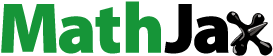
Abstract
Context: Turmeric (Curcuma longa L. [Zingiberaceae]) is used in the treatment of a variety of conditions including pesticide-induced toxicity.
Objective: The study reports the antioxidant properties and the protective effects of turmeric against carbofuran (CF)-induced toxicity in rats.
Materials and methods: The antioxidant potential was determined by using free radicals scavenging activity and ferric reducing antioxidant power values. Male Wistar rats were randomly divided into four groups, designated as control, turmeric (100 mg/kg/day), CF (1 mg/kg/day) and turmeric (100 mg/kg/day) + CF (1 mg/kg/day) treatments. All of the doses were administered orally for 28 consecutive days. The biological activity of the turmeric and CF was determined by using several standard biochemical methods.
Results: Turmeric contains high concentrations of polyphenols (8.97 ± 0.15 g GAEs), flavonoids (5.46 ± 0.29 g CEs), ascorbic acid (0.06 ± 0.00 mg AEs) and FRAP value (1972.66 ± 104.78 μM Fe2+) per 100 g of sample. Oral administration of CF caused significant changes in some of the blood indices, such as, mean corpuscular volume, corpuscular hemoglobin, white blood cell, platelet distribution width and induced severe hepatic injuries associated with oxidative stress, as observed by the significantly higher lipid peroxidation (LPO) levels when compared to control, while the activities of cellular antioxidant enzymes (including superoxide dismutase and glutathione peroxidase) were significantly suppressed in the liver tissue.
Discussion and conclusion: Turmeric supplementation could protect against CF-induced hematological perturbations and hepatic injuries in rats, plausibly by the up-regulation of antioxidant enzymes and inhibition of LPO to confer the protective effect.
Introduction
Carbofuran (CF) [2, 3-dihydro-2, 2-dimethyl-7-benzofuranyl-N methyl carbamate] is a major toxic carbamate pesticide and is widely used in agriculture and in many households. Food, water, and air contamination with CF has become a serious concern because of its adverse effects that pose hazards not only to humans, but also animals, wildlife, and fishes alike (de Siqueira et al. Citation2015; Ruiz-Suárez et al. Citation2015). Recent investigations suggest that CF has significant deleterious effects on the liver and kidney functions as well as on certain blood parameters (Islam et al. Citation2014; Jaiswal et al. Citation2015; Ojha & Gupta Citation2015). The insecticidal action of CF primarily relies on reversible inhibition of acetylcholinesterase (AChE) via carbamoylation of serine residue (Gupta et al. Citation2016). Oxidative stress has been proposed as an alternative mechanism of CF toxicity in certain animal tissues, via impairment of mitochondrial respiratory system that leads to increased generation of free radicals through lipid peroxidation of cell membrane which play significant role not only in the pathogenesis of neuronal diseases but also in non-neuronal complications (Kamboj et al. Citation2006; Kaur et al. Citation2012).
Since free radical generation is expected to induce organ toxicities including hepatotoxicity, supplementation with antioxidants may improve tissue capacity to cope with the high antioxidant demands (Samir et al. Citation2000; Tanvir et al. Citation2015a). Therefore, safe and effective natural products that may confer free radical scavenging activities are in global demand as an additional armamentarium against oxidative damage (Paul et al. Citation2016; Tanvir et al. Citation2015b; Yesiloglu & Aydin Citation2013).
Turmeric, referred to as the “Queen of spices” – is a rhizomatous herbaceous perennial plant (Curcuma longa L.) of the ginger family, Zingiberaceae. It is considered as a principal ingredient in many types of dishes originating from Bangladesh and India due to its attractive color, flavor, and taste (Gupta et al. Citation2013). Its rhizomes contain approximately 2% volatile oils, 5% curcuminoids as well as approximately 69.43% carbohydrate, 6.30% protein, 5.10% oil, 3.50% mineral, and other important elements in dry turmeric (Khan et al. Citation2015; Tayyem et al. Citation2006). It is also medicinally used for gastric, hepatic, gynecological or infectious diseases as well as cosmetics and dye in the textile industries (Gupta et al. Citation2013; Hasan & Mahmud Citation2014). The herbaceous perennial is extensively cultivated in the tropical areas of South Asia, including Bangladesh, India and China, with India being the primary exporter of turmeric (Gupta et al. Citation2013). However, there is lack of data on the antioxidant properties and medicinal benefits of the local turmeric from Bangladesh.
To date, although the toxic effects of CF are well documented (de Siqueira et al. Citation2015; King & Aaron Citation2015), insufficient information is available on the protective effect of turmeric against chronic or sub-chronic CF poisoning. Therefore, the current study was designed to investigate the antioxidant potentials and effects of turmeric against CF-induced hematological, biochemical and hepatic damage in rats.
Materials and methods
Pesticide and chemicals
CF (C12H15NO3, purity 98%) was purchased from Shetu Corporation Limited, Dhaka, Bangladesh while gallic acid, catechin, 1,1-diphenyl-2-picrylhydrazyl radical (DPPH) were purchased from Sigma-Aldrich (St. Louis, MO). All of the chemicals and reagents used in this study were of analytical grade.
Turmeric collection and extract preparation
Fresh and dry turmeric samples were collected from the Chittagong district of Bangladesh in July 2013. It was authenticated to be of the correct species by Professor Nuhu Alam from the Department of Botany, Jahangirnagar University, Bangladesh. Following collection, the turmeric samples were packed in sterile poly bags and were transported to the Laboratory of Preventive and Integrative Biomedicine in the Biochemistry and Molecular Biology department, Jahangirnagar University, Savar, Dhaka-1342, Bangladesh.
Turmeric samples were cleaned under running tap water and were dried in the shade for two days before being ground to a fine powder using a blender (CM/L7360065, Jaipan, Mumbai, India). Ethanol extract (20%) was prepared by adding turmeric powder (20 g) in 70% ethanol solution to make a 100 mL solution. The solution was shaken in a shaker for 72 h at room temperature. The solution was first filtered by sterile clothes followed by filtration using a Whatman No. 1 filter paper. Subsequently, the solvent was evaporated using a rotary evaporator under a reduced pressure (100 psi) at a controlled temperature (40 °C). The dried extracts were collected and were stored at −20 °C for subsequent experiments including analysis of antioxidant properties, in vivo effects on blood parameters and liver function markers. The yield of the extract was 21.95 g (17.93%), determined according to the following formula: % yield = [weight of sample extract/initial weight of sample] × 100.
Evaluation of antioxidant properties
Estimation of total polyphenol, flavonoid and ascorbic acid content
The total polyphenol content (TPC) of the turmeric extract was estimated by spectrometric determination according to Folin–Ciocalteu method (Shabana et al. Citation2017). A mixture of diluted solution (20 μL) of turmeric extract and 100 μL of Folin–Ciocalteu reagent (0.2 N) were prepared, followed by incubation at room temperature for 5 min and the addition of 80 μL of sodium carbonate solution (75 g/L in water). The absorbance was read at 765 nm after 1 h against a blank of water. A standard curve was prepared using different aliquots of gallic acid. All samples were analyzed three times using a PD-303 S spectrophotometer (APEL, Japan), and the mean values were obtained. The total polyphenol content present was determined as gallic acid equivalent (GAE) (6.25–200.00 μg/mL) and was expressed as g of GAEs/100 g of turmeric.
The total flavonoid content (TFC) was estimated using an aluminum chloride colorimetric assay (Chang et al. Citation2002; Shabana et al. Citation2017). A mixture of diluted solution (100 μL) of turmeric extract and 100 μL of aluminum trichloride (2% AlCl3) in methanol was prepared. The absorbance of the mixture was measured at 415 nm after 15 min. Methanol (100 μL) with extract (100 μL) without AlCl3 was as a blank. The standard curve was prepared using different aliquots of catechin (Sigma Chemical) as a reference compound. All the samples were analyzed three times, and the results were averaged. The total flavonoid content was determined as catechin equivalent (CE) (6.25–200.00 μg/mL) and was expressed as g of CEs/100 g of turmeric.
The ascorbic acid content (AAC) in turmeric sample was estimated by a method as established by Omaye et al. (Citation1979). The developed colored complex was monitored at 520 nm. Ascorbic acid concentration was determined as ascorbate equivalent (AEs) and was expressed as mg of ascorbate equivalents (AEs) per 100 g of turmeric sample.
DPPH free radical-scavenging activity
The antioxidant activities of all turmeric extracts were evaluated according to the DPPH radical-scavenging activity based on a method as established by Braca et al. (Citation2002). Briefly, 1 mL of the extract was mixed with 1.2 mL of 0.003% DPPH in methanol at varying concentrations (2.5–80.0 μg/mL). The percentage of DPPH inhibition was calculated using the following equation:
where, ADPPH is the absorbance of DPPH in the absence of a sample and AS is the absorbance of DPPH in the presence of either the sample or the standard (gallic acid).
DPPH scavenging activity is expressed as the concentration of sample required to decrease DPPH absorbance by 50% (IC50). The value can be graphically determined by plotting the absorbance (the percentage of inhibition of DPPH radicals) against the log concentration of DPPH and determining the slope of the nonlinear regression.
Ferric reducing antioxidant power (FRAP) assay
The FRAP assay was performed according to a method established by Benzie and Strain (Citation1999) as previously described by Afroz et al. (Citation2014). FRAP values expressed as micromoles of ferrous equivalent (μM Fe2+ per 100 g of sample).
Animals and experimental set-up
Male Wistar rats ranging from 150 to 155 g were housed at 25 ± 2 °C under 12 h cycles of dark and light and were allowed to access standard laboratory food and water ad libitum. All the animals were maintained in accordance with the Institutional Guidelines for the Care and Use of Animals for Scientific purposes.
After a one-week acclimatization period, a total of 28 rats were randomly divided into four groups (each containing 7 rats) and were maintained for the experimental period of 28 days, as follows:
Control: Animals received 0.5 mL olive oil/rat with normal diet for 28 days. This group served as a normal control group.
Turmeric: Animals received turmeric extract at 100 mg/kg/rat for 28 days and a normal diet. This group served as a positive control group.
CF: Animals received CF alone at 1 mg/kg (1/10 of lethal dose 50%, LD50) dissolved in olive oil (0.5 mL/rat) for 28 days. This group served as a negative control.
Turmeric + CF: Animals received turmeric extract (100 mg/kg) and CF (1 mg/kg) dissolved in olive oil (0.5 mL/rat) for 28 days and served as treatment group.
The dose of turmeric used in this study was based on the previous study (Prakash et al. Citation2014) while CF dose was according to Kaur et al. (Citation2012). During the investigation, the rats were observed for any abnormal clinical signs and death while body weight (BW) changes were monitored on a weekly basis. At the end of the experimental period, the rats in each group were sacrificed by deep anaesthetization with a ketamine hydrochloride injection (100 mg/kg). Blood samples (4 mL) were collected by using a heparinized syringe (5 mL) from the inferior vena cava. The blood was divided into two portions. The first portion (1 mL) was transferred into tubes containing ethylene diamine tetra acetic acid (EDTA) for hematological analysis while the remaining blood was placed into plain tubes at ambient temperature for 30 min before centrifugation at 2000 rpm for 10 min to yield the serum for subsequent biochemical analysis. In addition, the liver tissue was excised immediately from the surrounding tissues and was washed twice with ice-cold phosphate buffer saline (PBS), followed by weighting and storage at −20 °C. The relative organ weight was calculated by dividing the liver weight with the final body weight of each rat (Liu et al. Citation2004) according to following formula: relative organ weight (%) = wet organ weight/body weight ×100.
Evaluation of hematological parameters
Hematological parameters such as the red blood cells (RBC), white blood cells (WBC), lymphocytes (LYMPH), monocytes (MONO) and neutrophils (NEUT), hemoglobin (HB), hematocrit (HCT), mean corpuscular volume (MCV), mean corpuscular hemoglobin (MCH), mean corpuscular hemoglobin concentration (MCHC), red cell distribution width-standard deviation (RDW–SD), red cell distribution width-coefficient of variation (RDW-CV), platelets (PLT), mean platelet volume (MPV), platelet distribution width (PDW), and pro-calcitonin (PCT), platelet larger cell ratio (P-LCR)were analyzed based on established procedures using an automated Sysmex KX-21 hematology analyzer (XS 1000i, Sysmex Corporation Ltd., Japan).
Evaluation of biochemical parameters
Biochemical parameters for the liver function tests including serum alanine aminotransferase (ALT), aspartate aminotransferase (AST), alkaline phosphatase (ALP) and lactate dehydrogenase (LDH) activities, total cholesterol (TC) and high density lipoprotein cholesterol (HDL-C) levels were determined using an automated chemistry analyzer (Dimension EXL with LM Integrated Chemistry System, Siemens Medical Solutions Inc., Malvern, PA).
Oxidative stress parameter (LPO)
Malondialdehyde (MDA) levels were assayed to determine the lipid peroxidation (LPO) products in the liver tissues according to the method of Okhawa et al. (Citation1979). Briefly, tissue homogenate (0.2 mL) was mixed with 8.1% sodium dodecyl sulfate (0.2 mL), 20% acetic acid (1.5 mL) and 8% thiobarbituric acid (1.5 mL). The mixture was supplemented up to 4 mL with distilled water and was heated at 95 °C in a water bath for 60 min. After incubation, the tubes were cooled to room temperature, and the final volume was increased to 5 mL. A butanol:pyridine (15:1) mixture (5 mL) was added, and the contents were vortexed thoroughly for 2 min. After centrifugation at 3000 rpm for 10 min, the upper organic layer was aspirated, and its absorbance was read at 532 nm against a blank. The levels of MDA were expressed as nmol of thiobarbituric acid reactive substances (TBARS) per mg of protein.
The total protein in liver tissue homogenates was estimated by the method of Lowry et al. (Citation1951). Briefly, 0.2 mL of sample [digested with 0.1 N sodium hydroxide (NaOH)] was mixed with 2 mL of working reagent (a mixture of 2% sodium carbonate, 0.1 N NaOH, 1.56% copper sulfate and 2.37% sodium-potassium tartrate), and the reaction mixture was incubated for 10 min at room temperature. The addition of 1 N Folin–Ciocalteu’s phenol reagent (0.2 mL) was followed by 30 min of incubation at room temperature. Finally, the absorbance was measured at 660 nm using a spectrophotometer (APEL, Japan). Bovine serum albumin (BSA) was used as the standard.
Evaluation of antioxidant enzymes
The levels of endogenous antioxidant or anti peroxidative enzymes, such as superoxide dismutase (SOD) and glutathione peroxidase (GSH-Px) in the rat liver tissues were estimated using standard ELISA assay kits (CUSABIO, Baltimore, MD). To this end, the liver tissue homogenates were re-centrifuged at 12,000 rpm for 10 min at 4 °C using an Eppendorf 5415D centrifuges (Hamburg, Germany). Clean liver tissue supernatants were used for analysis. The levels of SOD and GSH-Px were expressed as pg/mL and mLU/mL, respectively.
Histopathological examination
The liver tissue were dissected out and washed immediately with saline following sacrifice. It was then fixed in 10% formalin. The fixed tissues were dehydrated in gradual ethanol (50–100%) and were embedded in paraffin wax. Sections of 5 μm thicknesses were then prepared using a rotary microtome following staining using hematoxylin and eosin dye for microscopic observation under alight microscope (MZ3000 Micros, St Veit/Glan, Austria). The pathologist performing histopathological evaluation was blinded to the treatment assignment of the different study groups.
Data analysis
All analyses were conducted in triplicate. The data were expressed as mean ± standard deviation (SD). The data were analyzed using SPSS (Statistical Packages for Social Science, version 22.0, IBM Corporation, Armonk, New York), GraphPad Prism, version 6, GraphPad Software, Inc., USA and Microsoft Excel 2013 (Redmond, Washington). Comparisons between the groups were performed using a One-way Analysis of Variance (ANOVA). Statistical analyses of the biochemical data were conducted using Duncan (for hematological analysis) and Tukey’s (for BW and biochemical analysis) post hoc tests. Significance level was fixed at p < 0.05.
Results
Antioxidant constituents
The TPC and TFC of turmeric were 8.97 ± 0.15 gGAEs and 5.46 ± 0.29 gCEs respectively while the AAC was 0.06 ± 0.00 mg AEs/100 g of sample.
Antioxidant activity
The antioxidant activity of turmeric was determined by both DPPH scavenging activity and the FRAP assays. The estimated IC50 value of turmeric for DPPH scavenging activity was 2.50 μg/mL when compared with standard gallic acid of 0.25 μg/mL (). The mean FRAP value was 1972.66 ± 104.78 μM Fe2+ per 100 g of sample.
Body weight
No death was observed in any of the experimental groups. shows the effects of turmeric and CF on the body and organ weights in different experimental groups. There was no significant difference in body weight (BW) gain in CF and turmeric treated rats, although CF alone treated rats had a significantly slight reduction in BW gain and increase in relative liver weights. Nevertheless, co-administration with turmeric extract followed by CF treatment ameliorated the body weight near to normalcy.
Table 1. The effects of turmeric and CF on BW and relative organ weights.
Hematological parameters
CF administration caused non-significant decrease in RBC, HB, HCT, MCHC, RDW-SD and RDW-SD levels but increase in MCV and MCH levels when compared with that of the normal control rats. However, co-treatment with turmeric caused significant increase in RBC and HB levels when compared to the rats from the negative control group while other hematological parameters remained unchanged (). Similarly, the WBC and lymphocyte levels were significantly higher in rats treated with CF alone and co-administration with turmeric again ameliorated these parameters. In addition, the monocyte and neutrophil counts were not significantly different between the experimental groups (). Meanwhile, there was also no significant change in PLT and PCT counts between the different groups, although significant changes in MPV, P-LCR levels were observed in animals which received turmeric and CF co-treatment while significant changes occur only in PDW for CF alone treated rats when compared to normal controls ().
Table 2. The effects of turmeric and CF administration on the erythrocytes count in rats.
Table 3. The effects of turmeric and CF administrations on the total and differential leucocytes count in rats.
Table 4. The effects of turmeric and CF administrations on the platelets count in rats.
Table 5. Semi-quantitative scoring of the architectural changes following the histopathological examination of the rat liver.
Biochemical parameters
No significant difference was observed in the biochemical parameters group animals which received only turmeric when compared with animals from the normal control. However, rats that were exposed to CF alone had significantly elevated levels of ALT, AST, ALP and LDH activities when compared with the control group. Nevertheless, co-administration of turmeric at 100 mg/kg significantly lowered the hepatic marker enzymes when compared to animals which received CF alone treated group ().
Figure 2. The effects of turmeric and CF on serum hepatic marker enzyme activities. Data are expressed as mean ± SD of seven animals per group. The bars with different superscript (a, b) denote significance differences based on an one-way ANOVA followed by Tukey’s multiple comparison tests.

A marked increase in circulating TC and HDL-C levels were observed in CF-treated rats (). Again, co-administration with turmeric significantly reduced the levels of TC while increasing HDL-C level when compared with the levels measured for the CF-exposed group. Administration of turmeric alone did not significantly affect cholesterol levels when compared with that of the normal control group.
Figure 3. The effects of turmeric and CF on serum cholesterol levels. Data are expressed as mean ± SD of seven animals per group. The bars with different superscript (a, b, c) denote significance differences based on an one-way ANOVA followed by Tukey’s multiple comparison tests.

Based on the investigation of oxidative stress biomarkers, there was a significant increase in LPO levels in the animals treated with CF alone, as evidenced by the increase in liver MDA levels compared to the control group. However, turmeric conferred a protective effect because animals co-treated with turmeric extract had significantly lower MDA levels when compared to the negative controls ().
Figure 4. The effects of turmeric and CF on liver LPO levels in normal and different treated rats. Data are expressed as mean ± SD of seven animals per group. The bars with different superscript (a, b, c) denote significance differences based on an one-way ANOVA followed by Tukey’s multiple comparison tests.

The effects of oral administration of turmeric for four weeks on antioxidant enzyme activities in liver tissues are shown in . The enzymes, such as SOD and GSH-Px activities were significantly decreased in animals which received CF only when compared with normal controls. However, co-administration with turmeric to CF-treated rats significantly ameliorated the levels of SOD, and GSH-Px enzymes when compared with rats treated with CF alone.
Figure 5. The effects of turmeric and CF on the activities of (A) superoxide dismutase (SOD) and (B) glutathione peroxidase (GSH-PX) in the liver tissue of normal and treated rats. Data are expressed as mean ± SD of seven animals per group. The bars with different superscript (a, b, c) denote significance differences based on an one-way ANOVA followed by Tukey’s multiple comparison tests.

Histomorphological examinations
Microscopic inspection of the liver tissues revealed that the livers of the animals from the control and turmeric groups had a regular organization of hepatocytes radiating from the central vein (CV) to the periphery of the lobule (). The nuclei of the hepatocytes also showed a normal vesicular assembly with a normal and uniform cytoplasm observed (). In contrast, the liver of the animals from CF-treated group showed some degenerative vicissitudes in the organ, including severe disruption of the cellular arrangement, degeneration of hepatocytes at the peripheral area of the CV and congestion in the CV associated with inflammatory infiltrates (). The Turmeric + CF group however, showed amelioration in all of the investigated histopathological features with moderate to mild degree of worsening of hepatocytes and a mild congestion of CV with inflammation observed ().
Figure 6. Photomicrographs of H & E (hematoxylin and eosin) stained sections of the liver of control rats and the rats treated with turmeric alone showing (A & B) normal histological structure of the central vein (CV) and surrounding hexagonal hepatocytes. (C) CF-treated rat livers showed severe disruption of the cellular arrangement radiating from the central vein (CV) and in the lobule (long black arrows), degeneration of hepatocytes at the peripheral area of the CV (short black arrows) and congestion in the CV associated with inflammatory infiltrates (white arrows). (D) A liver section of the animals treated with turmeric +CF, showing a remarkable degree of preservation in the cellular arrangement with only mild inflammation observed. [Magnification: 40X].
![Figure 6. Photomicrographs of H & E (hematoxylin and eosin) stained sections of the liver of control rats and the rats treated with turmeric alone showing (A & B) normal histological structure of the central vein (CV) and surrounding hexagonal hepatocytes. (C) CF-treated rat livers showed severe disruption of the cellular arrangement radiating from the central vein (CV) and in the lobule (long black arrows), degeneration of hepatocytes at the peripheral area of the CV (short black arrows) and congestion in the CV associated with inflammatory infiltrates (white arrows). (D) A liver section of the animals treated with turmeric +CF, showing a remarkable degree of preservation in the cellular arrangement with only mild inflammation observed. [Magnification: 40X].](/cms/asset/21fb9daf-171c-4db4-bc6d-5e8a2eb2c4a4/iphb_a_1345951_f0006_c.jpg)
Discussion
To our knowledge, this is the first study to demonstrate the protective effects of turmeric of the local variety in CF-induced toxicity by ameliorating hematological parameters, circulating cholesterols and cellular antioxidant enzymes along with inhibition of lipid peroxidation. Plant phenolics, flavonoids and ascorbic acids are important constituents that contribute to the functional quality, color and flavor of natural product which serve as powerful antioxidants due to their hydrogen or electron-donating ability to arrest the production of free radicals following oxidative stress (Tanvir et al. Citation2015a). To counteract the potential hazards of oxidative damage, dietary consumption of antioxidants may be regarded as the first line defense against highly reactive toxicants (Denre Citation2014). Our findings indicate that turmeric of the local variety serves as a useful reserve of phenolics, flavonoids and ascorbic acids, which are natural source of antioxidants and play a significant role as a singlet oxygen quencher and free radical scavenger that can minimize molecular damage to the cell. The findings from FRAP and DPPH assays further confirmed the high antioxidant potential of the investigated turmeric variety.
In the present study, changes in rats BW along with relative organ weights provided an imperative indication of CF-induced toxicity. CF-exposure for 28 consecutive days at 1 mg/kg caused slight decrease in rats BW which might either be due to the direct cytotoxic effects of the pesticides on somatic cells, and/or indirectly acting via the central nervous system which controls feed and water intake and regulate the endocrine system all of which result in decreased appetite and reduced absorption of nutrients from the gut (Venkatesanwarlu et al. Citation1997). Moreover, the increase in the relative liver weights following CF-exposure as observed in our study could be attributed to the relationship between the liver weight increase and toxicological effects such as edema or to the reduced BW gain in the experimental animals (Tanvir et al. Citation2015a, Citation2016). The rapid destruction of cell membrane as a result of lipid peroxidation might be the mechanism for CF toxicity (Mansour et al. Citation2009). The increased LPO as observed in the CF-treated rats supported these findings as also reported by a previous study (Adhikari et al. Citation2004). However, co-administration of turmeric to CF-treated animals ameliorated the body and liver weights, which could be attributed either to the phytochemical content in turmeric or its antioxidant properties which ameliorate the oxidative stress caused by CF administration.
Changes in hematological parameters can indicate the toxic effects of foreign compounds such as pesticides or plant extracts, as well as in determining the physiological and pathological status of the body (Olson et al. Citation2000). CF-exposure resulted in significant increase in MCV and MCH and slight decreases in RBC, HB, HCT, MCHC, RDW-SD and RDW-CV levels all of which may lead to anaemia. On the other hand, MCH reflects HB content and HB rate to RBC (Nussey et al. Citation1995) both of which decreased following CF administration indicating an episode of macrocytic normochromic anemia (Adhikari et al. Citation2004). In addition, the reduction in the erythrocyte counts (RBC, HB, HCT, RDW-SD and RDW-CV) may be attributed to hyperactivity of the bone marrow (Tung et al. Citation1975) leading to the production of red blood cells with impaired integrity which were easily destructed in the circulation or also termed as atrophied erythrocytes (Nussey et al.Citation1995). Our findings suggest that the decrease in RBC counts (i.e. microcytic hypochromic anaemia) occur due to excessive damage to the erythrocytes or inhibition of erythrocyte formation as also reported (Elsharkawy et al. Citation2013). However, co-administration of turmeric to CF-treated rats increased the RBC and HB levels, indicating immune stimulatory activity of the extract (Sahu Citation2004) rendering turmeric as a potential alternative armamentarium against anaemia. We also observed significant increase in total WBC and lymphocytes levels and a non-significant increase in the platelet count of CF-exposed rats, indicating an activation of the rat’s immune system to ameliorate CF-induced toxicity. It has been shown that leucocytosis may occur due to increased leukocyte mobilization and is directly proportional to the severity of the causative stress condition (Celik et al. Citation2009). Co-administration with turmeric however tended to normalize these levels to some extent in CF-treated rats.
The effects of CF and turmeric on the liver function were evaluated by the serum enzymes activities (including ALT, AST, ALP and LDH), used to determine hepatic dysfunction and directly implicated the extent of hepatic damage and toxicity (Tanvir et al. Citation2015a). Elevated levels of serum ALT, AST, ALP and LDH in serum indicated CF-induced hepatic injuries and disturbance in the biosynthesis of these enzymes. Alteration of the membrane permeability of the hepatocytes due to CF intoxication allows these enzymes to leak from the liver cytosol into the blood circulation, indicating necrosis and inflammatory reactions (Paul et al. Citation2016; Tanvir et al. Citation2015a). These observations matched with that of the recent study by Jaiswal et al. (Citation2015). Co-treatment of the rats with turmeric significantly ameliorated ALT, AST, ALP and LDH levels which can be attributed by the presence of high amount of phenolics, flavonoids and curcumin in turmeric extract all of which has inhibitory activities on membrane lipid peroxidation with robust free radical scavenging abilities (Milatovic et al. Citation2006; Saxena et al. Citation2009; Krup et al. Citation2013; Farkhondeh & Samarghandian Citation2016). Moreover, the elevated TC level in CF-treated rats could be due to blockage of the liver bile ducts causing significant reduction or cessation of its secretion into the duodenum subsequently leading to cholestasis (Tanvir et al. Citation2015a). The data corroborated with the results reported by Jaiswal et al. (Citation2013) in which rats were exposed to CF in sub-lethal doses for 30 days. The levels of TC and HDL-C in turmeric co-administered rats revealed that turmeric extract had significant capability to restore their levels when compared to the controls. This changes may be attributed to the inhibition of cholesterol absorption and biosynthesis, inhibition of biosynthesis of very-low-density lipoproteins, the precursor of LDL-C and acceleration of fractional turnover of LDL-C (Yoon et al. Citation2011) as well as the increased in excretion of bile acids through the active compounds in this turmeric (Vahouny et al. Citation1987).
LPO is a well-established mechanism for oxidative stress, leading to cell injury (Sankar et al. Citation2012). MDA is one of the most widely used indicators of the cellular redox state (Vincent & Taylor Citation2006) which was observed to be elevated in the rat’s liver receiving CF. Increased LPO appears to be the initial stage where the liver tissue becomes susceptible to oxidative damage. However, co-administration with turmeric significantly reduced the levels of lipid peroxides in CF-exposed rats suggesting its protective effects against oxidative damage. It is plausible that the active constituents in turmeric with antioxidant activities scavenge the free radicals and confer some protection on the liver tissue. Moreover, the presence of the endogenous antioxidant enzymatic defence is highly important for the amelioration of the free radical-mediated tissue injuries (Sankar et al. Citation2012).
SOD and GSH-Px are the primary free radical scavenging enzymes involved in the first-line cellular defence against oxidative injury before they form the more reactive hydroxyl radicals. In this study, CF-exposure significantly decreased the activities of SOD and GSH-Px in the liver tissues when compared to the control rats. The observed effects may be due to their increased utilization for scavenging free radicals and/or their inactivation by excessive CF oxidation. Similar observations were recorded in the rat’s kidney when the animals were exposed to CF (1 mg/kg) for consecutively 28 days (Kaur et al. Citation2012) and also in the rat’s brain stem when the animals were exposed to CF at two different doses, i.e. 15% (1.2 mg/kg) and 30% (2.4 mg/kg) of LD50 for 30 days (Jaiswal et al. Citation2016). Co-administration with turmeric, however, improved the activities of SOD and GSH-Px by scavenging superoxide and hydrogen peroxide produced by CF (Jaiswal et al. Citation2015, Citation2016). Curcumin, the main active ingredient of turmeric is potentially responsible in boosting these enzyme activities as confirmed by Jaiswal et al. (Citation2016). The enzyme levels were also higher in animals administrated with turmeric alone when compared with the control group thus clearly indicating that turmeric does not only neutralize the oxidative stress but also boosts the activity of the antioxidant enzymes in normal physiological conditions. Moreover, histomorphological changes observed in the liver tissues confirmed the hepato-protective effects of turmeric on CF-induced toxicity. CF-treated rat livers showed severe disruption of the cellular arrangement. On the other hand, the liver section of the animals treated with turmeric and CF, showed a remarkable degree of preservation in the cellular arrangement. The hypothesis to explain the protective action of turmeric extract in CF-induced histomorphological alternations is that curcuminoids, phenolics, flavonoids, ascorbic acids of this turmeric could scavenged the free radicals and inhibited oxidative damage and the consequent degeneration as well as necrosis of liver tissues (Saeidnia & Abdollahi Citation2013; Sankar et al. Citation2012).
Further study to elucidate the exact mechanism underlying the hepatic protection of turmeric and to identify the specific types of antioxidants present in turmeric is warranted.
Conclusions
Sub-acute exposure to CF has significant impact on some hematological and biochemical parameters while causing hepatic perturbation in rats. Supplementation with turmeric however ameliorates the toxic effects of CF in the blood and liver, which may contribute to the reduction in the oxidative stress induced by environmental toxicants. Turmeric has the potential to be used to protect against CF-induced hematological and hepatic toxicities in rats.
Disclosure statement
We declare that there is no conflict of interest.
Additional information
Funding
References
- Adhikari S, Sarkar B, Chatterjee A, Mahapatra CT, Ayyappan S. 2004. Effects of cypermethrin and carbofuran on certain hematological parameters and prediction of their recovery in a freshwater teleost, Labeorohita (Hamilton). Ecotoxicol Environ Saf. 58:220–226.
- Afroz R, Tanvir E, Islam M, Alam F, Gan SH, Khalil M. 2014. Potential antioxidant and antibacterial properties of a popular jujube fruit: apple kul (Zizyphus mauritiana). J Food Biochem. 38:592–601.
- Benzie IF, Strain JJ. 1999. Ferric reducing/antioxidant power assay: direct measure of total antioxidant activity of biological fluids and modified version for simultaneous measurement of total antioxidant power and ascorbic acid concentration. Meth Enzymol. 299:15–27.
- Braca A, Sortino C, Politi M, Morelli I, Mendez J. 2002. Antioxidant activity of flavonoids from Licania licaniaeflora. J Ethnopharmacol. 79:379–381.
- Celik I, Yilmaz Z, Turkoglu V. 2009. Hematotoxic and hepatotoxic effects of dichlorvos at sublethal dosages in rats. Environ Toxicol. 24:128–132.
- Chang C-C, Yang M-H, Wen H-M, Chern J-C. 2002. Estimation of total flavonoid content in propolis by two complementary colorimetric methods. J Food Drug Anal. 10:178–182.
- de Siqueira A, Salvagni FA, Yoshida AS, Goncalves-Junior V, Calefi AS, Fukushima AR, Spinosa Hde S, Maiorka PC. 2015. Poisoning of cats and dogs by the carbamate pesticides aldicarb and carbofuran. Res Vet Sci. 102:142–149.
- Denre M. 2014. The determination of vitamin C, total phenol and antioxidant activity of some commonly cooking spices crops used in West Bengal. Int J Plant Physiol Biochem. 6:66–70.
- Elsharkawy EE, Yahia D, El-Nisr NA. 2013. Sub-chronic exposure to chlorpyrifos induces hematological, metabolic disorders and oxidative stress in rat: attenuation by glutathione. Environ Toxicol Pharmacol. 35:218–227.
- Farkhondeh T, Samarghandian S. 2016. The hepatoprotective effects of curcumin against drugs and toxic agents: an updated review. Toxin Rev. 35:133–140.
- Gupta SC, Sung B, Kim JH, Prasad S, Li S, Aggarwal BB. 2013. Multitargeting by turmeric, the golden spice: From kitchen to clinic. Mol Nutr Food Res. 57:1510–1528.
- Gupta VK, Pathak A, Siddiqi NJ, Sharma B. 2016. Carbofuran modulating functions of acetylcholinesterase from rat brain in vitro. Adv Biol. 2016:3760967.
- Hasan M, Mahmud M. 2014. The contribution of turmeric research and development in the economy of Bangladesh: an ex-post analysis. Int J Agril Res Innov Tech. 4:1–10.
- Islam MS, Mohanta MK, Saha AK, Mondol A, Hoque MM, Roy AK. 2014. Carbofuran-induced alterations in body morphometrics and histopathology of liver and kidneys in the Swiss albino mice Mus Musculus L. IJSRES. 2:308.
- Jaiswal SK, Gupta VK, Siddiqi NJ, Pandey RS, Sharma B. 2015. Hepatoprotective effect of Citrus limon fruit extract against carbofuran induced toxicity in Wistar rats. Chinese J Biol. 2015:1–10.
- Jaiswal SK, Sharma A, Gupta VK, Singh RK, Sharma B. 2016. Curcumin mediated attenuation of carbofuran induced oxidative stress in rat brain. Biochem Res Int. 2016:1–7.
- Jaiswal SK, Siddiqi NJ, Sharma B. 2013. Carbofuran induced oxidative stress in rat heart: ameliorative effect of vitamin C. ISRN Oxidative Med. 2013:1–10.
- Kamboj A, Kiran R, Sandhir R. 2006. Carbofuran-induced neurochemical and neurobehavioral alterations in rats: attenuation by N-acetylcysteine. Exp Brain Res. 170:567–575.
- Kaur B, Khera A, Sandhir R. 2012. Attenuation of cellular antioxidant defense mechanisms in kidney of rats intoxicated with carbofuran. J Biochem Mol Toxicol. 26:393–398.
- Khan SA, Hussain M, Noureen N, Fatima S, Ane NU, Abbas Z. 2015. Yield performance of turmeric varieties intercropped with mulberry plantations. Am Eurasian J Agric Environ Sci. 15:2076–2079.
- King AM, Aaron CK. 2015. Organophosphate and carbamate poisoning. Emerg Med Clin North Am. 33:133–151.
- Krup V, Prakash L, Harini A. 2013. Pharmacological activities of turmeric (Curcuma longa Linn): a review. J Homeop Ayurv Med. 2:133.
- Liu Z, Li C, Li M, Li D, Liu K. 2004. The subchronic toxicity of hydroxysafflor yellow A of 90 days repeatedly intraperitoneal injections in rats. Toxicology. 203:139–143.
- Lowry OH, Rosebrough NJ, Farr AL, Randall RJ. 1951. Protein measurement with the Folin phenol reagent. J Biol Chem. 193:265–275.
- Mansour SA, Mossa AT, Heikal TM. 2009. Effects of methomyl on lipid peroxidation and antioxidant enzymes in rat erythrocytes: in vitro studies. Toxicol Ind Health. 25:557–563.
- Milatovic D, Gupta RC, Aschner M. 2006. Anticholinesterase toxicity and oxidative stress. Sci World J. 6:295–310.
- Nussey G, Van Vuren J, Du Preez H. 1995. Effect of copper on the haematology and osmoregulation of the Mozambique tilapia, Oreochromis mossambicus (Cichlidae). Comp Biochem Physiol C Pharmacol Toxicol Endocrinol. 111:369–380.
- Ohkawa H, Ohishi N, Yagi K. 1979. Assay for lipid peroxides in animal tissues by thiobarbituric acid reaction. Anal Biochem. 95:351–358.
- Ojha A, Gupta Y. 2015. Evaluation of genotoxic potential of commonly used organophosphate pesticides in peripheral blood lymphocytes of rats. Hum Exp Toxicol. 34:390–400.
- Olson H, Betton G, Robinson D, Thomas K, Monro A, Kolaja G, Lilly P, Sanders J, Sipes G, Bracken W, et al. 2000. Concordance of the toxicity of pharmaceuticals in humans and in animals. Regul Toxicol Pharmacol. 32:56–67.
- Omaye ST, Turnbull JD, Sauberlich HE. 1979. Selected methods for the determination of ascorbic acid in animal cells, tissues, and fluids. Meth Enzymol. 62:3–11.
- Paul S, Islam MA, Tanvir E, Ahmed R, Das S, Rumpa N-E, Hossen MS, Parvez M, Gan SH, Khalil MI. 2016. Satkara (Citrus macroptera) fruit protects against acetaminophen-induced hepatorenal toxicity in rats. Evid Based Complement Alternat Med. 2016:9470954.
- Prakash A, Khan S, Kumar D, Telang AG, Malik JK. 2014. Concurrent administration of curcumin mitigates arsenic-and chlorpyrifos-induced apoptosis in rat thymocytes. Adv Anim Vet Sci. 2:407–413.
- Ruiz-Suárez N, Boada LD, Henríquez-Hernández LA, González-Moreo F, Suárez-Pérez A, Camacho M, Zumbado M, Almeida-González M, del Mar Travieso-Aja M, Luzardo OP. 2015. Continued implication of the banned pesticides carbofuran and aldicarb in the poisoning of domestic and wild animals of the Canary Islands (Spain). Sci Total Environ. 505:1093–1099.
- Saeidnia S, Abdollahi M. 2013. Toxicological and pharmacological concerns on oxidative stress and related diseases. Toxicol Appl Pharmacol. 273:442–455.
- Sahu S. 2004. Antibacterial activity of plant extracts on fish microbial pathogens [dissertattion]. CIFA, Kausalyaganga, Bhubaneswar; p. 237.
- Samir A, Eman G, Talaat E, Somaia Z. 2000. Carbamate toxicity and protective effect of vit. A and vit. E on some biochemical aspects of male albino rats. Egypt J Hosp Med. 1:60–77.
- Sankar P, Telang AG, Manimaran A. 2012. Protective effect of curcumin on cypermethrin-induced oxidative stress in Wistar rats. Exp Toxicol Pathol. 64:487–493.
- Saxena PN, Anand S, Saxena N, Bajaj P. 2009. Effect of arsenic trioxide on renal functions and its modulation by Curcuma aromatica leaf extract in albino rat. J Environ Biol. 30:527–531.
- Shabana MH, Balbaa LK, Talaat IM. 2017. Effect of foliar applications of Zingiber officinale extracts on Origanum majorana. J Herbs Spices Med Plants. 23:89–97.
- Tanvir E, Afroz R, Chowdhury MAZ, Khalil MI, Hossain MS, Rahman MA, Rashid MH, Gan SH. 2015a. Honey has a protective effect against chlorpyrifos-induced toxicity on lipid peroxidation, diagnostic markers and hepatic histoarchitecture. Eur J Integr Med. 7:525–533.
- Tanvir E, Afroz R, Karim N, Mottalib M, Hossain M, Islam M, Gan SH, Khalil M. 2015b. Antioxidant and antibacterial activities of methanol extract of BAU kul (Ziziphus mauritiana), an improved variety of fruit from Bangladesh. J Food Biochem. 39:139–147.
- Tanvir EM, Afroz R, Chowdhury M, Gan SH, Karim N, Islam MN, Khalil MI. 2016. A model of chlorpyrifos distribution and its biochemical effects on the liver and kidneys of rats. Hum Exp Toxicol. 35:991–1004.
- Tayyem RF, Heath DD, Al-Delaimy WK, Rock CL. 2006. Curcumin content of turmeric and curry powders. Nutr Cancer. 55:126–131.
- Tung HT, Cook FW, Wyatt RD, Hamilton PB. 1975. The anemia caused by aflatoxin. Poult Sci. 54:1962–1969.
- Vahouny GV, Khalafi R, Satchithanandam S, Watkins DW, Story JA, Cassidy MM, Kritchevsky D. 1987. Dietary fiber supplementation and fecal bile acids, neutral steroids and divalent cations in rats. J Nutr. 117:2009–2015.
- Venkatesanwarlu P, Sharma B, Kalakumar B, Reddy K, Ravikumar P. 1997. Comparative evaluation of toxicity of carbaryl, cypermethrine and malathion of testifies in mice. Indian J Toxicol. 4:33–37.
- Vincent HK, Taylor AG. 2006. Biomarkers and potential mechanisms of obesity-induced oxidant stress in humans. Int J Obes Relat Metab Disord. 30:400–418.
- Yesiloglu Y, Aydin H. 2013. Evaluation of antioxidant activities of turmeric extracts from Turkey. Asian J Chem. 25:7199–7204.
- Yoon KN, Alam N, Lee JS, Cho HJ, Kim HY, Shim MJ, Lee MW, Lee TS. 2011. Antihyperlipidemic effect of dietary Lentinus edodes on plasma, feces and hepatic tissues in hypercholesterolemic rats. Mycobiology. 39:96–102.