ABSTRACT
Introduction
Studies have compared chimeric antigen receptor (CAR) T-cell therapies and salvage chemotherapy in relapsed/refractory large B-cell lymphoma (LBCL) patients, but further evidence of their relative effectiveness is warranted.
Methods
Our systematic review identified studies comparing efficacy and safety outcomes of axicabtagene ciloleucel (axi-cel), lisocabtagene maraleucel (liso-cel) and tisagenlecleucel (tisa-cel) trials to salvage chemotherapy cohorts in LBCL patients with ≥2 prior lines of treatment; and an extended evidence network included indirect comparisons comparing CAR T-cell therapies. We conducted network meta-analyzes using Bayesian hierarchical modeling.
Results
Three studies comparing ZUMA-1 (axi-cel), TRANSCEND (liso-cel) and JULIET (tisa-cel) trials to salvage chemotherapy within the SCHOLAR-1 cohort were identified. Axi-cel (odds ratio [OR]:5.63; 95% credible interval [CrI]:2.66–12.42) and liso-cel (OR:4.26; 95%CrI:2.33–7.93) showed a significant increased overall response rate compared to tisa-cel, but not to one-another. Axi-cel demonstrated significant improvements in overall survival relative to liso-cel (hazard ratio [HR]:0.54; 95%CrI:0.37–0.79) and tisa-cel (HR:0.47; 95%CrI:0.26–0.88). Higher rates of grade ≥3 neurological events were observed with axi-cel than with tisa-cel and liso-cel.
Conclusions
We highlight important differences in clinical outcomes between CAR T-cell therapies. Axi-cel demonstrated improved overall survival compared to tisa-cel and liso-cel, and both axi-cel and liso-cel showed higher response rates compared to tisa-cel.
1. Introduction
The introduction of anti-CD19 chimeric antigen receptor (CAR) T-cell therapies has transformed the treatment landscape of relapsed/refractory (R/R) large B-cell lymphoma (LBCL) in recent years. Three CAR T-cell therapies – axicabtagene ciloleucel (axi-cel), tisagenlecleucel (tisa-cel), and most recently lisocabtagene maraleucel (liso-cel) – may offer curative potential among patients who have failed curative treatment options in the first- and second-line of therapy (LoT). Although these treatments are all of the same class (CAR T-cell therapies), they differ with respect to their co-stimulatory domains (anti-CD19 vs 4-1BB) and differ in a variety of ways with respect to mechanisms of action [Citation1,Citation2]. It is unclear how these differences can translate with respect to efficacy and safety.
Initial regulatory approval of these treatments was based on results of three pivotal single-arm, Phase II trials: ZUMA-1 for axi-cel [Citation3,Citation4], TRANSCEND for liso-cel [Citation5], and JULIET for tisa-cel [Citation6]. And the promising results from these trials have been confirmed by a growing number of real-world CAR T-cell therapy studies [Citation7–9]. Recent real-world data also suggest important efficacy and safety differences may exist between these transformative agents, warranting further investigation of their relative treatment effects [Citation10–12].
Given the non-comparative nature of the CAR T-cell therapy clinical studies, indirect treatment comparisons (ITCs) are of interest. There are multiple methods that attempt to emulate randomized clinical trials (RCTs) by optimizing internal validity. When individual patient data (IPD) are available for both treatments, propensity score analyzes can be used to optimize the internal validity of a comparative analysis. However, when IPD is only available for one treatment, with aggregate data available for the other treatment, matching-adjusted indirect comparisons (MAICs) are an effective approach to reduce confounding bias [Citation13]. MAICs have been conducted to compare the CAR T-cell therapies to one another with respect to efficacy and safety using results from the pivotal trials [Citation14,Citation15]. As these pivotal trials were single-arm, all MAICs that have been conducted to compare the trial results are unanchored, meaning the two treatments are compared in the absence of a common comparator, such as salvage chemotherapy. For unanchored MAICs, the primary assumption is that there are no unobserved prognostic factors, while the primary assumption for propensity score analyzes is no unobserved confounders (a subset of prognostic factors). For this reason, anchored MAICs should be used, when possible, to provide a more robust comparison [Citation13,Citation16].
In addition to comparing CAR T-cell therapies to one another, ITC methods have been used to compare them to salvage chemotherapy, which was the historical standard of care (SoC) prior to the introduction of CAR T-cell therapies [Citation17]. For example, an external control cohort, SCHOLAR-1, was developed using two real-world cohorts and the extended follow-up of two trials to act as a historical SoC for comparison with outcomes from ZUMA-1 (axi-cel) [Citation3,Citation18]. A propensity score analysis has been used to compare axi-cel with SCHOLAR-1, which consists of R/R LBCL patients eligible for CAR T-cell therapy [Citation19]. The existence of such studies allows the construction of an anchored network for indirect treatment comparisons between the trial outcomes from CAR T-cell therapies.
Evaluating comparative effectiveness and safety of CAR T-cell therapies is important for clinical decisions and policymaking. Given the dearth of direct comparative evidence, our aim was to identify all comparative studies of CAR T-cell therapies and salvage chemotherapy through a systematic literature review, and, where feasible, to provide a more robust evaluation of comparative efficacy and safety using an anchored network meta-analysis (NMA).
2. Methods
2.1. Systematic literature review
We systematically searched Embase and Medline via Ovid on 16 January 2023, for evidence from inception (i.e. from 1946 to 2023). Searches of medical literature databases were supplemented by hand searches of conferences in years determined to not be indexed in Embase. Study selection was conducted using set PICOS (population, intervention, comparator, outcomes, and study design) criteria [Citation20]. Eligible studies included adults with R/R LBCL with ≥2 prior LoTs. Eligible interventions were CAR T-cell therapeutics commercially available to the target population: axi-cel, liso-cel and tisa-cel. Studies were required to be comparative, with eligible comparator for the primary evidence base being salvage chemotherapy or another form of historical SoC. Study selection was also extended for a secondary evidence base, which allowed for other CAR T-cell therapeutics among the eligible interventions as comparators. As our interest was in evaluating the trial evidence supporting CAR T-cell therapies in this population, real-world CAR T-cell studies were excluded. Furthermore, eligible studies were required to use methods designed to emulate RCTs, including propensity score analyses and population-adjusted indirect comparisons (PAIC) such as MAICs (see Supplementary Materials). Eligible studies were required to report on at least one outcome of interest, which included overall survival (OS), progression-free survival (PFS), objective response rate (ORR), complete response (CR), cytokine release syndrome (CRS) and neurological events (NE). The search terms used for database, full PICOS criteria, and conference searches are presented in Tables S1–3.
All study selection and data extraction steps were conducted by two reviewers working independently and in duplicate in accordance with PRISMA guidelines [Citation21]. In situations where consensus could not be reached between the two reviewers, a third reviewer provided arbitration. Following the identification of eligible publications, these were mapped to their underlying studies. Study characteristics, patient characteristics, and outcomes were extracted into standardized data extraction templates. Patient characteristics included both those observed and adjusted (e.g. by propensity score methods). Quality and risk of bias assessments were conducted using the Newcastle-Ottawa Scale (NOS) for cohort studies [Citation22]. The instrument evaluates the quality of observational studies based on three domains: study group selection; study group comparability; and outcome ascertainment.
2.2. Statistical analyses
Prior to statistical analysis, the evidence base was assessed to evaluate what analyses were feasible. The feasibility assessment consisted of determining the reporting of each outcome within the network of evidence, evaluating the methods and adjustments used in each study within the network, comparing the post-weighting patient characteristics and reviewing the resulting effective sample sizes and/or sum of weights. Outcomes were included in the analyzes if they were reported in two or more eligible studies.
For sufficiently reported outcomes, we conducted network meta-analyzes (NMAs) using Bayesian hierarchical models [Citation23,Citation24]. Bayesian models use priors, likelihoods, and models to obtain posterior estimates based on the observed data. In these analyses, the priors were non-informative, and results of these Bayesian analyses are similar to their frequentist NMA counterparts. Advantages to the Bayesian approach are that they use likelihoods that better reflect the underlying data (i.e. they avoid Normal approximations) and lend themselves better to more complicated models (not needed here). For time-to-event outcomes, the log hazard ratios from the included studies were modeled using a mean difference NMA. In these analyses, the log hazard ratios were treated as continuous outcomes from the studies using a normal likelihood with identity link function, the standard approach to modeling survival data meeting the proportional hazards condition. NMAs for dichotomous outcomes consisted of a logistic regression model with binomial likelihood. In all cases, non-informative normal priors were used. By using non-informative priors, the credible intervals presented in this study are equivalent to confidence intervals. All analyses used the adjusted estimates from the propensity score analyses or the MAICs. When multiple analyses were presented within the same study, the results of the primary analysis as identified by the study authors were selected.
The primary analysis consisted of the studies which reported CAR T-cell therapy vs. salvage chemotherapy comparisons, which allowed the construction of an anchored network with salvage chemotherapy as the common comparator. This complements the previously published CAR T-cell to CAR T-cell therapy comparisons, while providing an opportunity to compare results. As a secondary analysis, this network was expanded to include the studies that reported comparisons between CAR T-cell therapy (e.g. axi-cel vs tisa-cel, without a common comparator). In this larger network, all available evidence was included, and the network still benefitted from the addition of the common comparator. Fixed and random-effects models were applied, dependent upon how many studies were included in each model. Model selection was conducted using the deviance information criterion in accordance with set conventions [Citation25,Citation26]. As there were closed loops in the extended network, inconsistency was assessed using node splitting. All analyses were performed using R version 4.1.0 and using JAGS version 4.3. Bayesian analyses used a burn-in of 10,000 iterations with a further 25,000 iterations. Convergence was assessed using density plots and Gelman plots.
2.3. Ethical approval
No ethical approval was required as data in this study are from previously published studies in which informed consent was obtained by primary investigators.
3. Results
3.1. Evidence base
From the 467 publications identified through database and conference searches, a total of 3 studies [Citation19,Citation27,Citation28] were included in the primary evidence base (i.e. studies with salvage chemotherapy as a comparator), with an additional 5 studies [Citation29–33] included in the extended network (). The eight total studies were reported in 18 publications (Supplemental Table S4). From the feasibility assessment, OS, ORR, and CR were sufficiently well-reported to be included in the primary analysis, while neither PFS or safety outcomes could be analyzed. Quality assessment revealed studies were of generally high-quality (Table S5 of the Supplemental Materials).
presents the networks of evidence. Compared to most networks of evidence, there was an important overlap in the included salvage chemotherapy common comparator cohorts. Two studies included the SCHOLAR-1 external control cohort. The third study, salvage chemotherapy SoC to data from JULIET [Citation27], used the CORAL study cohort – a subset of SCHOLAR-1 consisting of follow-up data from a second-line clinical trial [Citation34,Citation35]. For the primary network, the comparisons between ZUMA-1 and SCHOLAR-1 [Citation19], and JULIET and CORAL [Citation27] used propensity score methods. The comparison between TRANSCEND and SCHOLAR-1 used a MAIC [Citation28]. Propensity score methods are better suited than MAICs to reduce bias. The extended network included an additional five studies. All five were unanchored MAICs comparing two CAR T-cell therapies: two comparisons between ZUMA-1 and TRANSCEND [Citation30,Citation32], two comparisons between TRANSCEND to JULIET [Citation29,Citation33], and one study comparing JULIET to ZUMA-1 [Citation31].
Figure 2. Network of evidence for (a) the primary evidence base; and (b) the extended evidence base.
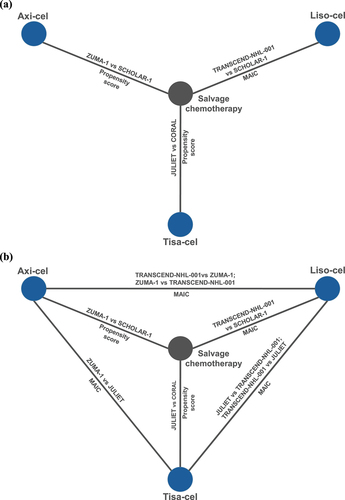
In addition to the methods used by each study, important considerations are the variables used to make these adjustments and the resulting sample sizes. These are reported in . For the primary network, age and prior stem cell transplant (SCT) were the only covariates that were adjusted for across all three studies. Many variables were adjusted for in one or more of the primary studies, including non-Hodgkin’s lymphoma (NHL) subtype, refractory to last LoT and either International Prognostic Index (IPI), or factors that contribute to IPI. Post-adjustment sample sizes and effective sample sizes (ESS) ranged from 80 to 248 patients among the CAR T-cell cohorts, while the sum of weights and ESS for the salvage chemotherapy cohorts ranged from 145 to 636 patients. Further details for the extended network are provided in Supplementary Table S6.
Table 1. Adjustment methods and details in the primary network.
3.2. Comparative analyses
Results of the NMAs for the primary analysis are presented in . As all hazard ratios (HRs) for OS were based on analyses that met the proportional hazards assumption, analyses were conducted using the HRs. Across all outcomes, CAR T-cell therapies performed significantly better than salvage chemotherapy. For OS, the HRs of 0.27 (95% credible interval [CrI]: 0.19–0.38), 0.50 (95% CrI: 0.40–0.60) and 0.57 (95% CrI: 0.44–0.73) favored axi-cel, liso-cel and tisa-cel, respectively, when compared to salvage chemotherapy. Similarly, response outcomes were in favor of CAR T-cell therapies in comparison to salvage chemotherapy, although CR was not reported in the tisa-cel study.
Table 2. Network meta-analysis results using the primary network.
When comparing CAR T-cell therapies using the anchored network, axi-cel was associated with a significant improvement in OS compared to liso-cel (HR: 0.54; 95% CrI: 0.37–0.79) and tisa-cel (HR: 0.47; 95% CrI: 0.26–0.88). The comparison of OS between liso-cel and tisa-cel was not statistically differentiable (HR: 0.87; 95% CrI: 0.42–1.78). For ORR, axi-cel was associated with a higher response rate compared to tisa-cel (OR: 5.62; 95% CrI: 2.64–12.42), but not liso-cel (OR: 1.32; 95% CrI: 0.64–2.87), and liso-cel was associated with a higher response rate compared to tisa-cel (OR: 4.24; 95% CrI: 2.28–7.91). For CR, only a comparison between axi-cel and liso-cel was possible, and no significant difference was observed (OR: 0.67; 95% CrI: 0.32–1.37) between these treatments.
All analyses were repeated in the expanded network (Supplementary Table S8). Despite the inclusion of more comparisons, the pattern of results and significance of the treatment comparisons did not change. For OS, the estimates of the comparisons remained almost unchanged, whilst effect sizes were generally increased for response outcomes in the extended network. Due to increased data availability, the extended network allowed for comparisons to tisa-cel to be made for CR. Axi-cel was associated with a higher CR compared to tisa-cel (OR: 3.23; 95% CrI: 1.96–5.26), but not liso-cel (OR: 1.01; 95% CrI: 0.88–2.00), and liso-cel was associated with a higher response rate compared to tisa-cel (OR: 3.23; 95% CrI: 2.04–5.00).
The extended network also provided an opportunity to analyze safety outcomes (Supplementary Table S9). There was an increased risk of CRS and NE with axi-cel relative to both liso-cel and tisa-cel. The odds ratio of grade ≥3 CRS with axi-cel relative to liso-cel was 4.63 (95% CrI: 2.01–11.48) and relative to tisa-cel was 0.47 (95% CrI: 0.17–1.13). The odds ratio of grade ≥3 NE with axi-cel relative to liso-cel was 4.53 (95% CrI: 2.68–7.69) and relative to tisa-cel was 2.85 (95% CrI: 1.47–5.45). Liso-cel and tisa-cel were not statistically different with respect to NEs, but liso-cel led to reduced odds of CRS relative to tisa-cel.
To place these results in context, presents the results from our primary analysis and the results from published unanchored CAR T-cell to CAR T-cell ITC studies, which also contributed to our extended network of evidence. For all other outcomes and comparisons, the direction and significance of the anchored network aligned well with the published ITCs, and this despite the comparisons being done using completely different (network) pathways. In most cases, the magnitude of the effect was also similar. For example, with respect to OS in the comparison of axi-cel to liso-cel, our study results aligned very well with those of a prior MAIC [Citation32]. However, there was a more notable difference in the estimated OR of liso-cel to tisa-cel for ORR, which shifted from 2.78 to 4.24.
Table 3. Comparison of the three CAR T-cell treatments – NMAs vs published ITC results.
4. Discussion
This study compared the efficacy of axi-cel, liso-cel, and tisa-cel through a common comparator. Our findings suggest that important differences exist between several clinical outcomes achieved by approved CAR T-cell therapies in treating 3 L+ R/R LBCL [Citation14]. In these analyses, axi-cel demonstrated significant improvements in OS compared to both tisa-cel and liso-cel, while no difference in OS was observed between tisa-cel and liso-cel. Axi-cel and liso-cel both significantly improved ORR when compared to tisa-cel; however, we did not observe a statistically significant difference in ORR or CR between them. These results help confirm those observed in previously published studies and add to the growing body of comparative evidence between CAR T-cell therapies.
For oncology trials and studies, OS is the gold-standard outcome due to both its objectivity (i.e. death can be measured without prejudice) and its clinical meaningfulness [Citation38]. All three commercially available CAR T-cell products showed survival benefits over salvage chemotherapy [Citation19,Citation27,Citation28]. Axi-cel also showed reduced mortality compared to both liso-cel and tisa-cel. This significant difference between axi-cel and liso-cel was found in a previous MAIC [Citation32]. Of note, relative to TRANSCEND-NHL-001, ZUMA-1 patients tended to have more prior lines of therapy, fewer patients with complete response as the best response to last treatment, more patients with higher tumor burden and more patients with elevated LDH [Citation3,Citation5]. While a naïve comparison between trials would not lead to a significant difference in overall survival, re-weighting on these variables does. The difference in overall survival between axi-cel and tisa-cel is also well-understood. The recent DESCAR-T registry real-world study provided robust, high-quality evidence of the comparative efficacy of axi-cel and tisa-cel using propensity score modeling [Citation10]. Here too, evidence supported improved overall survival and progression-free survival using axi-cel, which is further supported by a growing number of real-world studies adding to this evidence base [Citation11,Citation12,Citation39]. A similar study will be needed to draw inference on the comparison of liso-cel to both axi-cel and tisa-cel, but as this real-world evidence is not yet available, this study provides further insights into the comparison.
Like OS, all three CAR T-cell therapies were superior to salvage chemotherapy for ORR, and axi-cel and liso-cel both showed higher ORR compared to tisa-cel. Unlike for OS, axi-cel and liso-cel response rates were not statistically different (both ORR and CR). However, both treatments had high response rates, making differences more difficult to identify statistically. Moreover, prior studies have suggested a longer duration of response with axi-cel relative to liso-cel [Citation32], which could help explain why OS is differentiable and response is not.
In sensitivity analyzes, safety was investigated in the absence of salvage chemotherapy. For grade ≥3 CRS, liso-cel showed lower rates than both axi-cel and tisa-cel while the latter two were not statistically differentiable from one another. For grade ≥3 NE, liso-cel showed lower rates than axi-cel while the other comparisons were not statistically differentiable. Importantly, these data are from early clinical trial data, and real-world evidence suggests that safety outcomes have improved for CAR-T since this time [Citation10]. Specifically, the rates of grade ≥3 CRS and NE were both more than halved in the DESCAR-T registry relative to ZUMA-1 and JULIET, suggesting that safety outcomes have greatly changed since these trials were conducted.
The survival differences observed between CAR T-cell products in this setting may have multiple etiologies. One factor could be the construct differences between the agents. Another emerging factor could be variations across products in the time elapsed between apheresis and infusion (vein-to-vein time) [Citation40]. Recent studies of axi-cel and tisa-cel have suggested that an increased vein-to-vein time could result in less favorable clinical outcomes [Citation41,Citation42]. The median vein-to-vein times in ZUMA-1, TRANSCEND, and JULIET were 23 days, 37 days, and 54 days, respectively [Citation6,Citation36,Citation40]; however, further research is needed to establish the extent to which this factor could have played a role. There were some differences in the covariates that were used in the models comparing each CAR T-cell therapy to salvage chemotherapy, although the differences were unlikely to be drivers of the estimated survival difference. One covariate that was not addressed in the primary analyses was the use of bridging therapy because there was no need for bridging in the salvage chemotherapy arm. The difference due to bridging cannot be controlled for; however, a scenario analysis including patients from a ZUMA-1 cohort that allowed bridging did not affect the MAIC results [Citation32]. In addition, there were no indications in any of the baseline patient characteristics that suggested that ZUMA-1 patients were healthier than in other trials. Therefore, it is unlikely that selection bias explains the treatment effect we observed.
The results from the expanded network NMA aligned with the primary analyses. For the axi-cel to liso-cel comparison, results for the MAIC by Oluwole et al. suggested improved OS with axi-cel relative to liso-cel and our comparison through SCHOLAR-1 corroborate this finding [Citation32]. Results by Maloney et al. did not find a statistically significant difference between the two treatments, but most of their estimates were also in favor of axi-cel [Citation30]. The inclusion of both estimates in the secondary analysis did not deter from the NMA results aligning with those of Oluwole et al. Overall, there tended to be strong alignment between these NMA results and those of the previously published MAICs, thus providing a form of scientific replication.
Our study has several strengths and limitations. Among its strengths are the ability to inform the salvage chemotherapy node within the network of evidence with a common study and treatment (namely salvage chemotherapy SCHOLAR-1 and its sub-cohorts) [Citation18] and to compare the results against other indirect comparisons that have been conducted previously. The most important limitation is the study design of the studies included in the NMA. In general, evidence bases used to conduct NMAs are composed of RCTs in order to capitalize on their internal validity [Citation25]. By contrast, this evidence base uses studies that emulate RCTs. While these reduce bias, they may not eliminate it completely. Nonetheless, three points should be kept in mind. First, the SoC common comparator used the same patient cohort, thus reducing the within node heterogeneity. Second, there was strong alignment between the results of this study and those of previously published MAICs real-world comparative data, as discussed previously. For example, the HR for OS in real-world studies range between 0.41 and 0.69 [Citation11,Citation12], and our 0.54 HR estimate falls within that range. Third, indirect treatment comparisons lead to larger uncertainty in treatment effect estimates, so conducting NMA on propensity score and MAIC analyses will naturally lead to estimates with larger confidence intervals than what we could expect among randomized trials. Another limitation is that the adjustments for patient characteristic imbalances may not have included unobserved confounders or, in some cases, could not include known confounders. For example, the study by Maziarz et al. acknowledges that elevated lactate dehydrogenase is a prognostic factor, but it could not be included in the model. This concern is highlighted by the heterogeneity in the choice of variables used to derive the model weights. While our study adds further context to how these CAR T-cell therapies compare to one another, it provides limited insights into the challenges of determining when these treatments should be given, as discussed by others [Citation37]. Nonetheless, the recent success of ZUMA-7 and TRANSFORM do suggest that second-line use is appropriate [Citation1,Citation2]. Finally, our study was limited to only select efficacy outcomes due to the limited outcomes reported in the included studies. Importantly, none of the three included studies reported on safety outcomes. Fortunately, they were feasible in the secondary analyses.
5. Conclusion
Comparative effectiveness analyzes are critical to facilitate efficient and clinically appropriate decision-making in the treatment of R/R LBCL. CAR T-cell therapies have addressed a substantial unmet need for R/R LBCL patients who previously experienced poor outcomes from salvage chemotherapy. Among these, axi-cel has been associated with improved survival relative to tisa-cel and liso-cel and these findings are consistent both with other published ITCs and a growing body of real-world evidence.
Declaration of interest
F.L. Locke has received research support from Kite Pharma (Institutional), Allogene (Institutional), CERo Therapeutics (Institutional), Novartis (Institutional), BlueBird Bio (Institutional), BMS (Institutional), National Cancer Institute, Leukemia and Lymphoma Society; has served as Advisory Board Member/Consultant for A2, Allogene, Amgen, Bluebird Bio, BMS/Celgene, Calibr, Caribou, Cellular Biomedicine Group, Cowen, Daiichi Sankyo, EcoR1, Emerging Therapy Solutions, GammaDelta Therapeutics, Gerson Lehrman Group (GLG), Iovance, Kite Pharma, Janssen, Legend Biotech, Novartis, Sana, Takeda, Wugen, Umoja, Pfizer, Aptitude Health, ASH, BioPharma Communications CARE Education, Clinical Care Options Oncology, Imedex, Society for Immunotherapy of Cancer; and has intellectual property related to cell therapy. S.S. Neelapu has received research support from Kite/Gilead, BMS, Allogene, Precision Biosciences, and Adicet Bio; served as Advisory Board Member/Consultant for Kite/Gilead, Merck, Sellas Life Sciences, Athenex, Allogene, Incyte, Adicet Bio, BMS, Bluebird Bio, Fosun Kite, Sana Biotechnology, Caribou, Astellas Pharma, Morphosys, Janssen, Chimagen, ImmunoACT, Orna Therapeutics, Takeda, and Synthekine; has stock options from Longbow Immunotherapy, Inc; and has intellectual property related to cell therapy. M.D. Rey and A.R. Patel are currently employed at and hold individual stocks and stock options for Kite, a Gilead Company. S. Kanters and E. Limbrick-Oildfield are employed by RainCity Analytics. S.W. Wade has provided consulting services to Kite, AbbVie, and Johnson & Johnson. O.O. Oluwole: reports consultancy and advisory board for: Pfizer, Kite, Gilead, AbbVie, Janssen, TGR, ADC, Novartis, Epizyme, Nektar, Cargo, Caribou. Institution funding: Kite, Pfizer, Daichi Sankyo, Allogene. Honoraria: Pfizer, Gilead. The authors have no other relevant affiliations or financial involvement with any organization or entity with a financial interest in or financial conflict with the subject matter or materials discussed in the manuscript apart from those disclosed.
Reviewer disclosures
Peer reviewers on this manuscript have no relevant financial or other relationships to disclose.
Author contributions
OO Oluwole, SS Neelapu, MD Ray, EH Limbrick-Oldfield, SW Wade, S Kanters, AR Patel, FL Locke were all responsible for study conception and design, drafting of the original manuscript and critical revision of the manuscript. EH Limbrick-Oldfield and S Kanters were responsible for acquisition, analysis, and interpretation of data. MD Ray, SW Wade and AR Patel provided administrative, technical, and material support. FL Locke, SS Neelapu and OO Oluwole provided study supervision. All authors had full access to all of the data in the study. All authors take responsibility for the integrity of the data, the accuracy of the data analysis, and the final decision to submit for publication.
Supplemental Material
Download PDF (881.3 KB)Acknowledgments
Alana Stilla (Research Assistant, RainCity Analytics) and Leah Yang (Research Assistant, RainCity Analytics) assisted with some of the systematic literature review and manuscript preparation steps.
Supplementary material
Supplemental data for this article can be accessed online at https://doi.org/10.1080/14737140.2024.2343801.
Additional information
Funding
References
- Kamdar M, Solomon SR, Arnason J, et al. Lisocabtagene maraleucel versus standard of care with salvage chemotherapy followed by autologous stem cell transplantation as second-line treatment in patients with relapsed or refractory large B-cell lymphoma (TRANSFORM): results from an interim analysis of an open-label, randomised, phase 3 trial. Lancet. 2022 Jun 18;399(10343):2294–2308. doi: 10.1016/S0140-6736(22)00662-6
- Westin JR, Oluwole OO, Kersten MJ, et al. Survival with axicabtagene ciloleucel in large B-cell lymphoma. N Engl J Med. 2023 Jul 13;389(2):148–157. doi: 10.1056/NEJMoa2301665
- Neelapu SS, Locke FL, Bartlett NL, et al. Axicabtagene ciloleucel CAR T-Cell therapy in refractory large B-cell lymphoma. N Engl J Med. 2017 Dec 28;377(26):2531–2544. doi: 10.1056/NEJMoa1707447
- Neelapu SS, Jacobson CA, Ghobadi A, et al. 5-year follow-up supports curative potential of axicabtagene ciloleucel in refractory large B-cell lymphoma (zuma-1). Blood. 2023 Feb 23. doi: 10.1182/blood.2022018893
- Abramson JS, Palomba ML, Gordon LI, et al. Lisocabtagene maraleucel for patients with relapsed or refractory large B-cell lymphomas (TRANSCEND NHL 001): a multicentre seamless design study [Clinical Trial;Multicenter Study;Research Support, Non-U.S. Gov’t]. Lancet. 2020 Sep 19;396(10254):839–852. doi: 10.1016/S0140-6736(20)31366-0
- Schuster SJ, Bishop MR, Tam CS, et al. Tisagenlecleucel in adult relapsed or refractory diffuse large B-cell lymphoma. N Engl J Med. 2019 Jan 03;380(1):45–56. doi: 10.1056/NEJMoa1804980
- Jacobson CA, Locke FL, Ma L, et al. Real-world evidence of axicabtagene ciloleucel for the treatment of large B-Cell lymphoma in the United States. Transplant Cell Ther. 2022 Sep;28(9):581.e1–581.
- Nastoupil LJ, Jain MD, Feng L, et al. Standard-of-care axicabtagene ciloleucel for relapsed or refractory large B-cell lymphoma: results from the US lymphoma CAR T consortium. J Clin Oncol. 2020 20 Sep;38(27):3119–3128. doi: 10.1200/JCO.19.02104
- Jacobson CA, Locke FL, Ma L, et al. Effectiveness and safety of axicabtagene-ciloleucel and tisagenlecleucel for large B-cell lymphoma in the real-world setting: a systematic review and meta-analysis. 5th European CAR T-cell Meeting; 2023; Rotterdam NL.
- Bachy E, Le Gouill S, Di Blasi R, et al. A real-world comparison of tisagenlecleucel and axicabtagene ciloleucel CAR T cells in relapsed or refractory diffuse large B cell lymphoma. Nat Med. 2022 Sep 22;28(10):2145–2154. doi: 10.1038/s41591-022-01969-y
- Kwon M, Iacoboni G, Reguera JL, et al. Axicabtagene ciloleucel compared to tisagenlecleucel for the treatment of aggressive B-cell lymphoma. Haematologica. 2023 Jan 1;108(1):110–121.
- Riedell PA, Hwang WT, Nastoupil LJ, et al. Patterns of use, outcomes, and resource utilization among recipients of commercial axicabtagene ciloleucel and tisagenlecleucel for relapsed/refractory aggressive B cell lymphomas. Transplant Cell Ther. 2022 Jul 16;28(10):669–676. doi: 10.1016/j.jtct.2022.07.011
- Phillippo DM, Ades AE, Dias S, et al. Methods for population-adjusted indirect comparisons in health technology appraisal. Med Decis Mak. 2018;38(2):200–211. doi: 10.1177/0272989X17725740
- Maloney DG, Kuruvilla J, Liu FF, et al. Matching-adjusted indirect treatment comparison of liso-cel versus axi-cel in relapsed or refractory large B cell lymphoma. J Hematol Oncol. 2021 Dec;14(1). doi: 10.1186/s13045-021-01144-9
- Oluwole OO, Chen JM, Chan K, et al. ABCL-289: matching-adjusted indirect comparison (maic) of axicabtagene ciloleucel (axi-cel) and lisocabtagene maraleucel (liso-cel) in relapsed or refractory (r/r) large b-cell lymphoma (lbcl) after two or more prior lines of therapy [conference abstract]. Clin Lymphoma Myeloma Leuk. 2021 Sep;21(Supplement 1):S388.
- Gartlehner G, Moore CG. Direct versus indirect comparisons: a summary of the evidence. Int J Technol Assess Health Care. 2008;24(2):170–177. doi: 10.1017/S0266462308080240
- Banerjee R, Midha S, Kelkar AH, et al. Synthetic control arms in studies of multiple myeloma and diffuse large B-cell lymphoma. Br J Haematol. 2022 Mar;196(5):1274–1277.
- Crump M, Neelapu SS, Farooq U, et al. Outcomes in refractory diffuse large B-cell lymphoma: results from the international SCHOLAR-1 study. Blood. 2017 Aug 03;130(16):1800–1808. doi: 10.1182/blood-2017-03-769620
- Neelapu SS, Locke FL, Bartlett NL, et al. Comparison of 2-year outcomes with CAR T cells (ZUMA-1) vs salvage chemotherapy in refractory large B-cell lymphoma. Blood Adv. 2021 Oct 26;5(20):4149–4155. doi: 10.1182/bloodadvances.2020003848
- Methley AM, Campbell S, Chew-Graham C, et al. PICO, PICOS and SPIDER: a comparison study of specificity and sensitivity in three search tools for qualitative systematic reviews. BMC Health Serv Res. 2014 Nov 21;14(1):579. doi: 10.1186/s12913-014-0579-0
- Liberati A, Altman DG, Tetzlaff J, et al. The PRISMA statement for reporting systematic reviews and meta-analyses of studies that evaluate healthcare interventions: explanation and elaboration. BMJ. 2009 Jul 23;339(jul21 1):b2700. doi: 10.1136/bmj.b2700
- Peterson J, Welch V, Losos M, et al. The Newcastle-Ottawa scale (NOS) for assessing the quality of nonrandomised studies in meta-analyses. Ottawa: Ottawa Hospital Research Institute; 2011. p. 1–12.
- Salanti GH, Ades AE, Ioannidis JPA. Evaluation of networks of randomized trials. Stat Methods Med Res. 2008;17(3):279–301. doi: 10.1177/0962280207080643 Stat Methods Med Res.
- Lu GA, Ades AE. Assessing evidence inconsistency in mixed treatment comparisons. J Am Stat Assoc. 2006;101(474):447–459. doi: 10.1198/016214505000001302 J Am Stat Assoc.
- Dias S, Welton NJ, Sutton AJ, et al. NICE DSU technical support document 2: a generalised linear modelling framework for pairwise and network meta-analysis of randomised controlled trials. London (UK): National Institute for Health and Clinical Excellence; 2011.
- Macaskill P, Gatsonis C, Deeks JJ, et al. Chapter 10: Analysing and Presenting Results. In: Deeks JJ, Bossuyt PM, Gatsonis C, editors. Cochrane Handbook for Systematic Reviews of Diagnostic Test Accuracy Version 1.0. The Cochrane Collaboration; 2010. http://srdta.cochrane.org/
- Maziarz RT, Zhang J, Yang H, et al. Indirect comparison of tisagenlecleucel and historical treatments for relapsed/refractory diffuse large B-cell lymphoma. Blood Adv. 2022 Apr 26;6(8):2536–2547. doi: 10.1182/bloodadvances.2021006280
- Salles G, Spin P, Liu FF, et al. Indirect treatment comparison of liso-cel vs. salvage chemotherapy in diffuse large B-Cell lymphoma: transcend vs. scholar-1 [research support, non-U.S. Gov’t]. Adv Ther. 2021, 06;386: 3266–3280. doi: 10.1007/s12325-021-01756-0
- Cartron G, Fox CP, Liu FF, et al. Matching-adjusted indirect treatment comparison of chimeric antigen receptor T-cell therapies for third-line or later treatment of relapsed or refractory large B-cell lymphoma: lisocabtagene maraleucel versus tisagenlecleucel. Exp Hematol Oncol. 2022 Dec;11(1). no pagination. doi: 10.1186/s40164-022-00268-z
- Maloney DG, Kuruvilla J, Liu FF, et al. Matching-adjusted indirect treatment comparison of liso-cel versus axi-cel in relapsed or refractory large B cell lymphoma [clinical trial, phase i;comparative study; multicenter study; research support, non-U.S. Gov’t]. J Hematol Oncol. 2021 Sep 08;14(1):140. doi: 10.1186/s13045-021-01144-9
- Oluwole OO, Jansen JP, Lin VW, et al. Comparing efficacy, safety, and preinfusion period of axicabtagene ciloleucel versus tisagenlecleucel in relapsed/refractory large B cell lymphoma. Biol Blood Marrow Transplant. 2020 Sep;26(9):1581–1588.
- Oluwole OO, Chen JMH, Chan K, et al. Matching-adjusted indirect comparison of axi-cel and liso-cel in relapsed or refractory large B-cell lymphoma. Leukemia Lymphoma. 2022;63(13):3052–3062. doi: 10.1080/10428194.2022.2113526
- Schuster SJ, Zhang J, Yang H, et al. Comparative efficacy of tisagenlecleucel and lisocabtagene maraleucel among adults with relapsed/refractory large B-cell lymphomas: an indirect treatment comparison. Leukemia Lymphoma. 2022;63(4):845–854. doi: 10.1080/10428194.2021.2010069
- Van DNE, Schmitz N, Mounier N, et al. Outcome of patients with relapsed diffuse large B-cell lymphoma who fail second-line salvage regimens in the international CORAL study. Bone Marrow Transplant. 2016 [cited 2015 Sep 14];51(1):51–57. doi: 10.1038/bmt.2015.213
- Gisselbrecht C, Glass B, Mounier N, et al. Salvage regimens with autologous transplantation for relapsed large B-cell lymphoma in the rituximab era. J Clin Oncol. 2010 Jul 26;28(27):4184–4190. doi: 10.1200/JCO.2010.28.1618
- Locke FL, Ghobadi A, Jacobson CA, et al. Long-term safety and activity of axicabtagene ciloleucel in refractory large B-cell lymphoma (ZUMA-1): a single-arm, multicentre, phase 1-2 trial [clinical trial, phase i; clinical trial, phase ii; multicenter study; research support, n.i.h. extramural; research support, non-U.S. Gov’t]. Lancet Oncol. 2019 01;20(1):31–42. doi: 10.1016/S1470-2045(18)30864-7
- Gao J, Dahiya S, Patel SA. Challenges and solutions to superior chimeric antigen receptor-T design and deployment for B-cell lymphomas. Br J Haematol. 2023 Oct;203(2):161–168. doi: 10.1111/bjh.19001
- Evans SR. Fundamentals of clinical trial design. J Exp Stroke Transl Med. 2010 Jan 1;3(1):19–27. doi: 10.6030/1939-067X-3.1.19
- Bethge WA, Martus P, Schmitt M, et al. GLA/DRST real-world outcome analysis of CAR-T cell therapies for large B-cell lymphoma in Germany. Blood. 2022:22. doi: 10.1182/blood.2021015209
- Westin JR, Kersten MJ, Salles G, et al. Efficacy and safety of CD19-directed CAR-T cell therapies in patients with relapsed/refractory aggressive B-cell lymphomas: observations from the JULIET, ZUMA-1, and TRANSCEND trials. Am J Hematol. 2021 Oct 1;96(10):1295–1312. doi: 10.1002/ajh.26301
- Locke FL, Hu Z, Siddiqi T, et al. Real-world impact of time from leukapheresis to infusion (vein-to-vein time) in patients with relapsed or refractory (r/r) large B-cell lymphoma (lbcl) treated with axicabtagene ciloleucel. Blood. 2022;140(Supplement 1):7512–7515. doi: 10.1182/blood-2022-155603
- Buecklein VL, Perez-Perez A, Rejeski K, et al. Inferior outcomes of EU Vs. US patients with relapsed/refractory large B-Cell Lymphoma after CD19 CAR T-Cell therapy are associated with differences in tumor burden, systemic inflammation, bridging therapy utilization and CAR-T product selection. ASH. Blood. 2022;140(Supplement 1):2411–2413. doi: 10.1182/blood-2022-165452
- Cartron G, Fox CP, Liu FF, et al. Matching-adjusted indirect treatment comparison of chimeric antigen receptor T-cell therapies for third-line or later treatment of relapsed or refractory large B-cell lymphoma: lisocabtagene maraleucel versus tisagenlecleucel. Exp Hematol Oncol. 2022;11(1). doi: 10.1186/s40164-022-00268-z
- Schuster SJ, Zhang J, Yang H, et al. ABCL-166: tisagenlecleucel and lisocabtagene maraleucel: comparative efficacy in patients with relapsed/refractory diffuse large B-cell lymphoma [conference abstract]. Clin Lymphoma Myeloma Leuk. 2021 Sep;21(Supplement 1):S380–S381.