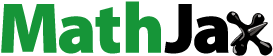
Abstract
Context
Peimine and paeoniflorin can be combined for the treatment of cough in paediatrics. The interaction during the co-administration could dramatically affect the bioavailability of drugs.
Objective
The interaction between peimine and paeoniflorin was investigated in this study.
Materials and methods
The pharmacokinetics of paeoniflorin (20 mg/kg) with or without the coadministration of peimine (5 mg/kg for 10 days before paeoniflorin) was orally investigated in Sprague-Dawley rats (n = 6). The group without the peimine was set as the control group. The metabolic stability of paeoniflorin was studied in rat liver with microsomes. The effect of peimine on the absorption of paeoniflorin was investigated with Caco-2 cell monolayers.
Results
The Cmax (244.98 ± 10.95 vs. 139.18 ± 15.14 μg/L) and AUC(0–t) (3295.92 ± 263.02 vs. 139.18 ± 15.14 h·μg/L) of paeoniflorin was increased by peimine. The t1/2 was prolonged from 5.33 ± 1.65 to 14.21 ± 4.97 h and the clearance was decreased from 15.43 ± 1.75 to 4.12 ± 0.57 L/h/kg. Consistently, peimine increased the metabolic stability of paeoniflorin with rat liver microsomes with the increased t1/2 (56.78 ± 2.62 vs. 26.33 ± 3.15 min) and the decreased intrinsic clearance (24.42 ± 3.78 vs. 52.64 ± 4.47 μL/min/mg protein). Moreover, the transportation of paeoniflorin was also inhibited by peimine as the efflux ratio decreased from 3.06 to 1.63.
Discussion and conclusions
Peimine increased the systemic exposure of paeoniflorin through inhibiting the activity of CYP3A4 and P-gp. These results provide a reference for further in vivo studies in a broader population.
Keywords:
Introduction
Due to the particularity of the patients, paediatric illnesses are always treated with mild drugs. Chinese traditional medicine is relatively mild, and the co-administration of different drugs can significantly improve therapeutic efficiency. Cough is symptomatic of a broad range of acute and chronic paediatric respiratory problems (Lamas et al. Citation2014). Peimine, a major bioactive alkaloid extracted from the herb Fritillaria thunbergii Miq (Liliaceae), is a commonly used antitussive and expectorant for the treatment of paediatric cough and pneumonia (Li et al. Citation1999, Citation2019). Additionally, peimine was also revealed to possess anti-inflammation and analgesic properties, making peimine widely used in the clinic (Park et al. Citation2017).
Paeonia lactiflora Pall. (Paeoniaceae) is also a commonly used cough remedy in paediatric cases that is well-known as the ‘Shaoyao Gancao Decoction’ (Chen et al. Citation2018). Paeoniflorin, the main bioactive compound isolated from Paeonia lactiflora (Chen et al. Citation2004), has been demonstrated to have anti-inflammatory and immunosuppressive effects (Xin et al. Citation2019). The therapeutic effect of paeoniflorin has been revealed in a variety of inflammatory disorder models, including rheumatoid arthritis, inflammatory bowel disease, psoriasis and asthma (Zhang et al. Citation2014; Chen et al. Citation2016; Jia and He Citation2016; Zhou et al. Citation2018). The pharmacokinetics of paeoniflorin could be affected by various drugs, such as glycyrrhizin, during the clinical co-administration (Sun et al. Citation2019).
Owing to the similar indication of peimine and paeoniflorin, these two compounds may be co-administrated in the clinic. Peimine was previously reported to exert inhibitory effects on the activity of various cytochrome P450 enzymes (CYP450s), including CYP3A4, 2E1 and 2D6, which are key mediators during the pharmacokinetics of various xenobiotics, including paeoniflorin (Li et al. Citation2020). Previously, the inhibition or induction of CYP450s was considered as the main cause of the adverse drug–drug interaction between different drugs, which has been widely reported (Geffrey et al. Citation2015; Yamamoto et al. Citation2017; Nishihara et al. Citation2019). It is speculated that the co-administration of peimine and paeoniflorin would induce drug–drug interaction, which might lead to failure of treatment or even drug toxicity.
Therefore, the co-administration of peimine and paeoniflorin was investigated in this study to determine the interaction between these two compounds and provide more guidance for the clinical application of drug co-administration.
Materials and methods
Chemicals
Peimine (>98%, Figure 1(A)), paeoniflorin (>98%, Figure 1(B)) and β-nicotinamide adenine dinucleotide phosphate (NADPH) were purchased from Sigma-Aldrich (St. Louis, MO). Pooled rat liver microsomes were obtained from BD Biosciences Discovery Labware (Woburn, MA). Acetonitrile and methanol were purchased from Merck (Darmstadt, Germany). Formic acid (HPLC grade) was purchased from Agilent Technologies (Santa Clara, CA). All reagents were prepared with Milli-Q water (18.2 megohms, Millipore, Billerica, MA). Other chemicals were of analytical grade or better.
Animals
The experiment was approved by the Animal Care and Use Committee of Hubei University of Medicine (IACUC-2019055). Adult male Sprague-Dawley rats weighed 220–250 g were obtained from Beijing HFK Bioscience Co. (Beijing, China). All animals were housed in a temperature and humidity-controlled environment and had free access to food and drink to acclimatize the facilities. Rats were fasted with free access to water for 12 h before the experiment.
Grouping and pharmacokinetic study
The experimental animals were randomly divided into two groups (the paeoniflorin group and the peimine + paeoniflorin group) of six animals each. The dosage of peimine and paeoniflorin was 5 and 20 mg/kg, respectively. For the peimine + paeoniflorin group, rats were orally treated with peimine for 10 days before the administration of paeoniflorin. The concentration of paeoniflorin in rat plasma was analysed at 0, 0.083, 0.25, 0.5, 1, 2, 3, 4, 6, 8, 12 and 24 h after administration with the help of LC–MS/MS.
Preparation of plasma samples
Aliquot of plasma samples (100 μL) were mixed with methanol and internal standard methanol in a polypropylene tube. After mixing for 60 s, the mixture was centrifuged at 12,000 rpm for min and removed the supernatant for further LC–MS/MS analysis.
Preparation of calibration standards and quality control samples
The standard stock solution of paeoniflorin was prepared in methanol at the concentration of 1 mg/mL. The concentration of calibration standards was 2, 6, 20, 60, 100, 200 and 400 μg/L. The quality control samples were prepared at the concentrations of 5, 50 and 300 μg/L. All solutions were kept at −20 °C and brought to room temperature before use.
LC–MS/MS condition
Agilent 1290 series liquid chromatography system and an Agilent 6460 triple-quadrupole mass spectrometer (Palo Alto, CA) were employed in this study. The chromatographic analysis was performed on a Waters X-Bridge C18 column (3.0 × 100 mm, i.d.; 3.5 μm, Milford, MA) at room temperature (25 °C). The mobile phase was water and acetonitrile with the 0.3 mL/min flow rate of isocratic elution and 4 min analysis time. The injection volume was 2 μL, and the auto-sampler temperature was maintained at 25 °C.
For the mass scan mode, the precursor ion and product ion were m/z 449.1 → 327.1 for paeoniflorin and m/z 463.1 → 300.0 for hyperoside as the internal standard, respectively (Supplementary Figure 1). The MS/MS conditions were optimized as follows: fragmentor, 120 V; capillary voltage, 4 kV; nozzle voltage, 500 V; nebulizer gas pressure (N2), 40 psig; drying gas flow (N2), 10 L/min; gas temperature, 350 °C; sheath gas temperature, 400 °C; and sheath gas flow, 11 L/min.
Metabolic stability experiments with rat liver microsomes
An NADPH-generating system containing 10 mM G-6-P, 1 mM NADP+, 4 mM magnesium chloride, 1 unit/mL of G-6-PDH, 30 μL rat liver microsome (20 mg/mL), 12 μL paeoniflorin solution (100 μM) and 1113 μL PBS buffer (0.1 M, pH 7.4) were mixed in the centrifuge tubes on ice. After a preincubation of 5 min, the reaction was initiated by adding 45 μL was increased-generating system into the microsomal suspension. Peimine (12 μL of 100 μM) was added to rat liver microsomes and incubated for 30 min followed by adding the NADPH-generating system to investigate the effect of peimine on the metabolic stability of paeoniflorin. After 0, 1, 3, 5, 15, 30 and 60 min of adding paeoniflorin, 100 μL aliquots were collected, and the concentration of paeoniflorin was analysed by LC-MS.
The in vitro half-life was calculated by the following equations:
Cell culture and Caco-2 Transwell model
The Caco-2 cells were purchased from ATCC (Manassas, VA) and cultured in DMEM high glucose medium with 15% FBS, 1% NEAA and 100 U/mL penicillin and streptomycin. Cell culture was conducted at 37 °C with 5% CO2.
1 × 105 cells/cm2 were seeded into Transwell polycarbonate insert filters with the pore size of 0.4 μm (Corning Costar Corporation, Cambridge, MA) and cultured for 21 days. In the first seven days, the medium was replaced freshly every two days, and then daily. The transepithelial electrical resistance (TEER) of the monolayer cells was measured using Millicell ERS-2 (Millipore Corporation, Billerica, MA), the cells can be used for the transport experiment when TEER exceeding 400 Ω·cm2.
Before the transport experiment, the Caco-2 cell monolayers were washed with warm (37 °C) Hanks’ balanced salt solution (HBSS) twice and incubated at 37 °C for 20 min. Then, the cell monolayers were incubated with paeoniflorin in fresh medium at 37 °C. The incubation volume of apical (AP) and basolateral (BL) was 0.5 and 1.5 mL, respectively. After incubating for the indicated time, 100 μL aliquot of the incubation solution was withdrawn and replaced with fresh HBSS (37 °C). Verapamil (50 μM) was added to both sides of cell monolayers and incubated for 30 min to inhibit the activity of P-gp. The permeability of paeoniflorin (10 μM) in all of the above conditions for both directions, i.e., from the AP side to the BL side and from the BL side to the AP side, was measured after incubation for 30, 60, 90 and 120 min at 37 °C. The efflux activity of P-gp was validated using a typical P-gp substrate rhodamine (10 μM).
The values of Papp and efflux ratio were calculated with the following equation:
where Papp BA is the apparent permeability coefficient from the BL side to the AP side (cm/s) and Papp AB is the apparent permeability coefficient from the AP side to the BL side (cm/s). ΔQ/Δt (μmol/s) is the rate at which the compound appears in the receiver chamber, C0 (μM) is the initial concentration of the compound in the donor chamber and A (cm2) represents the surface area of the cell monolayer.
Effect of peimine on the activity of CYP3A4
An incubation system of 200 μL including rat liver microsomal protein, sodium phosphate buffer (pH 7.4), MgCl2, testosterone, and various dosages of peimine (0, 2, 10, 25, 50, 150 and 300 μM) was used to evaluate the effect of peimine on the activity of CYP3A4. Reactions were started with the addition of NADPH after a pre-incubation of 5 min. After incubating for 30 min at 37 °C, ice-cold acetonitrile was added to terminate the reaction. Then, the mixture was centrifugated and analysed with HPLC.
Data analysis
Data were represented as mean ± SD obtained from triplicate experiments. The pharmacokinetic parameters, including the area under the plasma concentration–time curve (AUC), maximal plasma concentration (Cmax), the time for the maximal plasma concentration (Tmax), and the total clearance (CL/F) were calculated with the DAS 3.0 pharmacokinetic software (Chinese Pharmacological Association, Beijing, China). Paired Student’s t-test was performed to evaluate the difference between groups with the help of SPSS 20.0 (Chicago, IL). Differences were considered to be statistically significant when p< 0.05.
Results
Effect of peimine on the pharmacokinetic of paeoniflorin
The pharmacokinetic profile of paeoniflorin was investigated with or without the pre-treatment of peimine in vivo. As shown in , there is a significant difference between the paeoniflorin group and the peimine + paeoniflorin group in the plasma concentration–time curves of paeoniflorin.
Figure 3. The transport of paeoniflorin from apical (AP) to basolateral (BL) side and the opposite direction with the treatment of verapamil or peimine. Each symbol represents the mean ± SD of three determination. Papp BA is the apparent permeability coefficient from the BL side to the AP side (cm/s) and Papp AB is the apparent permeability coefficient from the AP side to the BL side.

The pharmacokinetic parameters of these two groups showed that after the oral administration of 20 mg/kg paeoniflorin, paeoniflorin was absorbed rapidly with the maximum plasma concentration (Cmax) of 139.18 ± 15.14 μg/L reached 1.78 ± 0.31 h (Tmax). The pre-treatment of peimine notably changed the pharmacokinetic profile of paeoniflorin. In the peimine + paeoniflorin group, the Cmax of paeoniflorin was reached at 5.14 ± 0.26 h with an increased value of 244.98 ± 10.95 μg/L, compared with the paeoniflorin group. The area under the curve (AUC(0–t)) was 1245.71 ± 115.82 h·μg/L, while it significantly increased to 3295.92 ± 263.02 h·μg/L after co-administrated with peimine. Moreover, the half-life (t1/2) of paeoniflorin was obtained as 5.33 ± 1.65 h, which was dramatically prolonged to 14.21 ± 4.97 h by the pre-treatment of peimine. For the clearance (CL/F) of paeoniflorin, peimine significantly suppressed the CL/F of paeoniflorin from 15.43 ± 1.75 to 4.12 ± 0.57 L/h/kg (p< 0.05, ).
Table 1. Pharmacokinetic parameters of 20 mg/kg paeoniflorin in the presence or absence of 5 mg/kg peimine.
Effect of peimine on the metabolic stability of paeoniflorin with rat liver microsomes
The metabolic stability of paeoniflorin in the presence or absence of peimine was investigated with the rat liver microsomes in vitro. The half-life of paeoniflorin in the rat liver microsomes was detected as 26.33 ± 3.15 min and the intrinsic clearance rate was 52.64 ± 4.47 μL/min/mg protein. The pre-treatment of peimine significantly prolonged the t1/2 to 56.78 ± 2.62 min with the intrinsic clearance rate markedly decreased to 24.42 ± 3.78 μL/min/mg protein (p< 0.05, ).
Effect of peimine on the transport of paeoniflorin with the Caco-2 cell model
The Papp AB value and the Papp BA value of paeoniflorin in the absence of peimine were 6.26 × 10−7 and 1.92 × 10−6 cm/s, respectively, and the efflux ratio was calculated as 3.06. In the presence of verapamil, a specific inhibitor of P-gp activity, the value of Papp AB of paeoniflorin increased to 9.94 × 10−7 cm/s, while the value of Papp BA decreased to 1.13 × 10−6 cm/s with the efflux ratio of 1.14. Additionally, peimine significantly inhibited the influx ration of paeoniflorin from 3.06 to 1.63 with Papp AB and Papp BA value of 8.34 × 10−7 and 1.36 × 10−6 cm/s, respectively (p< 0.05, ).
Effect of peimine on the activity of CYP3A4
In the presence of various dosages of peimine in rat liver microsomes, the activity of CYP3A4 was significantly reduced. Meanwhile, the inhibitory effect of peimine increased with the concentration of peimine ().
Discussion
Peimine and paeoniflorin are critical extracts of commonly used drugs in paediatrics for the treatment of paediatric cough and pneumonia (Zhang et al. Citation2020). Due to the similar pharmacological properties of these two compounds, such as anti-inflammation (Pae et al. Citation2002; Xu et al. Citation2016), they are always existed in the same prescription to improve the therapeutic efficiency and recovery rate. In the co-administration of different kinds of drugs, adverse drug–drug interaction always occurred, which would lead to treatment failure and even toxicity (Choi et al. Citation2011; Ji et al. Citation2013). Here, the co-administration of peimine and paeoniflorin was investigated in vivo and in vitro to declaim the potential drug–drug interaction between these two drugs and give guidance for the clinical medication of peimine and paeoniflorin.
The co-administration of peimine and paeoniflorin was found to influence the pharmacokinetic profile of paeoniflorin, of which the corresponding pharmacokinetic parameters, including t1/2, Cmax, Tmax and CL/F, were significantly different in the co-administrated group and the single-use group. The prolonged t1/2, increased Cmax and AUC(0–t), and the decrease CL/F of paeoniflorin in the presence of peimine indicated that the metabolism of paeoniflorin was inhibited by peimine. Consistently, the results of in vitro experiments in rat liver microsomes showed that peimine increased the metabolic stability of paeoniflorin with the increasing t1/2 and the decreasing intrinsic clearance rate of paeoniflorin. Previously, a variety of drug–drug interactions have been demonstrated to be associated with the effect of co-administrated drugs on the activity of CYPs. For example, the inhibitory effect of ketoconazole on the activity of CYP3A4 affected the pharmacokinetics of panobinostat, but it did not induce adverse clinical events. It was still necessary to monitor the panobinostat related adverse event (Hamberg et al. Citation2011). Glycyrrhizin was also demonstrated to inhibit the metabolism of paeoniflorin by inhibiting CYP3A4, which suggested the involvement of CYP3A4 in the metabolism of paeoniflorin (Sun et al. Citation2019). A previous study has reported that peimine was identified as an inhibitor of CYP3A4 with the IC50 of 13.43 μM (Li et al. Citation2020). Here, we demonstrated the inhibitory effect of peimine on the activity of CYP3A4 in rat liver microsomes and found that the inhibitory effect increased with the concentration of peimine (). Therefore, it was speculated that the inhibitory effect of peimine on the metabolism of paeoniflorin was a result of the inhibition of CYP3A4. The dosage of peimine and paeoniflorin during the co-administration should draw special attention.
P-gp is an important transporter that participates in the transport of various drugs and also plays a vital role in the drug–drug interaction between different types of drugs (Balayssac et al. Citation2005; Aszalos Citation2007). In the drug–drug interaction between glycyrrhizin and paeoniflorin, the activity of P-gp was also considered as a key factor that mediated the effect of glycyrrhizin on the pharmacokinetics of paeoniflorin (Sun et al. Citation2019). In our study, P-gp was revealed to mediate the transport of paeoniflorin as evidenced by the decreased efflux ratio by verapamil. In the presence of peimine, the efflux ratio of paeoniflorin was also suppressed, which is a bit higher than that in the presence of verapamil. Chen et al. previously reported the absorption of peimine involved the transporter P-gp (Chen L et al. Citation2016). Therefore, the inhibition in the transportation of paeoniflorin by peimine may result from the competition between peimine and paeoniflorin for P-gp.
Conclusions
The co-administration of peimine and paeoniflorin induced drug–drug interaction that increased the systemic exposure of paeoniflorin. The in vivo and in vitro experiments showed that the interaction between peimine and paeoniflorin may be the result of the inhibition of metabolism and transport of paeoniflorin. Peimine exerted these effects by inhibiting the activity of CYP3A4 and P-gp, which are responsible for the metabolism and absorption of paeoniflorin. Clinically, special attention should be paid to the dose of drugs during the combined medication of peimine and paeoniflorin.
Supplemental Material
Download ()Disclosure statement
No potential conflict of interest was reported by the author(s).
References
- Aszalos A. 2007. Drug–drug interactions affected by the transporter protein, P-glycoprotein (ABCB1, MDR1). I. Preclinical aspects. Drug Discov Today. 12(19–20):833–837.
- Balayssac D, Authier N, Cayre A, Coudore F. 2005. Does inhibition of P-glycoprotein lead to drug–drug interactions? Toxicol Lett. 156(3):319–329.
- Chen D, Zhao J, Cong W. 2018. Chinese herbal medicines facilitate the control of chemotherapy-induced side effects in colorectal cancer: progress and perspective. Front Pharmacol. 9:1442–1452.
- Chen F, Lu HT, Jiang Y. 2004. Purification of paeoniflorin from Paeonia lactiflora Pall. by high-speed counter-current chromatography. J Chromatogr A. 1040(2):205–208.
- Chen L, Lu X, Liang X, Hong D, Guan Z, Guan Y, Zhu W. 2016. Mechanistic studies of the transport of peimine in the Caco-2 cell model. Acta Pharm Sin B. 6(2):125–131.
- Chen T, Fu LX, Zhang LW, Yin B, Zhou PM, Cao N, Lu YH. 2016. Paeoniflorin suppresses inflammatory response in imiquimod-induced psoriasis-like mice and peripheral blood mononuclear cells (PBMCs) from psoriasis patients. Can J Physiol Pharmacol. 94(8):888–894.
- Choi YH, Chin YW, Kim YG. 2011. Herb–drug interactions: focus on metabolic enzymes and transporters. Arch Pharm Res. 34(11):1843–1863.
- Geffrey AL, Pollack SF, Bruno PL, Thiele EA. 2015. Drug–drug interaction between clobazam and cannabidiol in children with refractory epilepsy. Epilepsia. 56(8):1246–1251.
- Hamberg P, Woo MM, Chen LC, Verweij J, Porro MG, Zhao L, Li W, van der Biessen D, Sharma S, Hengelage T, et al. 2011. Effect of ketoconazole-mediated CYP3A4 inhibition on clinical pharmacokinetics of panobinostat (LBH589), an orally active histone deacetylase inhibitor. Cancer Chemother Pharmacol. 68(3):805–813.
- Ji HY, Liu KH, Kong TY, Jeong HU, Choi SZ, Son M, Cho YY, Lee HS. 2013. Evaluation of DA-9801, a new herbal drug for diabetic neuropathy, on metabolism-mediated interaction. Arch Pharm Res. 36(1):1–5.
- Jia Z, He J. 2016. Paeoniflorin ameliorates rheumatoid arthritis in rat models through oxidative stress, inflammation and cyclooxygenase 2. Exp Ther Med. 11(2):655–659.
- Lamas A, Ruiz de Valbuena M, Maiz L. 2014. Cough in children. Arch Bronconeumol. 50(7):294–300.
- Li H, Hung A, Li M, Yang AWH. 2019. Fritillariae thunbergii bulbus: traditional uses, phytochemistry, pharmacodynamics, pharmacokinetics and toxicity. Int J Mol Sci. 20(7):1667–1698.
- Li M, Liu X, Wang Y, Ju X. 2020. In vitro effects of peimine on the activity of cytochrome P450 enzymes. Xenobiotica. 50(10):1202–1207.
- Li SL, Chan SW, Li P, Lin G, Zhou GH, Ren YJ, Chiu FC. 1999. Pre-column derivatization and gas chromatographic determination of alkaloids in bulbs of Fritillaria. J Chromatogr A. 859(2):183–192.
- Nishihara M, Yamasaki H, Czerniak R, Jenkins H. 2019. In vitro assessment of potential for CYP-inhibition-based drug–drug interaction between vonoprazan and clopidogrel. Eur J Drug Metab Pharmacokinet. 44(2):217–227.
- Pae HO, Oh H, Choi BM, Oh GS, Paik SG, Jeong S, Hwang KM, Yun YG, Chung HT. 2002. Differentiation-inducing effects of verticinone, an isosteroidal alkaloid isolated from the bulbus of Fritillaria ussuriensis, on human promyelocytic leukemia HL-60 cells. Biol Pharm Bull. 25(11):1409–1411.
- Park JH, Lee B, Kim HK, Kim EY, Kim JH, Min JH, Kim S, Sohn Y, Jung HS. 2017. Peimine inhibits the production of proinflammatory cytokines through regulation of the phosphorylation of NF-κB and MAPKs in HMC-1 cells. Pharmacogn Mag. 13(Suppl. 2):S359–S364.
- Sun H, Wang J, Lv J. 2019. Effects of glycyrrhizin on the pharmacokinetics of paeoniflorin in rats and its potential mechanism. Pharm Biol. 57(1):550–554.
- Xin Q, Yuan R, Shi W, Zhu Z, Wang Y, Cong W. 2019. A review for the anti-inflammatory effects of paeoniflorin in inflammatory disorders. Life Sci. 237:116925.
- Xu J, Zhao W, Pan L, Zhang A, Chen Q, Xu K, Lu H, Chen Y. 2016. Peimine, a main active ingredient of Fritillaria, exhibits anti-inflammatory and pain suppression properties at the cellular level. Fitoterapia. 111:1–6.
- Yamamoto T, Furihata K, Hisaka A, Moritoyo T, Ogoe K, Kusayama S, Motohashi K, Mori A, Iwatsubo T, Suzuki H. 2017. Notable drug–drug interaction between etizolam and itraconazole in poor metabolizers of cytochrome P450 2C19. J Clin Pharmacol. 57(11):1491–1499.
- Zhang J, Dou W, Zhang E, Sun A, Ding L, Wei X, Chou G, Mani S, Wang Z. 2014. Paeoniflorin abrogates DSS-induced colitis via a TLR4-dependent pathway. Am J Physiol Gastrointest Liver Physiol. 306(1):G27–G36.
- Zhang L, Cui M, Chen S. 2020. Identification of the molecular mechanisms of peimine in the treatment of cough using computational target fishing. Molecules. 25(5):1105–1116.
- Zhou H, Wu Q, Wei L, Peng S. 2018. Paeoniflorin inhibits PDGF-BB-induced human airway smooth muscle cell growth and migration. Mol Med Rep. 17(2):2660–2664.