), rutin (antioxidant standard; purity: ≥94%), Tween_40, 2,2-diphenyl-1-picrylhydrazyl (DPPH), β-carotene, linoleic acid were purchased from Sigma Aldrich (USA). In addition, the AR grade toluene, ethyl acetate, chloroform, ethanol were procured from BDH (UK) and HPLC grade methanol were procured form Merck (Germany) where, glass-backed silica gel 60F254 plate was also procured for the HPTLC analysis. Furthermore, CAMAG Automatic TLC Sampler-4 (Switzerland) was used to apply the sakuranetine and the Rhus extracts (RRCE, RREE, RTCE and RTEE), band wise to the chromatographic plates and development of the plate was carried out in automatic development chamber (ADC2) (Switzerland). The developed HPTLC plates were then scanned and documented by CATS 4 (CAMAG) and TLC Reprostar 3 (CAMAG), respectively.
Antioxidant assay
1,1-Diphenyl-2-picrylhydrazyl (DPPH) radical scavenging assay
Antioxidant activities of RRCE, RREE, RTCE and RTEE were evaluated quantitatively by free-radical scavenging ability against DPPH as per the previously described method (Lee et al. Citation2013) with minor alteration to suite 96-well microtitre plate format. To sum up, 100 μL of different concentrations (31.25, 62.5, 125, 250 and 500 μg/mL) of each extract was mixed with 40 μL of DPPH (0.2 mM in methanol) in wells of a 96-well microtitre plate. Moreover, appropriate control was prepared using the solvent only in addition to the same amount of DPPH reagent to get rid of any inherent solvent effect. Rutin was used as standard. After 30 min incubation in dark at 25 °C, the decrease in absorbance (Abs) was measured at λ= 517 nm using microtitre plate reader. The test was carried out in triplicate. The radical scavenging activity was calculated from the equation:
β-Carotene-linoleic acid bleaching assay
The antioxidant activities of RRCE, RREE, RTCE and RTEE were evaluated by using the β-carotene bleaching method (Miller Citation1971) with minor modifications for working with 96 well plate. Briefly, 0.25 mg β-carotene was dissolved in 0.5 ml of chloroform and added to flasks containing 12.5 μg of linoleic acid and 100 mg of Tween-40. The chloroform was evaporated at 43 °C using speed vacuum concentrator (Savant, Thermo Electron Co.). The resultant mixture was immediately diluted to 25 mL with distilled water and shaken vigorously for 2–3 min to form an emulsion. A 150 μL aliquot of the emulsion was added to the wells of a 96-well microtitre plate containing 50 μL of each plant extract or rutin at 500 μg/mL. In addition, a control containing solvent instead of extract was also prepared. The plate was incubated at 50 °C for 2 h. Absorbance was taken at 470 nm at 30 min intervals using microplate spectrophotometer (BioRad Laboratories Inc., Hercules, CA). The test was carried out in triplicate. Then, antioxidant activity was expressed as % inhibition of lipid peroxidation using the formula:
where Abssam120 and Abscont120 are the absorbance of the sample and control, respectively, at time 120 min, and Abscon0 is the absorbance of the control at time 0 min.
HPTLC instrumentation and conditions
The HPTLC analysis of sakuranetin in RRCE, RREE, RTCE and RTEE was carried out on NP-HPTLC plates (20 × 10 cm) where the band size of each track was 6 mm wide and 9.4 mm apart. The marker and samples were applied on HPTLC plate (160 nL/sec), and developed in pre-saturated twin-trough glass chamber (20 × 10 cm) at 25 ± 2 °C under 60 ± 5% humidity. The developed HPTLC plate was dried and quantitatively analyzed at 292 nm wavelength in absorbance mode.
Preparation of standard stock solutions
The fresh stock solution of sakuranetin (1 mg/mL) was prepared in methanol and further diluted to furnish different concentrations (10-180 μg/mL) in the same solvent. All samples were applied (10 μL, each) through microliter syringe attached with the applicator on the HPTLC plate to provide the linearity range of 100–1800 ng/band.
Validation of method
Validation of the proposed HPTLC method was performed according to the ICH guidelines, for the determination of linearity range, limit of detection (LOD), limit of quantification (LOQ), precision, recovery as accuracy and robustness (ICH guidelines, Citation2005).
Statistical analysis
The statistical analysis was carried out by one-way analysis of variance (ANOVA) followed by Dunnet’s test for the estimation of total variation in a set of data. Results were expressed as mean ± SD. p < 0.01, which was considered significant.
Results
Antioxidant activity (in vitro)
Free radical scavenging activities (DPPH) of RRCE, RREE, RTCE and RTEE were tested for the first time and the results are represented in . The IC50 values for RRCE, RREE, RTCE and RTEE in DPPH assay were found to be 443.69, 315.75, 827.92 and 118.67 μg/mL, respectively, whereas in the case of β-carotene-linoleic acid bleaching assay they were found to be 292.75, 82.35, 380.00 and 256.26 μg/mL, respectively. At the concentration of 500 μg/mL, the maximal antioxidant activity was found in the ethanol extracts, RTEE (90.6%) and RREE (70.5%). However, the chloroform extracts (RTCE and RRCE) of the two plants had no significant difference. The radical scavenging activity of the RTEE was comparable to that of the positive control rutin (93.3%). In accordance to the findings of β-carotene-linoleic acid bleaching assay, RTEE and RREE showed higher antioxidant potential than RTCE and RRCE (). Taken together, the ethanol extract of R. tripartita had higher antioxidant potential than the other extracts of R. tripartita and R. retinorrhoea.
Figure 3. DPPH radical scavenging activity of different concentrations (31.25–500 μg/ml) of RRCE, RREE, RTCE and RTEE. Values are means of three experiments.

Figure 4. Antioxidant activity of RRCE, RREE, RTCE and RTEE in comparison to the standard antioxidant (rutin) assayed by the β-carotene bleaching method showing percentage of inhibition of lipid peroxidation by different concentrations (31.25–500 μg/ml) of the extracts. Values are means of three experiments.

HPTLC method development and validation
The mobile phase used in HPTLC analysis was selected by trying several compositions of different solvents. Of these, combination of toluene, ethyl acetate and methanol in the ratio of 8:2:0.2 (v/v/v) under chamber saturation condition was found to be the best mobile phase for the development and quantitative analysis of sakuranetin. The developed method was able to provide an intense, compact and sharp peak of sakuranetin at Rf 0.59 ± 0.003 (). This method clearly separated the biomarker sakuranetin and different constituents of RRCE, RREE, RTCE and RTEE (). The optimized saturation time and mobile phase volume for saturation were found to be 20 min and 20 ml, respectively. The identities of the bands were confirmed by overlaying their spectra along with sakuranetin (). The developed methods was therefore, found to be selective with high resolution baseline. The regression equation and correlation co-efficient (r2) for sakuranetin were found to be Y = 15.382x + 5325.43 and 0.9979 ± 0.0010 in the linearity range 100–1800 ng/spot. The limit of detection (LOD) and limit of quantification (LOQ) for sakuranetin were found to be 27.56 and 83.53 ng/band, respectively (). The recoveries as accuracy study for the proposed method was recorded (). The recovery (%), RSD (%) and SEM for sakuranetin were 98.65–99.58%, 0.229–0.298% and 0.186–0.550, respectively. The intra-day and inter-day precision for the proposed method was recorded (). The %RSD for intra-day and inter-day precisions (n = 6) were found to be 0.185–0.293% and 0.173–0.279%, respectively, which showed the good precision of the proposed method. The robustness data of sakuranetin were reported in the . The low SD, % RSD and SEM values indicated that the method was robust.
Figure 6. Quantification of sakuranetin in the extracts of two Rhus spp. by HPTLC. (A) Pictogram of developed HPTLC plate at 254 nm [mobile phase: Toluene: EA: Methanol, (8:2:0.2, v/v/v)]; (B) 3-D display of all tracks at 292 nm.
![Figure 6. Quantification of sakuranetin in the extracts of two Rhus spp. by HPTLC. (A) Pictogram of developed HPTLC plate at 254 nm [mobile phase: Toluene: EA: Methanol, (8:2:0.2, v/v/v)]; (B) 3-D display of all tracks at 292 nm.](/cms/asset/01c3f9e7-ac20-429e-88f7-72cdf26d9d9a/iphb_a_1304428_f0006_c.jpg)
Table 1. Rf, Linear regression data for the calibration curve of sakuranetin (n = 6).
Table 2. Recovery as accuracy studies of the proposed HPTLC method (n = 6).
Table 3. Precision of the proposed HPTLC method (n = 6).
Table 4. Robustness of the proposed HPTLC method (n = 6).
HPTLC analysis of sakuranetin
The proposed HPTLC method was used for quantitative analysis of sakuranetin in the Rhus extracts RRCE, RREE, RTEE and RTCE (). By applying this method, the estimated quantity of sakuranetin was 27.95 μg/mg for RREE (), 25.22 μg/mg for RRCE () and 0.487 μg/mg for RTEE () of the dried weight of extracts. However, sakuranetin was not detected in RTCE (). This maiden report thus, demonstrated the development of an economical, precise, accurate and simple HPTLC method for quantitative analysis of sakuranetin in different extracts of R. retinorrhoea and R. tripartita.
Figure 8. Chromatogram of sakuranetin estimation in the extracts of Rhus spp. at 292 nm [mobile phase: Toluene: EA: Methanol, (8:2:0.2, v/v/v)]. (A) R. retinorhoea chloroform extract (RRCE; spot 4, Rf = 0.59); (B) R. retinorhoea ethanol extract (RREE; spot 4, Rf = 0.59); (C) R. tripartita ethanol extract (RTEE; spot 4, Rf = 0.59); (D) R. tripartita chloroform extract (RTCE; Sakuranetin is absent).
![Figure 8. Chromatogram of sakuranetin estimation in the extracts of Rhus spp. at 292 nm [mobile phase: Toluene: EA: Methanol, (8:2:0.2, v/v/v)]. (A) R. retinorhoea chloroform extract (RRCE; spot 4, Rf = 0.59); (B) R. retinorhoea ethanol extract (RREE; spot 4, Rf = 0.59); (C) R. tripartita ethanol extract (RTEE; spot 4, Rf = 0.59); (D) R. tripartita chloroform extract (RTCE; Sakuranetin is absent).](/cms/asset/6d664ea9-4721-4323-9e35-d758eda619d9/iphb_a_1304428_f0008_c.jpg)
Discussion
It is commonly recommended to perform different assay methods for evaluation of antioxidant activity (Alam et al. Citation2013). In DPPH assay, an antioxidant molecule provides an electron to DPPH which results in decay of its purple color. The measured decrease in the absorbance therefore, correlates with the free radical scavenging potential of the test sample. This represents one of the most common methods used to measure an extract’s ability to scavenge free radical. In addition, this is a favoured method because of its simplicity and compatibility with hydrophilic, lipophilic, pH, temperature and light sensitive nature of antioxidant samples (Kedare & Singh Citation2011). On the other hand, in β-carotene bleaching method, the linoleic acid radical formed by the loss of a hydrogen atom from one of its diallylic methylene groups attacks unsaturated β-carotene molecules. As a result, β-carotene is oxidized and subsequently the system loses its chromophore and characteristic orange colour. In our in vitro assays, the more polar ethanol extract (RTEE and RREE) contains more phenolic compounds, therefore exhibited the highest antioxidant activity in comparison to the chloroform fraction of both plants. The available literature also revealed the presence of several phytoconstituents, like flavonoids, biflavonoids and isobiflavonoids in R. tripartita (Mahjoub et al. Citation2004, Citation2005; Alimi et al. Citation2013) which supports our findings of high-antioxidant property of RTEE. It was found that the sakuranetin content was more in RREE than RTEE. Sakuranetin has been proved to be a good antioxidant. However, its presence in high quantity in RREE and low quantity in RTEE is not only responsible for good antioxidant activity of RREE and RTEE, which reflected that there were several other flavonoids and phenolic compounds present in RREE and RTEE. The cumulative affect along with sakuranetin imparts excellent antioxidant property to RRTE and RREE. Our findings also approved the previous antioxidant finding of R. tripartita (Abd El-Salam Citation2015). The antioxidant activity of phenolic phytoconstituents is attributed to their redox properties, which make them to act as reducing agents and hydrogen donors.
According to published reports, sakuranetin has been analyzed in different plants by several techniques, like nuclear magnetic resonance (NMR) (Jerz et al. Citation2005); IR & UV (Zhang et al. Citation2006), Mass spectrometry (Danelutte et al. Citation2003); HPLC & LC–MS (Jung et al. Citation2005), and EI-MS (Ogawa et al. Citation2007). However, there is no validated HPTLC method reported so far, for the quality control of sakranetin in herbal formulations or drugs. Recently, HPTLC has been extensively used in quality control of herbal drugs because of its various unique features, like inexpensive, high sample throughput, and requirement of very less cleaning solvent (Alam et al. Citation2014). In individual capacity, HPTLC is a reliable analytical tool for identification of herbs and their constituents, purity and stability testing of their preparations and analysis of uniformity, including those of animal extracts, drugs and excipients. Therefore, it has been widely used in the standardization and quality control of formulated products viz. pharmaceuticals, cosmetics, herbal and nutritional supplements (Alajmi et al. Citation2013). The present validated HPTLC method developed for the estimation of sakuranetin in two Rhus species would be beneficial in helping and facilitating the quality control of herbal drugs.
Conclusion
The finding of high degree of antioxidant property of alcoholic extracts of R. retinorrhoea and R. tripartita warrants further evaluation of the two species in the various chronic diseases caused by free radical-induced oxidative damages. Our developed HPTLC method may be further applied for the quality control of herbal drugs or formulations containing sakuranetin, including the study of degradation kinetics.
Disclosure statement
The authors declare that they do not have any conflict of interest.
Additional information
Funding
References
- Abd El-Salam IM. 2015. Phytoconstituents and the study of antioxidant, antimalarial and antimicrobial activities of Rhus tripartite growing in Egypt. J Pharmacogn Phytochem. 4:276–281.
- Ahmed MS, Galal AM, Ross SA, Ferreira D, El Sohly MA, Ibrahim AS, Mossa JS, El-Feraly FS. 2001. A weakly antimalarial biflavanone from Rhus retinorrhoea. Phytochemistry. 58:599–602.
- Alajmi MF, Alam P, Shakeel F. 2013. Quantification of bioactive marker β-amyrin by validated high-performance thin-layer chromatographic-densitometric method in different species of Maytenus grown in Saudi Arabia. J Planar Chromatogr. 26:475–479.
- Alam MN, Bristi NJ, Rafiquzzaman M. 2013. Review on in vivo and in vitro methods evaluation of antioxidant activity. Saudi Pharm J. 21:143–152.
- Alam P, Siddiqui NA, Al-Rehaily AJ, Alajmi MF, Basudan OA, Khan TH. 2014. Stability-indicating densitometric high-performance thin-layer chromatographic method for the quantitative analysis of biomarker naringin in the leaves and stems of Rumex vesicarius L. J Planar Chromatogr. 27:204–209.
- Alimi H, Mbarki S, Barka ZB, Feriani A, Bouoni Z, Hfaeidh N, Sakly M, Tebourbi O, Rhouma KB. 2013. Phytochemical, antioxidant and protective effect of Rhus tripartitum root bark extract against ethanol-induced ulcer in rats. Gen Physiol Biophys. 32:115–127.
- Charles C, Nachtergael A, Ouedraogo M, Belayew A, Duez P. 2014. Effects of chemopreventive natural products on non-homologous end-joining DNA double-strand break repair. Mutat Res Genet Toxicol Environ Mutagen. 768:33–41.
- Cruz MP, Andrade CM, Silva KO, de Souza EP, Yatsuda R, Marques LM, David JP, David JM, Napimoga MH, Clemente-Napimoga JT. 2016. Antinoceptive and anti-inflammatory activities of the ethanol extract, fractions and flavones isolated from Mimosa tenuiflora (Willd.) Poir (Leguminosae). PLoS One. 11:1–29.
- Danelutte AP, Lago JH, Young MC, Kato MJ. 2003. Antifungal flavanones and prenylated hydroquinones from Piper crassinervium Kunth. Phytochemistry 64:555–559.
- Erichsen-Brown C. 1989. Medicinal and other uses of North American plants: a historical survey with special reference to the Eastern Indian Tribes. Mineola, New York, USA: Dover Publications.
- Giancarlo S, Rosa ML, Nadjafi F, Francesco M. 2006. Hypoglycaemic activity of two spices extracts: Rhus coriaria L. and Bunium persicum Boiss. Nat Prod Res. 20:882–886.
- International Conference on Harmonization (ICH) of Technical Requirements for Registration of Pharmaceuticals for Human use, Harmonised Triplicate Guideline on Validation of Analytical Procedures: Text and Methodology Q2 (R1). 2005. Complementary Guideline on Methodology incorporated in November 2005 by the ICH Steering Committee, IFPMA, Geneva.
- Jerz G, Waibel R, Achenbach H. 2005. Cyclohexanoid protoflavanones from the stem-bark and roots of Ongokea gore. Phytochemistry. 66:1698–1706.
- Jung YH, Lee JH, Agrawal GK, Rakwal R, Kim J-A, Shim J-K, Lee S-K, Jeon J-S, Koh H-J, Lee Y-H, et al. 2005. The rice (Oryza sativa) blast lesion mimic mutant, blm, may confer resistance to blast pathogens by triggering multiple defense-associated signaling pathways. Plant Physiol Biochem. 43:397–406.
- Kedare SB, Singh RP. 2011. Genesis and development of DPPH method of antioxidant assay. J Food Sci Technol. 48:412–422.
- Keppler HH. 1957. The isolation and constitution of molisacacidin, a new leucoanthocyanidin from the heartwood of Acacia mollisima. J Chem Soc. 2721–2724.
- Kossah R, Nsabimana C, Zhang H, Chen W. 2010. Optimization of extraction of polyphenols from Syrian Sumac (Rhus coriaria L.) and Chinese Sumac (Rhua typhina L.) fruits. Res J Phytochem. 4:146–153.
- Lee JC, Kim J, Lim KT, Yang MS, Jang YS. 2001. Ethanol eluted extract of Rhus verniciflua Stokes showed both antioxidant and cytotoxic effects on mouse thymocytes depending on the dose and time of the treatment. J Biochem Mol Biol. 34:250–258.
- Lee SK, Jung HS, Eo WK, Lee SY, Kim SH, Shim BS. 2010. Rhus verniciflua Stokes extract as a potential option for treatment of metastatic renal cell carcinoma: report of two cases. Ann Oncol. 21:1383–1385.
- Lee YJ, Kim DB, Lee J, Cho JH, Kim B, Choi HS, Lee JS, Cho J-H, Kim BK, Choi H-S, et al. 2013. Antioxidant activity and anti-adipogenic effects of wild herbs mainly cultivated in Korea. Molecules. 18:12937–12950.
- Lev E, Amar Z. 2002. Ethnopharmacological survey of traditional drugs sold in the Kingdom of Jordan. J Ethnopharmacol. 82:131–145.
- Liu YL, Ho DK, Cassady JM, Cook VM, Baird WM. 1992. Isolation of potential cancer chemopreventive agents from Eriodictyon californicum. J Nat Prod. 55:357–363.
- Mahjoub MA, Ammar S, Mighri Z. 2004. Isolation and structure studies of a few natural compounds from the Tunisian plant Rhus tripartitum. J Soc Alger Chim. 14:293–297.
- Mahjoub MA, Ammar S, Mighri Z. 2005. A new biflavonoid and an isobiflavonoid from Rhus tripartitum. Nat Prod Res. 19:723–729.
- Miller HE. 1971. A simplified method for the evaluation of antioxidants. J Am Oil Chem Soc. 48:91–105.
- Moreira DD, Guimaraes EF, Kaplan MA. 2000. A C-glucosylflavone from leaves of Piper ihotzkyanum. Phytochemistry. 55:783–786.
- Ogawa Y, Oku H, Iwaoka E, Iinuma M, Ishiguro K. 2007. Allergy-preventive flavonoids from Xanthorrhoea hastilis. Chem Pharm Bull (Tokyo). 55:675–667.
- Orjala J, Wright AD, Behrends H, Folkers G, Sticher O, Rüegger H, Rali T. 1994. Cytotoxic and antibacterial dihydrochalcones from Piper aduncum. J Nat Prod. 57:18–26.
- Ozcan M. 2003a. Effect of sumach (Rhus coriaria L.) extracts on the oxidative stability of peanut oil. J Med Food. 6:63–66.
- Ozcan M. 2003b. Antioxidant activities of rosemary, sage, and sumac extracts and their combinations on stability of natural peanut oil. J Med Food. 6:267–270.
- Park YS, Kim YS, Shin DH. 2002. Antioxidative effects of ethanol extracts from Rhus vernicifera Stoke on yukwa (oil popped rice snack) base during storage. J Food Sci. 67:2474–2479.
- Pier-Giorgio P. 2000. Flavonoids as antioxidants. J Nat Prod. 63:1035–1042.
- Rakwal R, Hasegawa M, Kodama O. 1996. A methyltransferase for synthesis of the flavanone phytoalexin sakuranetin in rice leaves. Biochem Biophys Res Commun. 222:732–735.
- Sezik E, Tabata M, Yesilada E. 1991. Traditional medicine in Turkey. I. Folk medicine in northeast Anatolia. J Ethnopharmacol. 35:191–196.
- Soarse DG, Andreazza AC, Salvador M. 2005. Evaluation of compounds with antioxidant activity in Sachhromyces cerevisiae yeast cells. Rev Bras Cienc Farm. 41:95–100.
- Tackholm V. 1974. Students' flora of Egypt. 2nd ed. Egypt: Cairo University Press.
- Tuchinda P, Reutrakul V, Claeson P, Pongprayoon U, Sematong T, Santisuk T, Taylor WC. 2002. Anti-inflammatory cyclohexenyl chalcone derivatives in Boesenbergia pandurata. Phytochemistry. 59:169–173.
- USDA. 2007. Germplasm Resources Information Network. Beltsville, MD, USA: United States Dept. Agric., Agric. Res Serv. Available from: http://www.ars-grin.gov/npgs/aboutgrin.html.
- Van Wyk BE, Wink M. 2004. Medicinal plants of the world. Portland, Oregon, USA: Timber Press, pp. 425.
- Wu T, McCallum JL, Wang S, Liu R, Zhu H, Tsao R. 2013. Evaluation of antioxidant activities and chemical characterization of staghorn sumac fruit (Rhus hirta L.). Food Chem. 138:1333–1340.
- Yasue M, Kato Y. 1957. Components of wood of Rhus trichocarpa. Yakugaku Zasshi. 77:1045–1047.
- Young DA. 1976. Flavonoid chemistry and the phylogenetic relationships of the Julianiaceae. Syst Bot. 1:149–162.
- Zhang X, Hung TM, Phuong PT, Ngoc TM, Min BS, Song KS, Seong YH, Bae K. 2006. Anti-inflammatory activity of flavonoids from Populus davidiana. Arch Pharm Res. 29:1102–1108.