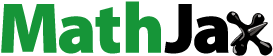
Abstract
Context: Sida acuta Burm.f. (Malvaceae) extracts are reported to have applications against malaria, diuretic, antipyretic, nervous and urinary diseases. No fungal endophytes of S. acuta are reported.
Objective: Isolation, identification and evaluation of antibacterial, antioxidant, anticancer and haemolytic potential of fungal endophytes from the ethnomedcinal plant S. acuta.
Materials and methods: Sida acuta stem segments were placed on PDA medium to isolate endophytic fungi. The fungus was identified by genomic DNA analysis and phylogenetic tree was constructed using ITS sequences (GenBank) to confirm species. The antibacterial efficacy of Aspergillus sulphureus MME12 ethyl acetate extract was tested against Gram-positive and Gram-negative pathogenic bacteria. DPPH free radical scavenging activity, anticancer and DNA fragmentation against EAC cells, and direct haemolytic activity (100–500 μg/mL) using human erythrocytes were determined.
Results and discussion: The ethyl acetate extract of A. sulphureus (Fresen.) Wehmer (Trichocomaceae) demonstrated significant antibacterial potential against Staphylococcus aureus, Bacillus subtilis, Escherichia coli and Salmonella typhi compared to streptomycin. MIC against test pathogens was in the range of 15.6–62.5 μg/mL. The antioxidant results revealed significant RSA from 12.43% to 62.02% (IC50 = 350.4 μg/mL, p ≤ 0.05). MME12 offered considerable inhibition of EAC proliferation (23% to 84%, IC50 = 216.7 μg/mL, p ≤ 0.05) supported by DNA fragmentation studies. The extract also offered insignificant haemolysis (5.6%) compared to Triton X-100.
Conclusions: A single endophytic fungus, A. sulphureus MME12 was isolated and identified using molecular profiling. The above-mentioned findings support the pharmacological application of A. sulphureus MME12 extract and demand for purification of the active principle(s).
Introduction
Natural products are a continuous source of new lead compounds, which can be utilized in various fields including pharmacy and agriculture. Unlike environmentally hazardous synthetic compounds, bioactive natural products retain an immense impact on modern medicine and agriculture (Deepika et al. Citation2016). Isolation of endophytes have carried out in a variety of plant species including those growing under extreme conditions, as the global diversity of endophytic fungi are limitless (Khan et al. Citation2011). However, still a large number of ecosystems need to be explored for such diversified endophytes and their medicinally important bioactive metabolites (Strobel Citation2006; Dutta et al. Citation2014; Zheng et al. Citation2016).
A comprehensive study has indicated that 51% of bioactive substances isolated from endophytic fungi were previously unknown (Schulz & Boyle Citation2005). Therefore, currently endophytic fungi are extensively explored for their ability to produce antimicrobial, anticancer, antioxidants, immunosuppressive compounds, in addition protect their host against various phytopathogens (Schulz & Boyle Citation2005; Saikkonen et al. Citation2006; Khan et al. Citation2011; Kumar et al. Citation2013; Dutta et al. Citation2014; Murali et al. Citation2016; Zheng et al. Citation2016). Endophytic fungi are also known to mimic the chemistry of their host plants and produce the same bioactive natural products, suggesting the possibility of inter-generic genetic exchange between the plant and the fungus (Kumar et al. Citation2013; Deepika et al. Citation2016).
Sida acuta Burm.f. (Malvaceae) is a medicinal plant with a broad range of phytochemicals such as tannins, saponins, flavonoids, alkaloids, organic acids and anthraquinones (Akaneme Citation2008), while the stem constitutes only tannins, alkaloids and anthraquinones (Edeoga et al. Citation2005). Sida acuta extracts find foremost applications against malaria, diuretic, antipyretic, nervous and also against urinary disease (Karou et al. Citation2007). Interestingly, no fungal endophytes of S. acuta are reported. Further, the metabolic constituents and their pharmacological applications need to be systematically explored. Therefore, the current study was conducted to isolate endophytic fungi from S. acuta and to evaluate their potential antibacterial, antioxidant and anticancer activities.
Materials and methods
Collection of plant material
The healthy and mature stem part of S. acuta was collected from Mysore region [Rainy season (July–September 2013)] (12.3°N 76.6°E, elevation 776 m), Karnataka, India and brought to the laboratory for immediate processing. The collected plant was identified by a Taxonomist (Prof. M.S. Sudarshana), Department of Studies in Botany, University of Mysore, Mysuru and authenticated with the help of Flora of Presidency of Madras (Gamble Citation1935). A Voucher Specimen No. MM2016 is deposited in the Herbarium, Department of Studies in Botany, University of Mysore, Manasagangotri, Mysore, Karnataka, India.
Isolation and identification of endophytic fungi
The collected stem samples were cut into small pieces (1–2 cm length) under aseptic conditions using a sterile scalpel and were subjected to surface sterilization by sequential steps (Rakshith et al. Citation2013). After sterilization, 8–10 stem segments were placed on Petri plates containing 20 mL of potato dextrose agar (PDA) medium supplemented with antibiotic chloramphenicol to avoid the emergence of endophytic bacteria. The Petri plates were sealed using Parafilm and incubated at 25 ± 2 °C for 3 weeks. Endophytic fungal colonies emerging from their host were sub-cultured onto the Petri plates containing PDA devoid of antibiotic to obtain pure cultures. The fungi were identified based on their morphological and cultural characteristics.
Molecular profiling of Aspergillus sp
Genomic DNA extraction
Genomic DNA was extracted from the lyophilized fungal mat of Aspergillus sp. MME12 by the cetyltrimethylammonium bromide (CTAB) method. The nuclear ribosomal DNA and internally transcribed spacer (ITS) region were amplified using ITS1 (5′- TCCGTAGGTGAACCTGCG-3′) and ITS4 (5′-TCCTCCGC-TTATTGATATG-3′) primers (White et al. Citation1990). The PCR conditions include; initial denaturation (95 °C for 10 min); 35 cycles [95 °C for 1 min, 55 °C for 1 min, 72 °C for 2 min] and a final extension at 72 °C for 8 min. The amplicon was sequenced using ITS1 and ITS4 primers in two different sequencing reactions. A contiguous sequence out of two sequences was generated using CAP3 sequence assembly program (Huang & Madan Citation1999) and submitted to the GenBank of the National Center for Biotechnology Information (NCBI).
Phylogenetic analysis
The phylogenetic tree was constructed using ITS sequences retrieved from GenBank included marine isolates of A. sulphureus, MME12 and other closely related Aspergillus spp. A distantly related isolate A. tubingensis was also used as an outgroup during analysis. The multiple sequence alignment was generated using the Clustal W version 1.7 (MSA) program. The phylogenetic dendrogram was generated by MEGA 6.0 software with a bootstrap consensus of 1000 replicates (Padhi & Tayung Citation2013).
Fermentation of endophytic fungus
Five to ten discs (10 mm) of A. sulphureus MME12 on PDA were picked and inoculated in 1000 mL Erlenmeyer flasks containing 500 mL of potato dextrose broth (PDB) for 4-6 weeks at 25 ± 2 °C under static conditions devoid of antibiotic. After incubation, the culture broth was filtered using Whatman No. 1 filter paper and the resulting culture filtrate was centrifuged at 5000 rpm for 10 min. The obtained supernatant was extracted with ethyl acetate and evaporated to dryness using flash evaporator. As ethyl acetate is nearly a polar solvent and immiscible with water during liquid–liquid extraction of secondary metabolites using separating funnel, it has been extensively used in endophyte extraction studies (Strobel et al. Citation1996; Schulz & Boyle Citation2005; Buatong et al. Citation2011).
Antibacterial activity
The antibacterial screening of the ethyl acetate extract was carried out by agar plug and disc diffusion method (Clinical and Laboratory Standards Institute (CLSI, 2012b)) against Gram-positive (Staphylococcus aureus MTCC 7443 and Bacillus subtilis MTCC 121) and Gram-negative (Escherichia coli MTCC 7410 and Salmonella typhi MTCC 733) bacteria obtained from Microbial Type Culture Collection and Gene Bank (MTCC), Institute of Microbial Technology, Chandigarh, India. About 100 μL of the test bacteria (1.5 × 108 CFU mL−1) were seeded uniformly onto the surface of NA media using a sterile glass spreader. The sterile discs (6 mm) were loaded with 50 μL of fungal extract (100 μg disc−1) and placed on NA plates. The inoculated plates were incubated at 37 ± 2 °C for 24 h and zone of inhibition around the discs were measured. The experiment was carried out in triplicates with appropriate controls.
Thin-layer chromatography and bioautography
The extract of A. sulphureus MME12 was subjected to TLC and agar overlay bioautography (Valgas et al. Citation2007). About 10 μL of ethyl acetate extract was spotted on pre-coated TLC silica gel plates (TLC Silica gel 60 F254, Merk, Germany) and air dried. The spotted TLC plates were eluted in an elution system of hexane and ethyl acetate (1:1). The developed chromatogram was air dried and observed in a UV chamber at 254 nm and 365 nm for clearly resolved bands and the corresponding Rf (Retention factor) values were calculated. The chromatograms were subjected to agar overlay bioautography against E. coli and the plates were incubated at 37 ± 2 °C for 24 h and observed for inhibition zone. The experiment was repeated thrice.
Minimum inhibitory concentration (MIC)
Minimal Inhibitory Concentration (MIC) was determined by broth microdilution technique (CLSI 2012a). The ethyl acetate extract was diluted to a concentration of 40 mg/mL, which served as a stock solution. Broth (100 μL) was dispensed into each well and a twofold serial dilution was carried out (2–0.0009 mg/mL). A 10 μL inoculum suspension was added to each well and incubated at 37 ± 2 °C for 24 h. Absorbance was measured at 620 nm using ELISA plate reader (LabTech 4000). MIC was also detected by adding 10 μL/well of TTC (2,3,5-triphenyl tetrazolium chloride at 2 mg/mL) and incubated for 30 min. The lowest concentration at which the colour change occurred was taken as the MIC value. All MIC tests were carried out in triplicates.
Antioxidant activity
The DPPH free radical scavenging activity (RSA) of A. sulphureus MME12 extract was determined by DPPH method (Sultanova et al. Citation2001). Different concentrations (100–500 μg/mL) of test sample were prepared, while the concentration of DPPH remained same. The reaction mixture containing 5 μL of extract and 95 μL of DPPH (300 μM) in methanol was incubated at 37 ± 2 °C for 30 min and the absorbance was measured at 517 nm. The experiment was repeated three times with ascorbic acid (HiMedia, Mumbai, India) as standard. The percent RSA was calculated by comparing with appropriate positive and negative controls.
Anticancer and DNA fragmentation assay
Short-term cytotoxicity was assessed by Trypan blue exclusion method (Gupta Citation2002). The in vitro cytotoxicity potentials of A. sulphureus MME12 extract was determined at various concentrations (100, 200, 300, 400 and 500 μg/mL) by dissolving it in 10% dimethyl sulfoxide. All the concentrations of the extract were mixed with 1 mL of Ehrlich Ascitic Carcinoma (EAC) cells (1 × 104 cells/mL) and incubated at 37 ± 2 °C for 4 h. After incubation, the Trypan blue dye (10 μL) was added to all the vials and the number of dead cells was counted using a haemocytometer. The cells that are viable exclude Trypan blue, while the uptake of trypan blue can be noticed in dead cells. The experiment was repeated three times with curcumin (Sigma-Aldrich, Bengaluru, India) as standard and the percentage of cytotoxicity was calculated.
The DNA fragmentation assay was carried out according to the method by McGahon et al. (Citation1995) with slight modifications. EAC cells (1 × 104 cells/mL) were incubated at 37 ± 2 °C for 4 h with A. sulphureus MME12 extract at a concentration of 500 μg/mL. Following incubation, the cell suspension was centrifuged at 2000 g (5 min at 4 °C) and DNA was isolated from pellets using DNA purification kit (HiMedia). Extracted DNA was dissolved in 50 μL TE buffer and electrophoresed on 1% agarose gel containing ethidium bromide and visualized under Gel-Documentation. The experiment was repeated three times.
Haemolytic assay
Haemolytic activity was carried out according to the method of Pagliara and Caroppo (Citation2012). In brief, about 10 mL of blood was collected from healthy volunteers following consent and was centrifuged at 3000 rpm for 3 min and the supernatant was discarded. Erythrocytes were washed three times using Dulbecco’s phosphate buffer saline (D-PBS) solution following centrifugation. The final pellet was diluted to 1:24 (v/v) in D-PBS, pH 7.0 containing 0.5 mM boric acid and 1 mM calcium chloride and brought to a final concentration of 5% (v/v).
For the haemolytic assay, various concentrations of endophytic extract (in triplicates) were incubated with erythrocytes suspension at 37 °C for 1 h. The free haemoglobin content in the supernatant was measured spectrophotometrically at 540 nm. D-PBS and 1% Triton X-100 (HiMedia, India) served as negative and positive controls, respectively. The experiment was repeated thrice and the per cent haemolytic activity was calculated using the formula:
Statistical analysis
Data from three replicates were analyzed for each experiment and analysis of variance (ANOVA) using SPSS Inc. 16.0. Significant effects of treatments were determined by F values (p ≤ 0.05). Tukey’s HSD test separated treatment means.
Results and discussion
Isolation and identification of endophytic fungi
A single endophytic fungus was isolated from S. acuta stem tissue, and no other fungal colonies emerged following imprint PDA plates that demonstrate active surface sterilization and true endophyte isolation. Similar, standardized endophytic fungal isolation by imprint method has been used in Ficus pumila, Basella rubra and Camellia sinensis to evaluate the effectiveness of surface sterilization of the plant materials (Rakshith et al. Citation2013; Hema et al. Citation2015; Guo et al. Citation2016). The results of the present study are in agreement with the findings of Rao et al. (Citation2015), where a single endophytic fungus Gliomastix polychrome was isolated from surface-sterilized bark of Combretum latifolium, while there are also reports of isolation of more than one endophytic fungi from Nymphaea nouchali (Dissanayake et al. Citation2016). Our fungal isolate emerging from the tissue of the inoculated plant part was identified as Aspergillus sp. MME12 by prominent morphological and cultural characteristics such as powdery texture, sulphur yellow with fewer spores on the surface and brownish on the reverse side with reduced sporulation ().
Figure 1. Characterization of endophytic fungus A. sulphureus MME12. (A) Colony and (B) conidial morphology of Aspergillus sp. MME12; (C) electropherograms of PCR amplification of A. sulphureus MME12; (D) Phylogenetic tree derived from Neighbour Joining analysis showing the evolutionary relationship of A. sulphureus MME12 with its closest BLAST hits. M: DNA marker; F: A. sulphureus MME12.

Molecular profiling of Aspergillus sp. MME12
Genomic DNA extraction and phylogenetic analysis
The PCR amplification of the isolated DNA from Aspergillus sp. MME12 with specific primers generated a band ranging from 550–600 base pair for the ITS region (). The ITS rDNA profiling is a standard and cost-effective method to identify the complex microbial communities in species level at exceptional depth and resolution following morphological and cultural examination (Rastogi & Sani Citation2011; Rao et al. Citation2015). Therefore, based on the ITS sequences, we identified the Aspergillus sp. MME12 as A. sulphureus. The DNA fragments were sequenced separately and deposited in NCBI-GenBank under the accession number KU234551. The ITS sequence of A. sulphureus MME12 (∼559 bp) revealed high similarity (99% sequence identity, E-value = 0) with the marine fungus A. sulphureus (JQ724467 and JQ724412). Further, phylogenetic analysis using NJ tree with 1000 bootstraps showed clustering based on species and ecological characters (). The alignment of the obtained ITS sequence with those of NCBI database resulted in identifying closely related sequences and NJ tree firmly demonstrated that A. sulphureus MME12 fell into the cluster of a marine A. sulphureus.
Antibacterial activity
The ethyl acetate extract of A. sulphureus MME12 was evaluated for antibacterial potential against a wide range of multi-drug-resistant Gram-positive (S. aureus and B. subtilis) and Gram-negative (E. coli and S. typhi) human pathogens by agar plug and disc diffusion methods. In agar plug method, the antibacterial activity zone of the A. sulphureus MME12 was comparable to standard control streptomycin (). Similarly, the results of antibacterial activity by disc diffusion assay offered 41–45 mm zone of inhibition against test pathogens (). Ramasamy et al. (Citation2010), with the ethyl acetate extracts of two endophytic Aspergillus sp. have shown parallel results against B. subtilis, E. coli, Micrococcus luteus, Pseudomonas aeruginosa and S. aureus. Likewise, Rao et al. (Citation2015) and Dissanayake et al. (Citation2016) reported significant antibacterial potential of endophytic fungus isolated from Combretum latifolium and Nymphaea nouchali, respectively.
TLC-bioautography
TLC-bioautography is considered to be one of the simplest and reproducible methods for the isolation of active metabolites from natural products (Patra et al. Citation2012; Rao et al. Citation2015; Balouiri et al. Citation2016). A TLC-based bioautography was carried out to separate the active metabolites of ethyl acetate extract of A. sulphureus MME12 and examine their antibacterial potential following separation. Hexane and ethyl acetate solvent system in the ratio of 1:1 was optimized based on pilot experiments. A total of 10 bands with Rf values of 0.07, 0.15, 0.25, 0.33, 0.47, 0.52, 0.57, 0.71, 0.77 and 0.88 were obtained (). A dark spot at the Rf value of 0.52 in TLC showed significant antibacterial potential with an effective inhibition zone against the test pathogen (). Correspondingly, TLC-bioautography has been used to check the antimicrobial potential of separated fractions of ethyl acetate extract of endophytic fungi (Rakshith et al. Citation2013; Rao et al. Citation2015).
Minimum inhibitory concentration (MIC)
MIC results of Aspergillus sp. MME12 ethyl acetate extract varied in the range of 15.6–62.5 μg/mL. The results revealed MIC of 15.6, 62.5, 15.6 and 62.5 μg/mL against S. aureus, B. subtilis, S. typhi and E. coli, respectively (). Likewise, ethyl acetate extract of endophytic fungi from Bacopa monnieri displayed antimicrobial activity against B. subtilis, P. aeruginosa, S. typhimurium, E. coli, K. pneumonia and S. aureus with MIC of 10–100 μg/mL (Katoch et al. Citation2014). Also, Ratnaweera et al. (Citation2015) and Dissanayake et al. (Citation2016) have reported that endophytic fungi Fusarium sp. and Chaetomium globosum isolated from Opuntia dillenii and N. nouchali, respectively, offered antibacterial activity with MIC ranging from 8 to 32 μg/mL against B. subtilis, S. aureus and methicillin-resistant S. aureus.
Figure 4. Bioactive potential of ethyl acetate extract of A. sulphureus MME12. (A) MIC against test pathogens; (B) DPPH radical scavenging activity; (C) cytotoxicity against Ehrlich Ascitic Carcinoma cells; (D) haemolytic activity against human erythrocytes; (E) DNA damage study against EAC cells. L1: DNA of EAC cells; L2: DNA of EAC cells treated with extract of A. sulphureus MME12. Each value is the mean for three replicates (n = 3) and bars sharing the same letters are not significantly different (p ≤ 0.05) according to Tukey’s HSD (honest significant difference). The vertical bar indicates the standard error.

Antioxidant activity by DPPH method
The ethyl acetate extract of MME12 was examined for its antioxidant activity by DPPH free Radical Scavenging Activity (RSA) method. The results revealed significant (p ≤ 0.05) RSA in A. sulphureus MME12 extracts from 12.43% to 62.02% (), with an IC50 at 350.4 μg/mL, while ascorbic acid offered 77% inhibition at 50 μg/mL. Khiralla et al. (Citation2015) isolated endophytic Aspergillus sp. from Trigonella foenum-graecum seeds, which provided antioxidant activity with an IC50 value of 18 μg/mL. Further, potent antioxidant activity has been observed in endophytic fungi isolated from Mimosa pudica, Tridax procumbens and Eugenia jambolana (Sharma & Sharma Citation2010; Yadav et al. Citation2014), which supports our findings.
Anticancer and DNA fragmentation assay
Cytotoxicity of A. sulphureus MME12 extract was determined against EAC cells using Trypan blue exclusion method by counting viable and non-viable cells in a haemocytometer. A spergillus sulphureus MME12 extract offered significant (p ≤ 0.05) inhibition of the proliferation of EAC cells with inhibition per cent ranging from 23% to 84% among the tested concentrations (), while the standard curcumin offered 87% inhibition at 10 μg/mL. The test extract was found to have an IC50 value of 216.7 μg/mL. Correspondingly, an endophytic fungus similar to Phoma sp. isolated from Cinnamomum mollissimum offered potent cytotoxicity against murine leucemia cells (Santiago et al. Citation2012). Also, the ethyl acetate extract of endophytic fungi isolated from P. crocatum and red alga Bostrychia tenella inhibited the growth of different tumour cell lines with IC50 value of 37.43 (T47D) and 1 μg/mL (SF-295), respectively (Astuti et al. Citation2014; de Felicio et al. Citation2015). It was also noted that EAC cells without the treatment of extract had no cytotoxicity effect in the present study. Further, the result of the DNA fragmentation assay evidences the influence of cytotoxicity due to damage of DNA in EAC cells treated with the extract (). The results are in corroboration with earlier findings of Ruma et al. (Citation2013), as DNA damage in super-coiled pBR322 plasmid cells were noticed upon treatment with the extracts of endophytic fungi. The above results clearly suggest that A. sulphureus MME12 extract is cytotoxic to cancer cells through DNA damage.
Haemolytic assay
Our findings have demonstrated the antioxidant and antimicrobial potential of A. sulphureus MME12 extract against most common and lethal human bacteria. Also, the anticancer and DNA damage study indicates its anticancer applications. Considering its potential, it becomes significantly relevant to determine the cytotoxicity of the extract against normal cells. Therefore, we determined the direct haemolytic activity using normal human erythrocytes. The extract was tested at various doses from 100–500 μg/mL in comparison to 1% Triton X-100. Encouragingly, the extract even at its maximum dose tested demonstrated insignificant haemolysis. The haemolysis was found to be 1.4 ± 0.8 to 5.6 ± 1.2% at 100–500 μg/mL concentrations, respectively, compared to 100% haemolysis in 1% Triton X-100, a known haemolytic agent (). Recent studies by Li et al. (Citation2016) showed the anticancer activity of an extract from B. ochroleuca M21 against liver (HepG2), gastric (SGC-7901) and colon (HT29) cancer cell lines in concentration ranges of 0.1–0.45 mg/mL. However, the extracts did not have cytotoxic activity on normal human liver HL-7702 cells at concentrations of 0.025–1.6 mg/mL indicating their selectivity for cancer cells. Along similar lines, our data further confirms the biological application of A. sulphureus MME12 extract for applications in pharmaceutical fields without harming the healthy cells of the body.
Conclusions
The present work is the first report on the incidence of endophytic fungi A. sulphureus inhabiting S. acuta. The ethyl acetate extract from A. sulphureus MME12 demonstrated significant antibacterial potential, antioxidant and anticancer activity comparable to the standard drugs. Further, the toxicity studies of the extract tested against human erythrocytes using a haemolytic assay show their nontoxic nature. The above results demand for purification of the novel active principle(s) from A. sulphureus MME12 and determination of their pharmacological application.
Amruthesh_KN_et_al_supplemental_content.zip
Download Zip (2.7 MB)Acknowledgements
The first author thanks UGC, New Delhi, for awarding Post-Doctoral Fellowship. The authors are also grateful to DST- FIST, IOE and UGC Govt. of India, New Delhi for facilities.
Disclosure statement
The authors report no conflicts of interest. The authors alone are responsible for the content and writing of this article.
Additional information
Funding
References
- Akaneme FI. 2008. Identification and preliminary phytochemical analysis of herbs that can arrest threatened miscarriage in Orba and Nsukka towns of Enugu State. Afr J Biotech. 7:6–11.
- Astuti P, Wahyono, Nababan O. 2014. Antimicrobial and cytotoxic activities of endophytic fungi isolated from Piper crocatum Ruiz & Pav. Asian Pac J Trop Biomed. 4:S592–S596.
- Balouiri M, Sadiki M, Ibnsouda SK. 2016. Methods for in vitro evaluating antimicrobial activity: a review. J Pharmaceutical Anal. 6:71–79.
- Buatong J, Phongpaichit S, Rukachaisirikul V, Sakayaroj J. 2011. Antimicrobial activity of crude extracts from mangrove fungal endophytes. World J Microbiol Biotechnol. 27:3005–3008.
- Clinical and Laboratory Standards Institute (CLSI). 2012a. Methods for Dilution Antimicrobial Susceptibility Tests for Bacteria that Grow Aerobically, Approved Standard, 9th ed., CLSI document M07-A9. Clinical and Laboratory Standards Institute, 950 West Valley Road, Suite 2500, Wayne, Pennsylvania 19087, USA.
- Clinical and Laboratory Standards Institute (CLSI). 2012b. Performance Standards for Antimicrobial Disk Susceptibility Tests, Approved Standard, 7th ed., CLSI document M02-A11. Clinical and Laboratory Standards Institute, 950 West Valley Road, Suite 2500, Wayne, Pennsylvania 19087, USA.
- de Felicio R, Pavao GB, de Oliveira AL, Erbert C, Conti R, Pupo MT, Furtado NA, Ferreira EG, Costa-Lotufo LV, Young MC, et al. 2015. Antibacterial, antifungal and cytotoxic activities exhibited by endophytic fungi from the Brazilian marine red alga Bostrychia tenella (Ceramiales). Rev Brasil Farmacog. 25:641–650.
- Deepika VB, Murali TS, Satyamoorthy K. 2016. Modulation of genetic clusters for synthesis of bioactive molecules in fungal endophytes: a review. Microbiol Res. 182:125–140.
- Dissanayake RK, Ratnaweera PB, Williams DE, Wijayarathne CD, Wijesundera RL, Andersen RJ, de Silva ED. 2016. Antimicrobial activities of endophytic fungi of the Sri Lankan aquatic plant Nymphaea nouchali and chaetoglobosin A and C, produced by the endophytic fungus Chaetomium globosum. Mycol. 7:1–8.
- Dutta D, Puzari KC, Gogoi R, Dutta P. 2014. Endophytes: Exploitation as a tool in plant protection. Braz Arch Biol Technol. 57:621–629.
- Edeoga HO, Okwu DE, Mbaebie BO. 2005. Phytochemical constituents of some Nigerian medicinal plants. Afr J Biotechnol. 4:685–688.
- Gamble JS. 1935. The Flora of the Presidency of Madras. vol. 3. Calcutta: BSI.
- Guo F, Li Z, Xu X, Wang K, Shao M, Zhao F, Wang H, Hua H, Pei Y, Bai J. 2016. Butenolide derivatives from the plant endophytic fungus Aspergillus terreus. Fitoterapia. 113:44–50.
- Gupta SK. 2002. Cytotoxicity Assays. In: Talwar GP, Gupta SK, editors. A handbook of practical and clinical immunology. New Delhi: CBS, p. 299–300.
- Hema P, Murali M, Thriveni MC, Prathibha M, Jayaramu SC, Amruthesh KN. 2015. Antibacterial activity of endophytic fungi isolated from Basella rubra L. - A medicinal plant. J Pure Appl Microbiol. 9:2971–2978.
- Huang X, Madan A. 1999. CAP3: A DNA sequence assembly program. Genome Res. 9:868–877.
- Karou SD, Wendyam MC, Nadembega Ilboudo DP, Ouermi D, Gbeassor M, De Souza C, Simpore J. 2007. Sida acuta Burm. f.: a medicinal plant with numerous potencies. Afr J Biotechnol. 6:2953–2959.
- Katoch M, Singh G, Sharma S, Gupta N, Sangwan PL, Saxena AK. 2014. Cytotoxic and antimicrobial activities of endophytic fungi isolated from Bacopa monnieri (L.) Pennell (Scrophulariaceae). BMC Complement Altern Med. 14:52.
- Khan AL, Hussain J, Al-Harrasi A, Al-Rawahi A, In-Jung L. 2011. Endophytic fungi: resource for gibberellins and crop abiotic stress resistance. Crit Rev Biotechnol. 35:62–74.
- Khiralla A, Mohamed I, Thomas J, Mignard B, Spina R, Yagi S, Laurain-Mattar D. 2015. A pilot study of antioxidant potential of endophytic fungi from some Sudanese medicinal plants. Asian Pac J Trop Med. 8:701–704.
- Kumar A, Patil D, Rajamohanan PR, Ahmad A. 2013. Isolation, purification and characterization of vinblastine and vincristine from endophytic fungus Fusarium oxysporum isolated from Catharanthus roseus. PLoS ONE. 8:e71805.
- Li Y, Guo S, Zhu H. 2016. Statistical optimization of culture medium for production of exopolysaccharide from endophytic fungus Bionectria ochroleuca and its antitumor effect in vitro. EXCLI J. 15:211–220.
- McGahon AJ, Seamus JM, Bissonette RP, Mahboubi A, Shi Y, Mogil RJ, Nishioka WK, Green DR. 1995. The end of the (cell) line: methods for the study of apoptosis in vitro. In: Schwartz LM, Osborne BA, editors. Cell death: methods in cell biology. Academic Press: New York, vol. 46:p. 153–185.
- Murali M, Hema P, Thriveni MC, Manjula S, Prathibha M, Jayaramu SC, Amruthesh KN. 2016. Antibacterial potential of fungal endophytes isolated from Boerhaavia diffusa L. J Appl Pharm Sci. 6:216–221.
- Padhi S, Tayung K. 2013. Antimicrobial activity and molecular characterization of an endophytic fungus, Quambalaria sp. isolated from Ipomoea carnea. Ann Microbiol. 63:793–800.
- Pagliara P, Caroppo C. 2012. Toxicity assessment of Amphidinium carterae, Coolia cfr. monotis and Ostreopsis cfr. ovata (Dinophyta) isolated from the northern Ionian Sea (Mediterranean Sea). Toxicon. 60:1203–1214.
- Patra JK, Gouda S, Sahoo SK, Thatoi HN. 2012. Chromatography separation, 1H NMR analysis and bioautography screening of methanol extract of Excoecaria agallocha L. from Bhitarkanika, Orissa, India. Asian Pac J Trop Biomed. 2:S50–S56.
- Rakshith D, Santosh P, Satish S. 2013. Isolation and characterization of antimicrobial metabolite producing endophytic Phomopsis sp. from Ficus pumila Linn. (Moraceae). Int J Chem Anal Sci. 4:156–160.
- Ramasamy K, Lim SM, Bakar AB, Ismail N, Ismail MS, Ali MF, Weber JFF, Cole ALJ. 2010. Antimicrobial and cytotoxic activities of Malaysian endophytes. Phytother Res. 24:640–643.
- Rao HY, Baker S, Rakshith D, Satish S. 2015. Molecular profiling and antimicrobial potential of endophytic Gliomastix polychroma CLB32 inhabiting Combretum latifolium Blume. Mycol. 6:176–181.
- Rastogi G, Sani RK. 2011. Molecular techniques to assess microbial community structure, function, and dynamics in the environment. In: Ahmad I, Ahmad F, Pichtel J, editors. Microbes and microbial technology: agricultural and environmental applications. New York: Springer, p. 29–57.
- Ratnaweera PB, de Silva ED, Williams DE, Andersen RJ. 2015. Antimicrobial activities of endophytic fungi obtained from the arid zone invasive plant Opuntia dillenii and the isolation of equisetin, from endophytic Fusarium sp. BMC Complement Alternat Med. 5:1–7.
- Ruma K, Sunil K, Prakash HS. 2013. Antioxidant, anti-inflammatory, antimicrobial and cytotoxic properties of fungal endophytes from Garcinia species. Int J Pharm Pharm Sci. 5:889–897.
- Saikkonen K, Lehtonen P, Helander M, Koricheva J, Faeth SH. 2006. Model systems in ecology: dissecting the endophyte-grass literature. Trends Plant Sci. 11:428–433.
- Santiago C, Fitchett C, Murray HG, Munro Jalil J, Santhanam J. 2012. Cytotoxic and antifungal activities of 5-hydroxyramulosin, a compound produced by an endophytic fungus isolated from Cinnamomum mollisimum. Evid Based Complement Alternat Med. 2012:Article ID 689310, 6 pages.
- Schulz B, Boyle C. 2005. The endophytic continuum. Mycol Res. 109:661–686.
- Sharma MC, Sharma S. 2010. Phytochemical and pharmacological screening of combined Mimosa pudica Linn. and Tridax procumbens for in vitro antimicrobial activity. Int J Microbiologi Res. 1:171–174.
- Strobel G, Yang X, Sears J, Kramer R, Sidhu RS, Hess WM. 1996. Taxol from Pestalotiopsis microspora, an endophytic fungus of Taxus wallachiana. Microbiology. 142:435–440.
- Strobel G. 2006. Harnessing endophytes for industrial microbiology. Curr Opin Microbiol. 9:240–244.
- Sultanova N, Makhmoor T, Abilov ZA, Parween Z, Omurkamzinova VB, Rahman A, Iqbal CM. 2001. Antioxidant and antimicrobial activities of Tamarix ramosissima. J Ethnopharmacol. 78:201–205.
- Valgas C, De Souza SM, Smania EFA, Smania AJ. 2007. Screening methods to determine antibacterial activity of natural products. Braz J Microbiol. 38:369–380.
- White TJ, Burns T, Lee S, Taylor J. 1990. Amplification and direct sequencing of fungal ribosomal genes for phylogenetics. In: Innis MA, Gelfand, DH, Shinsky J, White TJ, editors. PCR protocols: a guide to methods and application. USA, San Diego: Academic Press, p. 315–322.
- Yadav M, Yadav A, Yadav JP. 2014. In vitro antioxidant activity and total phenolic content of endophytic fungi isolated from Eugenia jambolana Lam. Asian Pac J Trop Med. 7:S256–S261.
- Zheng YK, Miao CP, Chen HH, Huang FF, Xia YM, Chen YW, Zhao LX. 2016. Endophytic fungi harbored in Panax notoginseng: diversity and potential as biological control agents against host plant pathogens of root-rot disease. J Ginseng Res. DOI: http://dx.doi.org/10.1016/j.jgr.2016.07.005