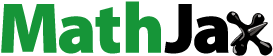
Abstract
Context: Glucosidases are a group of enzymes playing crucial roles in digestion of carbohydrates. Glucosidase inhibitors can reduce carbohydrate digestion rate and have the potential to prevent development of type 2 diabetes. The Labiatae is one of the largest plant families grown globally and many studies that have isolated new pharmaceutical compounds. In folk medicine, some of Labiatae plants such as Zataria multiflora Boiss, Salvia mirzayanii Rech. F. & Esfand, and Otostegia persica Boiss are consumed for the treatment of diabetes.
Objectives: This study investigates the inhibitory effects of different fractions of three mentioned species extracts on α-glucosidase.
Materials and methods: Ethanol extracts of these plants leaves were fractionated using petroleum ether, chloroform, ethyl acetate, and n-butanol solutions. The duration of this study was 12 months. To measure enzyme inhibition, 5 μL of the enzyme, 20 μL of substrate and samples were used and for evaluation mode of inhibition, constant amounts of α-glucosidase were incubated with rising concentrations of substrate (PNPG).
Results: The results revealed that the ethyl acetate fraction of Zataria multiflora (IC50 = 0.35 ± 0.01 mg/mL) and petroleum ether fraction of Salvia mirzayanii (IC50 = 0.4 ± 0.11 mg/mL) were the most potent inhibitors of α-glucosidase in comparison with the other samples and acarbose as the standard (IC50 = 7 ± 0.19 mg/mL). All of the samples exhibited noncompetitive-uncompetitive inhibition.
Discussion and conclusion: It can be inferred from this study that α-glucosidase inhibitory potential of the studied extracts may be a marker of antidiabetic potential of these extracts.
Introduction
Prevalence of type 2 diabetes mellitus (T2DM) has been increasing rapidly over the past decade. Unless appropriate action is taken, it is foretold that there will be at least 350 million people in the world with type 2 diabetes by the year 2030 (World Health Organization Citation2003). Type 2 diabetes mellitus (T2DM) is a multifaceted heterogeneous group of metabolic disarray with hyperglycemia and defective insulin action and/or insulin secretion (Lin & Sun Citation2010). Impairment of insulin actions is known as insulin resistance, the failure which is located at the signaling pathways held after insulin binding to its receptor.Chronic occurrence of the mentioned failure leads to hyperglycemia (Fernández-Mejía Citation2013). Postprandial hyperglycemia is a prominent and early defect in diabetes type 2, which can induce oxidative stress through excessive generation of free radicals that may impair the endogenous antioxidant defense and in turn lead to various secondary complications including cardiovascular risk factors (Johansen et al. Citation2005). Therefore, one of the therapeutic approaches proposed for diabetes is to control the postprandial hyperglycemia by delaying glucose absorption (Akhilesh Citation2012). One of the strategies adopted to treat diabetes mellitus is inhibition of carbohydrate breakdown enzymes such as α-glucosidase (EC 3.2.1.20) in the epithelial mucosa of small intestine performed with association retardation of intestinal glucose absorption and decrease of postprandial blood glucose levels (Shai et al. Citation2010). Nowadays, some α-glucosidase inhibitors, e.g., acarbose and voglibose, are widely used clinically to control patients blood glucose levels. Although they are observed to be effective, they usually cause negative effects such as abdominal distension, flatulence, meteorism, and possibly diarrhea (Verspohl Citation2012). With consideration of the mentioned point, many efforts have been made to specify more effective and unique α-glucosidase inhibitors, and a good number of them have been identified with application of nature sources. On account of their matchless chemical diversity and biological connection, natural materials have been widely recognized as potential chemical resources in drug screening. Therefore, discovery of α-glucosidase inhibitors from natural materials for treatment of diabetes can be regarded as a promising topic (Coman et al. Citation2012). To treat T2DM, more than 1000 plant species have been made use of worldwide (Trojan-Rodrigues et al. Citation2012). Lamiaceae family is one of the largest families of flowering plants with approximately 220 genera and almost 4000 species. Several species of this family such as Zataria multiflora Boiss (Zm), Salvia mirzayanii Rech. F& Esfand (Sm), and Otostegia persica Boiss (Op) (Naghibi et al. Citation2010) are used to treat diabetes in both traditional and modern medicine. Studies conducted in this regard have demonstrated that Zm aqeous extract has a strong inhibitory effect on α-glucosidase (Gholamhoseinian et al. Citation2008) and also significantly decreases postprandial glucose level (Najar et al. Citation2009). This extract increases insulin sensitivity and PPARγ gene expression in high fructose fed insulin resistant rats (Mohammadi et al. Citation2014). The aerial parts of Sm are used in Iranian traditional medicine as anti-stomachache, anti-diabetes, and anti-spasmolytic (Keller Citation1978; Zargari Citation1990; Kamatou et al. Citation2008). Otostegia persica is traditionally used in some regions of Iran as antidiabetic medicinal plant. Thus, its addressed potential has been investigated by some research groups (Lima et al. Citation2006; Ebrahimpoor et al. Citation2009; Tofighi et al. Citation2009; Hajzadeh et al. Citation2011; Manzari-Tavakoli et al. Citation2013; Mohammadi et al. Citation2014; Sepehri et al. Citation2014). As the review literature reveals no documents about the effects of different fractions of Zm, Op, and Sm extracts on inhibition of α-glucosidase have been presented. Hence, the very aim of this study was to investigate this property.
Materials and methods
Chemicals
PNPG (4-nitrophenyl α-d-glucopyranoside) and α-glucosidase from Saccharomyces cerevisiae were purchased from Sigma Aldrich, USA. Other chemicals were purchased from Merck.
Specimen collection and extracts fractionation
In this study, 1 kg of aerial parts of three plants was used. Sm (MPRCM 94-84) and OP (MPRCM 94-86) were collected from Genu Mountains in the northeast of Bandar Abbas on 20 and 15 June, 2012, respectively. Zm (MPRCM 94-83) was obtained from Shiraz medicinal plants store on 25 June 2012 and identified by Dr. Moein. Voucher specimens have been deposited in the herbarium of Medicinal Plants Processing Research Center, Shiraz University of Medical Sciences, Iran. The collected plants were carefully separated, washed with distilled water, and then dried under shade for two weeks. The dried plants were crushed and grounded with a blender to obtain the powder. Condensed powders, namely, 650 g of OP, 415 g of Sm, and 522 g of Zm, were extracted with 99% ethanol (13 L, 8 L, and 11 L, respectively) for 1 week to obtain ethanol extracts. The extraction process was repeated three times, and the extracts were concentrated by rotary evaporator under vacuum at 40 °C.
Then, crude extracts were suspended in water 30% and methanol 70%, and then filtered. Subsequently, fractionation was done consecutively with 3 × 2000 mL petroleum ether, 3 × 1000 mL chloroform, 3 × 650 mL ethyl acetate, and 3 × 160 mL n-butanol.
Enzyme inhibition procedure
Inhibition of α-glucosidase was evaluated according to the chromogenic method described by McCue et al. (Citation2005), with some modifications. The enzyme solution contained 5 μL of α-glucosidase (25 unit/mL) and 125 μL of phosphate buffer (pH 6.9, 0.1 M). p-Nitrophenyl-α-d-glucopyranoside (11 mM) in the same buffer (pH 6.9) was used as the substrate solution.
Test samples (20 μL) at various concentrations were mixed with enzyme solution in microplate wells and then incubated for 15 min at 37 °C. The reaction was started by adding 20 μL of substrate solution and then incubated for an additional 15 min. The reaction was terminated by adding 80 μL of 0.2 M sodium carbonate solution.
Absorbance of the wells was measured by a microplate reader at 405 nm, while the reaction system without plant extracts was used as thec ontrol. The system without α-glucosidase was used as the blank, and acarbose was used as the positive control. All determinations were performed in triplicate. The enzyme inhibitory rates of the samples were calculated as follows:
Kinetics of α-glucosidase inhibition
Inhibition modes of the samples against α-glucosidase were determined according to the method described by Kim et al. (Citation2005). Briefly, fixed amounts of α-glucosidase were incubated with increasing concentrations of its substrates (PNPG) at 37 °C for 15 min, in the absence or presence of samples (concentration equivalent to IC50). Reactions were terminated, and absorption was measured and converted to reaction by Lineweaver–Burk plot. All the determinations were performed in triplicate.
Statistical analysis
The data were expressed as the mean ± SD of three replicates. Analysis was performed using Graphpad Software and Excel 2010. One-way analysis of variance (ANOVA) and Tukey posttest were used to evaluate the possible differences among the means. p values ≤0.05 were considered as significant differences.
Results
In vitro α-glucosidase inhibition
Alcoholic extracts of Sm, Zm and Op and four different fractions of them were tested for α-glucosidase inhibitory property with application of colorimetric method. Sampling information including taxonomic status, date, and location of gathering the plants is presented in . The α-glucosidase inhibition potential of three extracts as well as their fractions were compared on the basis of their IC50 values, which are the concentrations that produce 50% inhibition under the researchers’s specific set of assay conditions (). Ethyl acetate fraction of Zm (ZMA) exhibited the highest inhibitory effect with an IC50 = 0.35 ± 0.01 mg/mL in comparison with other samples and standard. indicate the enzyme inhibition percentage versus increasing concentrations of the samples.
Figure 1. Inhibition of α-glucosidase by alcoholic extract of Salvia mirzayanii and its fractions at different concentrations.

Figure 2. Inhibition of α-glucosidase by alcoholic extract of Zataria multiflora and its fractions at different concentrations.

Figure 3. Inhibition of α-glucosidase by alcoholic extract of Otostegia persica and its fractions at different concentrations.

Table 1. Sampling information: taxonomic status, date and location of gathering.
Table 2. α-Glucosidase inhibitory potential (IC50) of the different samples (mg/ml) (Positive control: acarbose (IC50 = 11 ± 0.19 mM∼7 mg/ml).
α-Glucosidase kinetic studies
To characterize the inhibition process, i.e., competitive, noncompetitive, uncompetitive, or mixed, data are often analyzed by those set of techniques that linearize inherently non-linear relationships. One of the techniques is the Linewaver–Burk kinetic analysis. demonstrates the Km and Vmax values of the extracts against α-glucosidase. As compared with the uninhibited reaction, a decrease in Vmax was noted for all tested extracts; however, the effects of the extracts on Km were different, which means that Km values were reduced in presence of chloroform fraction of Zm ethanol extract (ZMC), chloroform fraction of Sm ethanol extract (SMC), ethyl acetate fraction of Sm ethanol extract (SMA), Sm ethanol extract (SME), and chloroform fraction of Op ethanol extract (OPC) and increased in presence of ZMA, petroleum ether fraction of Zm ethanol extract (ZMP), petroleum ether fraction of Sm ethanol extract (SMP), ethyl acetate fraction of Op ethanol extract of (OPA) and n-butanol fraction of Op ethanol extract (OPB) (). It is the first time that the inhibition mechanisms of α-glucosidase by Sm, Op, and Zm different fractions of ethanolic extracts were investigated according to these patterns (). The results showed that all of the samples may display patterns of mixed inhibition as uncompetitive-noncompetitive inhibitions.
Figure 4. Kinetic analysis of-glucosidase inhibition by different fractions of Salvia mirzayanii extract.

Figure 5. Kinetic analysis of α-glucosidase inhibition by different fractions of Zataria multiflora extract.

Figure 6. Kinetic analysis of α-glucosidase inhibition by different fractions of Otostegia persica extract.

Table 3. Kinetic parameters of α-glucosidase inhibition by plant extracts and their different fractions.
Discussion
There is a rising interest among researchers to specify new and effective α-glucosidase inhibitors with minimum side effects, which can be obtained from medicinal plants with acknowledged and scientifically proven antidiabetic properties (Grover et al. Citation2002; Onal et al. Citation2005; Bnouham et al. Citation2006). The present study investigated the inhibitory effects of Sm, Zm, and Op ethanol extracts as well as their four different fractions on α-glucosidase. Approximately, all of the samples, with exception of Zm ethanol extract (ZME) and ethanol extract Op (OPE), revealed inhibitory effects on α-glucosidase. This finding about ZME is in agreement with results presented in another study conducted by Gholamhoseinian et al. (Citation2008), which showed that Zm methanol extract had no effect on α-glucosidase inhibition. In three geniuses, inhibition effects of ethanol extracts were less than those of their fractions, which might be due to interactions taking place between compounds in the crude extracts. This finding was in contrast with the already reported results (Chen et al. Citation2013).
The IC50 of the three samples including SMB, ZMB, and OPP could not be determined since inhibition percentage of these samples was not dependent on the concentrations (). It means that by increasing the concentration, no increase was observed in inhibition. Moreover, at different concentrations of, petroleum ether fraction of Op ethanol extract (OPP), n-butanol fraction of Sm ethanol extract (SMB), and n-butanol fraction of Zm ethanol extract (ZMB), just 80%, 70%, and 30% inhibition were observed, respectively. The mentioned finding has been reported in another study, which examined α-amylase inhibitory effects of six Salvia species and reported no relationship between the concentration level and inhibition. In other words, as the concentrations of Salvia hydrangea, Salvia hypoleuca, and Salvia officinalis extracts increased, the enzyme inhibition presented no increase (Nickavar et al. Citation2010). In the present study, the samples with determination IC50 were categorized into 3 groups. Group 1 included ZMA, SMP, OPA and SMA, which exhibited very noticeable inhibition activities, and their inhibitory IC50 values were much less than acarbose as the standard (p < 0.001). Furthermore, there were not significant differences between their IC50 values (, p > 0.05). Group 2 included OPB, SMC, ZMP, and ZMC, which showed remarkable inhibition activities. Their IC50 values were less than acarbose (, p < 0.001); however, it must be mentioned that IC50 values were not less than those of the previous group. Moreover, significant differences were observed in their IC50 values (p < 0.01). Other researchers reported that inhibition of α-glucosidase by some plant extracts such as Susongsaengi-susu, Sikyung-susu, and Quercus brantii extracts (Azemi et al. Citation2015) is more than acarbose. Group 3 included OPC, whose IC50 value was not significantly different from that of the standard (p > 0.05) and SME, whose IC50 value was more than that of the standard (, p < 0.001). This finding is agreement with results presented in another study,which examined species of Salvia containing three flavonoids, namely, luteolin 7-O-glucoside, luteolin 7-O-glucuronide, and diosmetin 7-O-glucuronide. These compounds were isolated and showed inhibitory effects on α-glucosidase with IC50 values close to that of acarbose as the standard (Asghari et al. Citation2015). Phytochemical screening of the active components of OP species have been revealed that several types of diterpenoids and flavonols including morin, kaempferol, and quercetin are identified in this plant; moreover, two compounds such as morin and quercetin are responsible for antioxidant activities of OP methanol extract; therefore, these compounds may inhibit the α-glucosidase.
In addition to, components such as catechine and rosmarinic acid were separated from Salvia extracts by HPLC analysis (Asadi et al. Citation2011).
Also, in other research, in SMA, chemical components such as apigenin and kaempferol were identified (the data was not published) which these components may be responsible for inhibiting the activity of α-glucosidase. The essential oil of Zm was analyzed by GC/MS and the major compounds were thymul (26.32%) and carvacrol (25.51%); (Emamjomeh et al. Citation2014); moreover, three known compounds, dihydroxy aromadendrane, luteolin and α-tocopherol quinone, have also been isolated from Zm extract (Ali et al. Citation2000). In this study, three geniuses of Labiatae revealed very significant inhibitory effects on α-glucosidase (), and it seems that they enjoyed strong antidiabetic activities. Hence, with due attention to the antidiabetic compounds in the natural products (Arif et al. Citation2014; Benalla et al. Citation2010), these three plant extracts may contain antidiabetic compounds such as polyphenols, triterpenes, phytosterols, flavonoids, flavanones, genine derivatives, and anthocyanin (Ali et al. Citation2000; Lu & Foo Citation2002; Yassa et al. Citation2005; Sadeghi & Alizadeh Citation2013; Sajed et al. Citation2013; Sadeghi et al. Citation2014; Zarshenas & Krenn Citation2015).
Results from Lineweaver-Burk plots demonstrated that inhibition modes of all samples may be mixed inhibitions. Mixed inhibition may result in either a decrease in the apparent affinity of the enzyme for the substrate (Kmapp> Km), which means that Km value appears to increase in cases where the inhibitor favors binding to the free enzyme, or an increase in the apparent affinity (Kmapp< Km), which means that Km value appears to decrease when the inhibitor binds favorably to the enzyme–substrate complex. It is probable that the ability to bind to wide regions of the enzyme other than the active site enables these extracts as mixed inhibitors to reveal a broader specificity of inhibition compared with acarbose as a competitive inhibitor (Zhang et al. Citation2014).
One advantage of these extracts over acarbose is that in contrast with acarbose as a competitive inhibitor, these extracts (mixed inhibitors) may not be affected by higher concentrations of the substrate. It has been reported that with higher carbohydrate food intake, higher concentrations of acarbose as a competitive inhibitor would be required to present the same effect; however, with respect to mixed inhibition, the inhibitor would be still effective at lower concentrations (Ghadyale et al. Citation2011; Zhang et al. Citation2014).
As the association between phenolic compounds and inhibition of carbohydrate hydrolyzing enzymes has been revealed (Shobana et al. Citation2009), it seems that these samples’s mode of inhibition is due to presence of high levels of flavonoids compounds (Tadera et al. Citation2006; Zhang et al. Citation2014).
Researchers have described the following characteristics of α-glucosidase inhibitors (Azemi et al. Citation2015): (1) sugar (substrate)-mimic structures, (2) potency to establish ionic bonds with nucleophilic catalyzing residues, (3) transition state-like structures, (4) potency to construct hydrogen bonds with catalytic acid residues, (5) potency to construct ionic and hydrophobic interactions at sites other than the active site, and (6) potency to construct covalent bond with enzymes through an epoxy or aziridine group (Moorthy et al. Citation2012).
Further studies should be directed towards chemical isolation, purification, and characterization of these three extracts to elucidate the compounds responsible for inhibition potential as these may play significant role in the development of antidiabetic agents.
Conclusions
The results of this study could offer a basis for further investigation and isolation of active compounds with α-glucosidase inhibition from the Op, Sm, and Zm extracts. The knowledge about the mechanisms of inhibition by these extracts could lead to affluent application of plant chemicals as drug targets.
Acknowledgements
The authors thank Hormozgan University of Medical Sciences for offering the grant [Grant No.94102].
Disclosure statement
Financial support was provided by Hormozgan University of Medical Sciences, Iran. The authors declared that they have no conflicts of interest.
Additional information
Funding
References
- Akhilesh K. 2012. Inhibition of alpha-glucosidase by Acacia nilotica prevents hyperglycemia along with improvement of diabetic complications via aldose reductase inhibition. J Diabetes Metab. 6:1–7.
- Ali MS, Saleem M, Ali Z, Ahmad VU. 2000. Chemistry of Zataria multiflora (Lamiaceae). Phytochemistry. 55:933–936.
- Arif T, Sharma B, Gahlaut A, Kumar V, Dabur R. 2014. Antidiabetic agents from medicinal plants: a review. Chem Biol Lett. 1:1–13.
- Asadi S, Khodagholi F, Esmaeili MA, Tusi SK, Ansari N, Shaerzadeh F, Sonboli A, Ghahremanzamaneh M. 2011. Chemical composition analysis, antioxidant, antiglycating activities and neuroprotective effects of S. choloroleuca, S. mirzayanii and S. santolinifolia from Iran. Am J Chin Med. 39:615–638.
- Asghari B, Salehi P, Sonboli A, Ebrahimi SN. 2015. Flavonoids from Salvia chloroleuca with α-amylsae and α-glucosidase inhibitory effect. Iran J Pharm Res. 14:609–611.
- Azemi ME, Khodayar MJ, Ayatamiri FN, Tahmasebi L, Abdollahi E. 2015. Inhibitory activities of Phoenix dactylifera, Capparis spinosa, Quercus brantii, and Falcaria vulgaris hydroalcoholic extracts on α- amylase and α-glucosidase. Int J Curr Res Chem Pharm Sci. 2:19–25.
- Benalla W, Bellahcen S, Bnouham M. 2010. Antidiabetic medicinal plants as a source of alpha glucosidase inhibitors. Curr Diabetes Rev. 6:247–254.
- Bnouham M, Ziyyat A, Mekhfi H, Tahri A, Legssyer A. 2006. Medicinal plants with potential antidiabetic activity: a review of ten years of herbal medicine research (1990–2000). Int J Diabetes Metab. 14:1–25.
- Chen Y-G, Li P, Li P, Yan R, Zhang X-Q, Wang Y, Zhang X-T, Ye W-C, Zhang Q-W. 2013. A-glucosidase inhibitory effect and simultaneous quantification of three major flavonoid glycosides in Microctis folium. Molecules. 18:4221–4232.
- Coman C, Rugina OD, Socaciu C. 2012. Plants and natural compounds with antidiabetic action. Not Bot Horti Agrob. 40:314–325.
- Ebrahimpoor M, Khaksar Z, Noorafshan A. 2009. Antidiabetic effect of Otostegia persica oral extract on streptozotocin-diabetic rats. Res J Biol Sci. 4:1227–1229.
- Fernández-Mejía C. 2013. Oxidative stress and chronic degenerative diseases-a role for antioxidants. Mexico City: InTech.
- Emamjomeh L, Imani S, Talebi K-H, Moharramipour S, Larijani K. 2014. Chemical composition and insecticidal activity of essential oil of Zataria multiflora Boiss. (Lamiaceae) against Ephestia kuehniella (Lepidoptera: Pyralidae). Eur J Exp Biol. 4:253–257.
- Ghadyale V, Takalikar S, Haldavnekar V, Arvindekar A. 2011. Effective control of postprandial glucose level through inhibition of intestinal alpha-glucosidase by Cymbopogon martinii (Roxb.). Evid Based Complemen Alternat Med. 2012:1–6.
- Gholamhoseinian A, Falah H, Sharififar F, Mirtaj AS. 2008. The inhibitory effect of some Iranian plants extracts on the alpha-glucosidase. Iran J Basic Med Sci. 11:1–9.
- Grover JK, Yadav S, Vats V. 2002. Medicinal plants of India with anti-diabetic potential. J Ethnopharmacol. 81:81–100.
- Hajzadeh M, Rajaei Z, Ghamami G, Tamiz A. 2011. The effect of Salvia officinalis leaf extract on blood glucose in streptozotocin-diabetic rats. Pharmacol Online. 1:213–220.
- Johansen JS, Harris AK, Rychly DJ, Ergul A. 2005. Oxidative stress and the use of antioxidants in diabetes: Linking basic science to clinical practice. Cardiovasc Diabetol. 4:5.
- Kamatou G, Makunga N, Ramogola W, Viljoen A. 2008. South African Salvia species: a review of biological activities and phytochemistry. J Ethnopharmacol. 119:664–672.
- Keller MS. 1978. Mysterious herbs and roots: Ancient secrets for beautie health, magick, prevention and youth. Virginis: Peace Press.
- Kim Y-M, Jeong Y-K, Wang M-H, Lee W-Y, Rhee H-I. 2005. Inhibitory effect of pine extract on alpha-glucosidase activity and postprandial hyperglycemia. Nutrition. 21:756–761.
- Lima CF, Azevedo MF, Araujo R, Fernandes-Ferreira M, Pereira-Wilson C. 2006. Metformin-like effect of Salvia officinalis (common sage): Is it useful in diabetes prevention. Br J Nutr. 96:326–333.
- Lin Y, Sun Z. 2010. Current views on type 2 diabetes. J Endocrinol. 204:1–11.
- Lu Y, Foo LY. 2002. Polyphenolics of Salvia: a review. Phytochemistry. 59:117–140.
- Manzari-Tavakoli A, Pouraboli I, Yaghoobi MM, Mehrabani M, Mirtadzadini SM. 2013. Antihyperglycemic, antilipid peroxidation, and insulin secretory activities of Otostegia persica shoot extract in streptozotocin-induced diabetic rats and in vitro c187 pancreatic β-cells. Pharm Biol. 51:253–259.
- McCue P, Kwon Y-I, Shetty K. 2005. Anti‐amylase, anti‐glucosidase and anti‐angiotensin converting enzyme potential of selected foods. J Food Biochem. 29:278–294.
- Mohammadi A, Gholamhoseinian A, Fallah H. 2014. Zataria multiflora increases insulin sensitivity and pparγ gene expression in high fructose fed insulin resistant rats. Iran J Basic Med Sci. 17:263.
- Moorthy NS, Ramos MJ, A Fernandes P. 2012. Studies on α-glucosidase inhibitors development: Magic molecules for the treatment of carbohydrate mediated diseases. Mini Rev Med Chem. 12:713–720.
- Naghibi F, Mosaddegh M, Mohammadi Motamed M, Ghorbani A. 2010. Labiatae family in folk medicine in Iran: from ethnobotany to pharmacology. Iran J Pharm Res. 4:63–79.
- Najar AG, Fallah H, Sharififar F. 2009. Anti-hyperglycemic activity of four plants extracts effective against alpha-glucosidase in normal and diabetic rats. J Kerman Uni Med Sci. 16:35–44.
- Nickavar B, Abolhasani L, Izadpanah H. 2010. α-amylase inhibitory activities of six Salvia species. Iran J Pharm Res. 7:297–303.
- Onal S, Timur S, Okutucu B, Zihnioglu F. 2005. Inhibition of alpha-glucosidase by aqueous extracts of some potent antidiabetic medicinal herbs. Prep Biochem Biotechnol. 35:29–36.
- Sadeghi F, Alizadeh A. 2013. Phytochemical composition of the essential oil, total phenolic content and antioxidant activity in Salvia mirzayanii Rech. & Esfand. Grown wild and cultivated in Iran. Int Res J Appl Basic Sci. 6:977–982.
- Sadeghi Z, Akaberi M, Valizadeh J. 2014. Otostegia persica (Lamiaceae): A review on its ethnopharmacology, phytochemistry, and pharmacology. Avicenna J Phytomed. 4:79–88.
- Sajed H, Sahebkar A, Iranshahi M. 2013. Zataria multiflora Boiss. (Shirazi thyme)-an ancient condiment with modern pharmaceutical uses. J Ethnopharmacol. 145:686–698.
- Sepehri G, Khaksari M, Najar AG. 2014. The effect of water extract of Zataria multiflora on microvascular permeability in streptozocin induced diabetic rats. Annu Res Rev Bio. 4:3119.
- Shai L-J, Masoko P, Mokgotho M-P, Magano S-R, Mogale A, Boaduo N, Eloff J-N. 2010. Yeast alpha-glucosidase inhibitory and antioxidant activities of six medicinal plants collected in Phalaborwa, South Africa. S Afr J Bot. 76:465–470.
- Shobana S, Sreerama Y, Malleshi N. 2009. Composition and enzyme inhibitory properties of finger millet (Eleusine coracana L.) seed coat phenolics: Mode of inhibition of α-glucosidase and pancreatic amylase. Food Chem. 115:1268–1273.
- Tadera K, Minami Y, Takamatsu K, Matsuoka T. 2006. Inhibition of alpha-glucosidase and alpha-amylase by flavonoids. J Nutr Sci Vitaminol. 52:149–153.
- Tofighi Z, Alipour F, Goodarzi S, Yassa N, Hadjiakhoondi A, Hadavinia H. 2009. Phytochemical and antidiabetic investigations of Otostegia persica from Iran. Planta Med. 75:877–1094.
- Trojan-Rodrigues M, Alves T, Soares G, Ritter M. 2012. Plants used as antidiabetics in popular medicine in Rio Grande do Sul, southern Brazil. J Ethnopharmacol. 139:155–163.
- Verspohl E. 2012. Novel pharmacological approaches to the treatment of type 2 diabetes. Pharmacol Rev. 64:188–237.
- World Health Organization (WHO). 2003. WHO: Definition and diagnosis of diabetes mellitus and intermediate hyperglycemia [Internet]. c2003–2016. Geneva, Switzerland: Report of a WHO/IDF Consultation; [cited 2016 Jun 29]. Available from: http://www.who.int/diabetes/publications/en/
- Yassa N, Sharififar F, Shafiee A. 2005. Otostegia persica as a source of natural antioxidants. Pharm Biol. 43:33–38.
- Zargari A. 1990. Medicinal plants. Iran: Tehran University.
- Zarshenas MM, Krenn L. 2015. Phytochemical and pharmacological aspects of Salvia mirzayanii Rech. f. Esfand. J Evid Based Complementary Altern Med. 20:65–72.
- Zhang H, Wang G, Dong J. 2014. Inhibitory properties of aqueous ethanol extracts of propolis on alpha-glucosidase. J Evid Based Complement Alternat Med. 2015:1–8.