Abstract
Context
Polyporus polysaccharide (PPS), the leading bioactive ingredient extracted from Polyporus umbellatus (Pers.) Fr. (Polyporaceae), has been demonstrated to exert anti-bladder cancer and immunomodulatory functions in macrophages.
Objective
To explore the effects of homogeneous Polyporus polysaccharide (HPP) on the proliferation and autophagy of bladder cancer cells co‐cultured with macrophages.
Materials and methods
MB49 bladder cancer cells and RAW264.7 macrophages were co-cultured with or without HPP intervention (50, 100, or 200 μg/mL) for 24 h. The cell counting kit-8 (CCK-8) assay and 5-ethynyl-2″-deoxyuridine (EdU) staining evaluated MB49 cell proliferation. Monodansylcadaverine (MDC) staining and transmission electron microscopy (TEM) observed autophagosomes. Western blotting detected the expression levels of autophagy-related proteins and PI3K/Akt/mTOR pathway proteins.
Results
HPP inhibited the proliferation of MB49 cells co-cultured with RAW264.7 cells but not MB49 cells alone. HPP altered the expression of autophagy-related proteins and promoted the formation of autophagosomes in MB49 cells in the co-culture system. Autophagy inhibitors 3-methyladenine (3-MA) and chloroquine (CQ) not only antagonized HPP-induced autophagy but also attenuated the inhibitory effects of HPP on MB49 cell proliferation in the co-culture system. HPP or RAW264.7 alone was not sufficient to induce autophagy in MB49 cells. In addition, HPP suppressed the protein expression of the PI3K/Akt/mTOR pathway in MB49 cells in the co-culture system.
Discussion and conclusions
HPP induced bladder cancer cell autophagy by regulating macrophages in the co-culture system, resulting in the inhibition of cancer cell proliferation. The PI3K/Akt/mTOR pathway was involved in HPP-induced autophagy in the co-culture system.
Keywords:
Introduction
Tumor microenvironment (TME) is a complex environment in which tumor exists. The TME consists of immune cells, stromal cells, the extracellular matrix, and soluble factors such as cytokines, chemokines, and growth factors (Anderson and Simon Citation2020; Xiao and Yu Citation2021). During the course of tumor progression, interactions between the TME and cancer cells can drive tumor proliferation, angiogenesis, metastasis, and immune escape (Casey et al. Citation2015; Roma-Rodrigues et al. Citation2019). Recently, the TME has been a focus in the field of cancer, including bladder cancer. Tumor-associated macrophages (TAMs), considered essential drivers of cancer progression and metastasis, are the most abundant innate immune population in the TME. Accumulating evidence suggests that the density of TAMs is highly associated with poor prognosis in bladder cancer (Nielsen and Schmid Citation2017; Leblond et al. Citation2021). Given the immunogenicity of bladder cancer (Crispen and Kusmartsev Citation2020; van Wilpe et al. Citation2020), modulating immune cells, especially macrophages, in the TME seems to be a promising strategy for the treatment of bladder cancer.
Polyporus polysaccharide (PPS) is the major active component originally isolated from Polyporus umbellatus (Pers.) Fr. (Polyporaceae), which has various biological effects, including anti-bladder cancer and immunomodulatory activity (Zhang et al. Citation2011; Liu et al. Citation2017). Homogeneous Polyporus polysaccharide (HPP) was obtained by further purification of PPS (Liu et al. Citation2020). In our previous study, we found that HPP stimulated macrophage polarization toward the M1 phenotype, leading to the secretion of pro-inflammatory cytokines, such as TNF-α, IL-6, and IL-1β (Jia et al. Citation2021; Liu et al. Citation2022). In addition, HPP-stimulated macrophages promote apoptosis of bladder cancer cells, and inhibit their proliferation, migration, and epithelial-mesenchymal transition (Jia et al. Citation2021). However, the specific mechanism of action of HPP-stimulated macrophages in bladder cancer remains to be elucidated.
There is growing evidence that several pro-inflammatory cytokines, such as TNF-α, IL-6, and IL-1β, can induce autophagy (Shen et al. Citation2017; Ge et al. Citation2018; Yuan et al. Citation2018; Hu et al. Citation2021). Autophagy is a highly conserved process of cellular self-consumption that maintains cellular homeostasis by degrading cytoplasmic organelles and macromolecules, and recycling catabolic products (Mizushima & Komatsu 2011; Parzych & Klionsky 2014). Autophagy mainly occurs in four stages: induction, autophagosome formation, autophagosome-lysosome fusion, and final degradation (Levy et al. Citation2017). Autophagy-related proteins, such as Beclin1, LC3, and p62, are involved in the autophagy process (Lv et al. Citation2021). Autophagy is regulated by various signaling pathways, among which the PI3K/Akt/mTOR pathway is the key regulator (Sun et al. Citation2013; Heras-Sandoval et al. Citation2014). Previous studies have illustrated that autophagy acts as a tumor suppressor to prevent the development of cancer (Li et al. Citation2020). Therefore, we hypothesized that autophagy may be a critical mechanism by which HPP-stimulated macrophages act against bladder cancer.
In this study, we used a co‐culture system to mimic the interaction between bladder cancer cells and macrophages in the TME. The investigation focused on the effects of HPP on the proliferation and autophagy of bladder cancer cells, as well as exploring the association between proliferation and autophagy. Our findings reveal a novel therapeutic mechanism of HPP on bladder cancer.
Materials and methods
Materials
Dulbecco’s Modified Eagle Medium (DMEM), fetal bovine serum (FBS), and phosphate-buffered saline (PBS) were procured from Thermo Fisher Scientific, USA. 3-Methyladenine (3-MA; S2767) was supplied by Selleck, USA. Chloroquine (CQ; HY-17589A) was purchased from MedChemExpress, USA. The cell counting kit-8 (CCK-8) (C0038), and the monodansylcadaverine (MDC) staining kit (C3018S) were acquired from Shanghai Beyotime Biotechnology Co. LTD, China. The 5-ethynyl-2″-deoxyuridine (EdU) staining kit (C10310-1) was purchased from Guangzhou RiboBio Co. LTD, China. RIPA (9806S), anti-PI3K p85 (4257S), anti-Akt (4691S), anti-phospho-Akt (Ser473) (4060S), anti-mTOR (2983S), anti-phospho-mTOR (Ser2448) (5536S), anti-SirT1 (9475S), anti-Beclin1 (3495S), anti-p62 (5114S) and anti-GAPDH (5174S) antibodies were provided by Cell Signaling Technology, USA. LC3 antibody (14600-1-AP) and horseradish peroxidase (HRP)-linked goat anti-rabbit IgG (SA00001-2) were purchased from Proteintech, USA. The enhanced chemiluminescence solution kit (WBKLS0500) was obtained from Millipore, USA.
Preparation of HPP
HPP was prepared as previously described (Liu et al. Citation2020). The HPP obtained an invention patent authorized by the United States (Patent No. US 10723811B2). The average molecular weight of the HPP was 6.88 kDa.
Cell cultures
MB49 bladder cancer cells and RAW264.7 macrophages were originally obtained from American Type Culture Collection (ATCC) and preserved in our laboratory. The cells were maintained in DMEM supplemented with 10% FBS, 100 U/mL penicillin, and 100 μg/mL streptomycin. The medium was refreshed every two days until the cells reached 70–80% confluence for subculturing or subsequent experiments. The cells were incubated in a humidified atmosphere at 37 °C and 5% CO2.
Co‐culture experiment
MB49 cells and RAW264.7 cells were co-cultured in a 1:1 ratio using a Transwell Permeable Support System (0.4 µm pore-size, Corning, USA). The cells were co-cultured in fresh medium with or without HPP treatment (50, 100, or 200 μg/mL) for 24 h. 3-MA and CQ were used as inhibitors of autophagy. MB49 cells were pre-treated with 5 mM 3-MA for 4 h or 50 μM CQ for 3 h, and then co-cultured with RAW264.7 cells in fresh medium containing 100 μg/mL HPP.
CCK-8 assay
MB49 cells were harvested and seeded in 96-well plates at a density of 2 × 104 cells/mL. Five parallel wells were used for each treatment group. After incubation for 24 h, 10 μL of CCK-8 solution was added to each well, and the cells were cultured at 37 °C for 4 h. The absorbance was measured using a microplate reader (BioTek, Winooski, VT, USA) at 450 nm, and the cell proliferation activity was determined.
EdU staining assay
EdU staining was performed according to the manufacturer’s instructions. After Apollo and Hoechst fluorescence staining, photographs were taken under an inverted fluorescence microscope (Nikon, Tokyo, Japan). The percentage of EDU-positive (proliferating) cells was calculated using the formula: (EDU-positive cells/total number of cells) × 100%.
Western blotting analysis
Western blotting was performed to detect the expression levels of autophagy-related proteins and PI3K/Akt/mTOR pathway proteins in MB49 cells. To obtain total protein extracts, MB49 cells were lysed by RIPA buffer containing protease and phosphatase inhibitors. The protein concentration was quantified using a bicinchoninic acid assay. Protein samples were separated from SDS-PAGE, then transferred to polyvinylidene difluoride membranes. Subsequently, the membranes were blocked with 5% bovine serum albumin (BSA) at room temperature for 1 h. The bands were incubated overnight with the primary antibodies at 4 °C. After washing three times in TBST solution, the membranes were incubated with goat anti-rabbit secondary antibodies at room temperature for 1 h. Protein bands were visualized using ECL kits and an imaging system (Bio-Rad, Hercules, CA, USA).
MDC Staining assay
Cell autophagy was measured by the MDC staining assay according to the manufacturer’s instructions. Briefly, MB49 cells were treated with MDC staining solution at 37 °C for 30 min, followed by washing three times with 1× assay buffer. Autophagic vacuoles were observed under an inverted fluorescence microscope (Nikon, Tokyo, Japan).
Transmission electron microscopy (TEM) analysis
MB49 cells were collected and fixed overnight in 2.5% glutaraldehyde at 4 °C. After post-fixation with 1% osmium tetroxide, the MB49 cells were dehydrated in a graded ethanol solution and embedded in epoxy resin at 4 °C. Ultrathin sections (70 nm-thick) were double-stained with uranyl acetate and lead citrate at room temperature. Finally, autophagosomes were captured using TEM (JEOL, Tokyo, Japan).
Statistical analysis
Statistical analysis was conducted using SPSS 22.0. All experiments were repeated three times. Data were expressed as the mean ± standard deviation (SD) for normally distributed variables. Comparisons of data among the groups were performed using one-way ANOVA. p < 0.05 was considered statistically significant. Statistical graphs were generated using GraphPad Prism 8.0.
Results
HPP inhibited the proliferation of bladder cancer cells in the co-culture system
Our previous study indicated that HPP stimulated macrophage polarization toward the M1 phenotype, which inhibited the proliferation of bladder cancer cells (Jia et al. Citation2021). This study adopted a co‐culture system to better mimic the interaction between bladder cancer cells and macrophages in the TME, and investigated the effects of HPP on the proliferation of bladder cancer cells in the co-culture system. Consistent with our previous findings, HPP inhibited the viability of MB49 bladder cancer cells in the co-culture system (). EdU staining assay showed that the percentage of EDU-positive (proliferating) cells was significantly decreased after HPP treatment (). Additionally, we tested the effects of HPP on the viability of MB49 cells cultured alone. Notably, HPP had no effects on the viability of MB49 cells cultured alone (). These results demonstrated that HPP inhibited the proliferation of bladder cancer cells by regulating macrophages in the co-culture system.
Figure 1. HPP inhibited the proliferation of bladder cancer cells in the co-culture system. A CCK-8 assay. B-C EdU staining assay (magnification, ×400). The fluorescence of EdU (red) and Hoechst (blue) represent proliferating cells and cell nuclei, respectively. D CCK-8 assay. *p < 0.05; **p < 0.01; ***p < 0.001 compared with the control group.
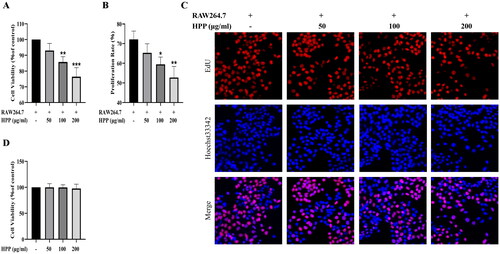
HPP inhibited the proliferation of bladder cancer cells by inducing cancer cell autophagy in the co-culture system
Next, we investigated how HPP inhibited the proliferation of MB49 cells in the co-culture system. Interestingly, HPP showed ability to increase the expression of LC3II (), a marker for autophagy. Besides, the expression of Beclin1, an autophagy-related protein, was distinctly increased, whereas the expression of p62, an autophagic substrate, was markedly decreased after HPP treatment (). Additionally, the autophagy inhibitors 3-MA and CQ were used to determine autophagic flux. 3-MA is an early inhibitor of autophagy that blocks autophagosome formation. As expected, the upregulation of LC3II/I stimulated by HPP was suppressed by 3-MA treatment (). Conversely, CQ is a late inhibitor of autophagy that inhibits autophagosome degradation. We discovered that pre-treatment with CQ resulted in the accumulation of LC3-II (). These results indicated that HPP induced autophagic flux in MB49 cells in the co-culture system.
Figure 2. HPP induced bladder cancer cell autophagy in the co-culture system. A-B Protein expression of p62, Beclin1, and LC3II/I was determined by Western blotting analysis. C-F MB49 cells were pre-treated with 5 mM 3-MA for 4 h or 50 μM CQ for 3 h, and then co-cultured with RAW264.7 cells in fresh medium containing 100 μg/mL HPP. Protein expression of LC3II/I was determined by Western blotting analysis. *p < 0.05; **p < 0.01; ***p < 0.001 compared with the control group. ##p < 0.01 compared with the HPP group.
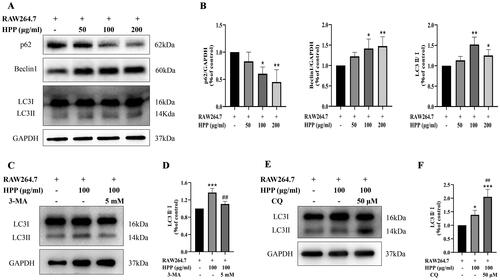
To further confirm autophagy induction, we performed MDC staining assay to visualize the level of the autophagosomes. Consistent with the Western blotting results, HPP induced autophagy and promoted the formation of autophagosomes, which was inhibited by 3-MA (). We also performed TEM analysis, which is the Gold Standard method for confirmation of autophagy. The results showed that autophagosomes with a double membrane structure increased conspicuously after HPP treatment, which was also restrained by 3-MA (). These data revealed that HPP significantly induced MB49 cell autophagy in the co-culture system.
Figure 3. HPP induced bladder cancer cell autophagy by regulating macrophages in the co-culture system, resulting in the inhibition of cancer cell proliferation. A the formation of autophagosomes in MB49 cells was observed by MDC staining (magnification, ×200) and TEM (scale bar, 2 μm). The red arrows indicate autophagosomes. B-C CCK-8 assay. D-G Protein expression of LC3II/I was determined by Western blotting analysis. *p < 0.05; **p < 0.01; ***p < 0.001 compared with the control group. #p < 0.05; ##p < 0.01 compared with the HPP group.
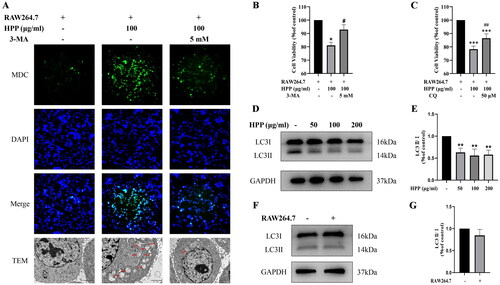
Furthermore, we explored the role of HPP-induced autophagy on MB49 cell proliferation in the co-culture system. CCK-8 assay showed that the autophagy inhibitors 3-MA and CQ attenuated HPP-induced proliferative inhibition (). Thus, it is likely that HPP inhibited the proliferation of bladder cancer cells by inducing cancer cell autophagy in the co-culture system.
HPP induced bladder cancer cell autophagy via macrophages in the co-culture system
After confirming that HPP induced bladder cancer cell autophagy in the co-culture system, we tried to elucidate the mechanisms underlying the HPP-induced autophagy. We first tested the effects of HPP on the expression of the autophagy marker protein LC3 in MB49 cells cultured alone. Interestingly, HPP treatment resulted in a reduction of LC3II in MB49 cells cultured alone, rather than increase (). This indicated that HPP did not induce autophagy in MB49 cells cultured alone. We next tested the expression of LC3 protein in both MB49 cells cultured alone and co-cultured with RAW264.7 cells. Western blotting results showed that the expression level of LC3II was not changed significantly (), suggesting that RAW264.7 alone was not sufficient to induce autophagy in MB49 cells. Combined with the results of the aforementioned experiments, it was reasonable to speculate that HPP induced bladder cancer cell autophagy by regulating macrophages in the co-culture system, resulting in the inhibition of cancer cell proliferation.
PI3K/Akt/mTOR signaling pathway was involved in HPP-induced autophagy in the co-culture system
PI3K/Akt/mTOR pathway is a critical signaling pathway involved in autophagy regulation, and its inhibition promotes autophagy (Heras-Sandoval et al. Citation2014; Polivka and Janku Citation2014). Therefore, we further explored whether or not HPP affected the proteins levels of PI3K/Akt/mTOR pathway in MB49 cells in the co-culture system. Western blotting results showed that HPP markedly suppressed the expression levels of PI3K, Akt, p-Akt, mTOR, and p-mTOR (), suggesting that HPP inhibited PI3K/Akt/mTOR signaling pathway in MB49 cells in the co-culture system. PI3K/Akt/mTOR signaling pathway was involved in HPP-induced autophagy in the co-culture system.
Discussion
Interactions between bladder cancer cells and macrophages in the TME play a pivotal role in cancer progression and treatment. In this study, a co‐culture system was designed to simulate the interactions between bladder cancer cells and macrophages in the TME. Our major findings were as follows: (1) HPP induced bladder cancer cell autophagy by regulating macrophages in the co-culture system, resulting in the inhibition of cancer cell proliferation, and (2) PI3K/Akt/mTOR pathway was involved in HPP-induced autophagy in the co-culture system
Nowadays, research on traditional Chinese medicine for cancer treatment is attracting increasing attention (Xiang et al. Citation2019). Polyporus umbellatus is used as a diuretic in clinics and its main active ingredient is PPS (Guo et al. Citation2019). Additionally, PPS has multiple biochemical and pharmacological effects, including anti-cancer, immunoregulatory, and hepatoprotection (He et al. Citation2016). Previous studies have shown that PPS can effectively inhibit the progression of bladder cancer in rats induced by N-butyl-N-(4-hydroxybutyl)-nitrosamine (BBN) (Zhang et al. Citation2011), and increase the expression of CD86 and CD40 in bladder cancer tissues (Zhang et al. Citation2015). Moreover, PPS can promote the polarization of macrophages toward an M1-like phenotype to regulate immune activity (Liu et al. Citation2017). These findings provide evidence that PPS has great potential to modulate the TME in bladder cancer.
In our previous study, we obtained HPP with strong immunomodulatory activity by purifying PPS (Liu et al. Citation2020). HPP was found to stimulated macrophage polarization toward the M1 phenotype, which inhibited the proliferation of bladder cancer cells (Jia et al. Citation2021; Liu et al. Citation2022). In this study, a co-culture system was constructed to explore the regulatory effects of HPP on MB49 bladder cancer cells and RAW264.7 macrophages in the TME. We found that HPP inhibited the proliferation of MB49 cells co-cultured with RAW264.7 cells but not MB49 cells alone. This is consistent with our previous results in which HPP inhibits the proliferation of bladder cancer cells by regulating macrophages in the co-culture system. We further identified that HPP induced autophagy and promoted the formation of autophagosomes in MB49 cells in the co-culture system. Besides, HPP inhibited the PI3K/Akt/mTOR pathway in MB49 cells in the co-culture system, which is a well-known pathway involved in the regulation of autophagy (Xue et al. Citation2017).
Autophagy, a dynamic and conserved catabolic process, plays dual roles in cancer development and therapy. Autophagy can be an adaptive mechanism for tumor survival under hypoxic, metabolic, or therapeutic stress (Marinković et al. Citation2018; Udristioiu and Nica-Badea Citation2019). On the other hand, autophagy contributes to tumor suppression. Autophagy maintains genomic integrity to prevent tumor formation by eliminating damaged structures in the early stages of cancer (Konac et al. Citation2021; Vitto et al. Citation2022). Activation of autophagy can restrain the growth of cancer cells (Zhen et al. Citation2020; Zhang et al. Citation2021; Huang et al. Citation2022), which induces autophagic cell death under certain conditions (Denton and Kumar Citation2019; Towers et al. Citation2020). Autophagy has been reported to be an important target for bladder cancer therapy. Inhibition of protective autophagy may be able to increase the sensitivity of bladder cancer to chemotherapy or radiotherapy, whereas activation of cytotoxic autophagy may facilitate cell death either alone or in association with apoptosis (Li et al. Citation2019). In this study, we found that the autophagy inhibitors 3-MA and CQ not only antagonized HPP-induced autophagy but also attenuated the inhibitory effects of HPP on MB49 cell proliferation in the co-culture system. Therefore, autophagy may be a mechanism by which HPP induces cell death in bladder cancer cells in the co-culture system.
To further elucidate the mechanisms underlying the HPP-induced autophagy, we explored factors that influence MB49 cell autophagy in the co-culture system. We evaluated the effects of HPP and RAW264.7 on LC3II expression in MB49 cells, respectively. It was surprising to find that HPP reduced LC3II expression while RAW264.7 had no effects on LC3II expression in MB49 cells cultured alone. HPP or RAW264.7 alone was not sufficient to induce autophagy in MB49 cells. Thus, HPP induced bladder cancer cell autophagy by regulating macrophages in the co-culture system. In our previous studies, we have demonstrated that HPP stimulates macrophage polarization toward the M1 phenotype and increases the secretion of pro-inflammatory cytokines, such as TNF-α, IL-6, and IL-1β (Jia et al. Citation2021; Liu et al. Citation2022). Therefore, it was reasonable to speculate that HPP induced bladder cancer cell autophagy by stimulated macrophage polarization toward the M1 phenotype in the co-culture system. However, only one bladder cancer cell line was used in the present study. Thus, follow-up studies using other cell lines are required to further validate the conclusions of this study.
The present study demonstrated that HPP induced bladder cancer cell autophagy by regulating macrophages in the co-culture system. Accumulating studies show that autophagy and immune system reciprocally affect each other, and the autophagy-mediated regulation of the immune system might strengthen or attenuate the effects of immunotherapy (Jiang et al. Citation2019; Gao et al. Citation2022). Bacillus Calmette-Guérin (BCG) is the preferred treatment for high-risk non–muscle-invasive bladder cancer (NMIBC) and an option for intermediate-risk NMIBC (Lenis et al. Citation2020). It has been reported that autophagy contributes to trained immunity induced by BCG, and the pharmacologic or genetic inhibition of autophagy blocks epigenetic reprogramming of monocytes in response to BCG training (Buffen et al. Citation2014). Programmed death ligand‐1 (PD‐L1) is the critical checkpoint protein in the immune system. Previous study has demonstrated that the expression levels of PD-L1 negatively associates with autophagic activities, and autophagy inhibition increases PD‐L1 expression leading to immune evasion in bladder cancer (Tsai et al. Citation2022). Additionally, autophagy-related genes signature has been proved to be efficient in predicting immunotherapeutic effect in bladder cancer (Cao et al. Citation2021). These findings indicate that autophagy plays a crucial role in bladder cancer immunity, which provides targets and enlightenment for bladder cancer immunotherapy. Our study demonstrates the regulatory effect of HPP on autophagy and immunity in bladder cancer. HPP shows great potential alone or in combination with the immunotherapy for treatment of bladder cancer.
Conclusions
Our results provide new evidence that HPP induces bladder cancer cell autophagy by regulating macrophages in the co-culture system, resulting in the inhibition of cancer cell proliferation. The PI3K/Akt/mTOR pathway is involved in HPP-induced autophagy in the co-culture system.
Disclosure statement
No potential conflict of interest was reported by the author(s).
Data availability statement
The data supporting the findings of this study are available from the corresponding author upon reasonable request.
Additional information
Funding
References
- Anderson NM, Simon MC. 2020. The tumor microenvironment. Curr Biol. 30(16):R921–R925. doi: 10.1016/j.cub.2020.06.081.
- Buffen K, Oosting M, Quintin J, Ng A, Kleinnijenhuis J, Kumar V, van de Vosse E, Wijmenga C, van Crevel R, Oosterwijk E, et al. 2014. Autophagy controls BCG-induced trained immunity and the response to intravesical BCG therapy for bladder cancer. PLoS Pathog. 10(10):e1004485. doi: 10.1371/journal.ppat.1004485.
- Cao R, Ma B, Wang G, Xiong Y, Tian Y, Yuan L. 2021. Identification of autophagy-related genes signature predicts chemotherapeutic and immunotherapeutic efficiency in bladder cancer (BLCA). J Cell Mol Med. 25(12):5417–5433. doi: 10.1111/jcmm.16552.
- Casey SC, Amedei A, Aquilano K, Azmi AS, Benencia F, Bhakta D, Bilsland AE, Boosani CS, Chen S, Ciriolo MR, et al. 2015. Cancer prevention and therapy through the modulation of the tumor microenvironment. Semin Cancer Biol. 35 Suppl(Suppl):S199–S223. doi: 10.1016/j.semcancer.2015.02.007.
- Crispen PL, Kusmartsev S. 2020. Mechanisms of immune evasion in bladder cancer. Cancer Immunol Immunother. 69(1):3–14. doi: 10.1007/s00262-019-02443-4.
- Denton D, Kumar S. 2019. Autophagy-dependent cell death. Cell Death Differ. 26(4):605–616. doi: 10.1038/s41418-018-0252-y.
- Gao W, Wang X, Zhou Y, Wang X, Yu Y. 2022. Autophagy, ferroptosis, pyroptosis, and necroptosis in tumor immunotherapy. Signal Transduct Target Ther. 7(1):196.
- Ge Y, Huang M, Yao YM. 2018. Autophagy and proinflammatory cytokines: interactions and clinical implications. Cytokine Growth Factor Rev. 43:38–46. doi: 10.1016/j.cytogfr.2018.07.001.
- Guo N, Bai Z, Jia W, Sun J, Wang W, Chen S, Wang H. 2019. Quantitative analysis of polysaccharide composition in Polyporus umbellatus by HPLC-ESI-TOF-MS. Molecules. 24(14):2526. doi: 10.3390/molecules24142526.
- He PF, Zhang AQ, Wang XL, Qu L, Li GL, Li YP, Sun PL. 2016. Structure elucidation and antioxidant activity of a novel polysaccharide from Polyporus umbellatus sclerotia. Int J Biol Macromol. 82:411–417. doi: 10.1016/j.ijbiomac.2015.10.032.
- Heras-Sandoval D, Pérez-Rojas JM, Hernández-Damián J, Pedraza-Chaverri J. 2014. The role of PI3K/AKT/mTOR pathway in the modulation of autophagy and the clearance of protein aggregates in neurodegeneration. Cell Signal. 26(12):2694–2701. doi: 10.1016/j.cellsig.2014.08.019.
- Hu F, Song D, Yan Y, Huang C, Shen C, Lan J, Chen Y, Liu A, Wu Q, Sun L, et al. 2021. IL-6 regulates autophagy and chemotherapy resistance by promoting BECN1 phosphorylation. Nat Commun. 12(1):3651. doi: 10.1038/s41467-021-23923-1.
- Huang Z, Gan S, Zhuang X, Chen Y, Lu L, Wang Y, Qi X, Feng Q, Huang Q, Du B, et al. 2022. Artesunate inhibits the cell growth in colorectal cancer by promoting ROS-dependent cell senescence and autophagy. Cells. 11(16):2472. doi: 10.3390/cells11162472.
- Jia W, Luo S, Lai G, Li S, Huo S, Li M, Zeng X. 2021. Homogeneous Polyporus polysaccharide inhibits bladder cancer by polarizing macrophages to M1 subtype in tumor microenvironment. BMC Complement Med Ther. 21(1):150. doi: 10.1186/s12906-021-03318-x.
- Jiang GM, Tan Y, Wang H, Peng L, Chen HT, Meng XJ, Li LL, Liu Y, Li WF, Shan H. 2019. The relationship between autophagy and the immune system and its applications for tumor immunotherapy. Mol Cancer. 18(1):17. doi: 10.1186/s12943-019-0944-z.
- Konac E, Kurman Y, Baltaci S. 2021. Contrast effects of autophagy in the treatment of bladder cancer. Exp Biol Med (Maywood). 246(3):354–367. doi: 10.1177/1535370220959336.
- Leblond MM, Zdimerova H, Desponds E, Verdeil G. 2021. Tumor-associated macrophages in bladder cancer: biological role, impact on therapeutic response and perspectives for immunotherapy. Cancers (Basel). 13(18):4712. doi: 10.3390/cancers13184712.
- Lenis AT, Lec PM, Chamie K, Mshs MD. 2020. Bladder cancer: a review. JAMA. 324(19):1980–1991. doi: 10.1001/jama.2020.17598.
- Levy JMM, Towers CG, Thorburn A. 2017. Targeting autophagy in cancer. Nat Rev Cancer. 17(9):528–542. doi: 10.1038/nrc.2017.53.
- Li F, Guo H, Yang Y, Feng M, Liu B, Ren X, Zhou H. 2019. Autophagy modulation in bladder cancer development and treatment (Review). Oncol Rep. 42(5):1647–1655. doi: 10.3892/or.2019.7286.
- Li X, He S, Ma B. 2020. Autophagy and autophagy-related proteins in cancer. Mol Cancer. 19(1):12. doi: 10.1186/s12943-020-1138-4.
- Liu CP, Zhang X, Tan QL, Xu WX, Zhou CY, Luo M, Li X, Huang RY, Zeng X. 2017. NF-κB pathways are involved in M1 polarization of RAW 264.7 macrophage by Polyporus polysaccharide in the tumor microenvironment. PLoS One. 12(11):e0188317. doi: 10.1371/journal.pone.0188317.
- Liu CP, Li X, Lai GN, Li JH, Jia WY, Cao YY, Xu WX, Tan QL, Zhou CY, Luo M, et al. 2020. Mechanisms of macrophage immunomodulatory activity induced by a new polysaccharide isolated from Polyporus umbellatus (Pers.) Fries. Front Chem. 8:581. doi: 10.3389/fchem.2020.00581.
- Liu C, He D, Zhang S, Chen H, Zhao J, Li X, Zeng X. 2022. Homogeneous Polyporus polysaccharide inhibit bladder cancer by resetting tumor-associated macrophages toward M1 through NF-κB/NLRP3 signaling. Front Immunol. 13:839460. doi: 10.3389/fimmu.2022.839460.
- Lv S, Liu H, Wang H. 2021. Exogenous hydrogen sulfide plays an important role by regulating autophagy in diabetic-related diseases. Int J Mol Sci. 22(13):6715. doi: 10.3390/ijms22136715.
- Marinković M, Šprung M, Buljubašić M, Novak I. 2018. Autophagy modulation in cancer: current knowledge on action and therapy. Oxid Med Cell Longev. 2018:8023821–8023818. doi: 10.1155/2018/8023821.
- Mizushima N, Komatsu M. 2011. Autophagy: renovation of cells and tissues. Cell. 147(4):728–741. doi: 10.1016/j.cell.2011.10.026.
- Nielsen SR, Schmid MC. 2017. Macrophages as key drivers of cancer progression and metastasis. Mediators Inflamm. 2017:9624760–9624711. doi: 10.1155/2017/9624760.
- Parzych KR, Klionsky DJ. 2014. An overview of autophagy: morphology, mechanism, and regulation. Antioxid Redox Signal. 20(3):460–473. doi: 10.1089/ars.2013.5371.
- Polivka J, Jr, Janku F. 2014. Molecular targets for cancer therapy in the PI3K/AKT/mTOR pathway. Pharmacol Ther. 142(2):164–175. doi: 10.1016/j.pharmthera.2013.12.004.
- Roma-Rodrigues C, Mendes R, Baptista PV, Fernandes AR. 2019. Targeting tumor microenvironment for cancer therapy. Int J Mol Sci. 20(4):840. doi: 10.3390/ijms20040840.
- Shen J, Xu S, Zhou H, Liu H, Jiang W, Hao J, Hu Z. 2017. IL-1β induces apoptosis and autophagy via mitochondria pathway in human degenerative nucleus pulposus cells. Sci Rep. 7(1):41067. doi: 10.1038/srep41067.
- Sun H, Wang Z, Yakisich JS. 2013. Natural products targeting autophagy via the PI3K/Akt/mTOR pathway as anticancer agents. Anticancer Agents Med Chem. 13(7):1048–1056. doi: 10.2174/18715206113139990130.
- Towers CG, Wodetzki D, Thorburn A. 2020. Autophagy and cancer: modulation of cell death pathways and cancer cell adaptations. J Cell Biol. 219(1):e201909033. doi: 10.1083/jcb.201909033.
- Tsai TF, Chang AC, Chen PC, Ho CY, Chen HE, Chou KY, Hwang TI. 2022. Autophagy blockade potentiates cancer-associated immunosuppression through programmed death ligand-1 upregulation in bladder cancer. J Cell Physiol. 237(9):3587–3597. doi: 10.1002/jcp.30817.
- Udristioiu A, Nica-Badea D. 2019. Autophagy dysfunctions associated with cancer cells and their therapeutic implications. Biomed Pharmacother. 115:108892. doi: 10.1016/j.biopha.2019.108892.
- van Wilpe S, Gerretsen ECF, van der Heijden AG, de Vries IJM, Gerritsen WR, Mehra N. 2020. Prognostic and predictive value of tumor-infiltrating immune cells in urothelial cancer of the bladder. Cancers (Basel). 12(9):2692. doi: 10.3390/cancers12092692.
- Vitto VAM, Bianchin S, Zolondick AA, Pellielo G, Rimessi A, Chianese D, Yang H, Carbone M, Pinton P, Giorgi C, et al. 2022. Molecular mechanisms of autophagy in cancer development, progression, and therapy. Biomedicines. 10(7):1596. doi: 10.3390/biomedicines10071596.
- Xiang Y, Guo Z, Zhu P, Chen J, Huang Y. 2019. Traditional Chinese medicine as a cancer treatment: modern perspectives of ancient but advanced science. Cancer Med. 8(5):1958–1975. doi: 10.1002/cam4.2108.
- Xiao Y, Yu D. 2021. Tumor microenvironment as a therapeutic target in cancer. Pharmacol Ther. 221:107753. doi: 10.1016/j.pharmthera.2020.107753.
- Xue JF, Shi ZM, Zou J, Li XL. 2017. Inhibition of PI3K/AKT/mTOR signaling pathway promotes autophagy of articular chondrocytes and attenuates inflammatory response in rats with osteoarthritis. Biomed Pharmacother. 89:1252–1261. doi: 10.1016/j.biopha.2017.01.130.
- Yuan Y, Ding D, Zhang N, Xia Z, Wang J, Yang H, Guo F, Li B. 2018. TNF-α induces autophagy through ERK1/2 pathway to regulate apoptosis in neonatal necrotizing enterocolitis model cells IEC-6. Cell Cycle. 17(11):1390–1402. doi: 10.1080/15384101.2018.1482150.
- Zhang G, Zeng X, Li C, Li J, Huang Y, Han L, Wei JA, Huang H. 2011. Inhibition of urinary bladder carcinogenesis by aqueous extract of sclerotia of Polyporus umbellatus Fries and Polyporus polysaccharide. Am J Chin Med. 39(1):135–144. doi: 10.1142/S0192415X11008701.
- Zhang GW, Qin GF, Han B, Li CX, Yang HG, Nie PH, Zeng X. 2015. Efficacy of Zhuling Polyporus polysaccharide with BCG to inhibit bladder carcinoma. Carbohydr Polym. 118:30–35. doi: 10.1016/j.carbpol.2014.11.012.
- Zhang Y, He N, Zhou X, Wang F, Cai H, Huang SH, Chen X, Hu Z, Jin X. 2021. Betulinic acid induces autophagy-dependent apoptosis via Bmi-1/ROS/AMPK-mTOR-ULK1 axis in human bladder cancer cells. Aging (Albany NY). 13(17):21251–21267. doi: 10.18632/aging.203441.
- Zhen Y, Zhao R, Wang M, Jiang X, Gao F, Fu L, Zhang L, Zhou XL. 2020. Flubendazole elicits anti-cancer effects via targeting EVA1A-modulated autophagy and apoptosis in Triple-negative Breast Cancer. Theranostics. 10(18):8080–8097. doi: 10.7150/thno.43473.