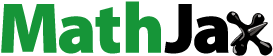
ABSTRACT
Introduction
Central nervous system infections (CNSI) disproportionately affect individuals in low-resource settings where diagnosis is challenging; large proportions of patients never receive a confirmed microbiological diagnosis resulting in inadequate management and high mortality. The epidemiology of CNSI varies globally and conventional diagnostics deployed in resource-limited settings have significant limitations, with an urgent need for improved diagnostic strategies.
Areas covered
This review describes molecular platforms and other novel diagnostics used in the diagnosis of CNSI that are applicable to resource-limited settings. An extensive literature search of Medline and PubMed was performed. The emphasis is on investigations targeting infections of relevance to resource-limited settings either due to variation in regional CNSI epidemiology or due to increased prevalence in patients with immunosuppression. This includes commercially available multiplex PCR platforms, mycobacterial PCR platforms, and rapid diagnostics tests. To offer a framework for the optimal implementation in clinical settings, existing evidence highlighting the advantages and limitations of available platforms is reviewed.
Expert opinion
The implementation of molecular platforms and other novel diagnostics has the potential to transform CNSI diagnosis in resource-limited settings, with several examples of successful rollout of novel diagnostics such as Xpert MTB/RIF Ultra and cryptococcal antigen testing.
1. Introduction
Central nervous system infections (CNSI) lead to a significant burden of morbidity and mortality globally [Citation1–4]. The burden of CNSI is highest in resource-limited settings where outcomes are often poor due restricted access to healthcare during acute infection and few resources to support survivors with long-term neurological sequelae [Citation1,Citation5]. Diagnosis of CNSI is challenging, and a large proportion of patients never receive a confirmed microbiological diagnosis, particularly in resource-limited settings where access to modern diagnostic platforms is limited [Citation6,Citation7]. The epidemiology of CNSI in resource-limited settings is therefore poorly understood making presumptive diagnosis and empirical treatment challenging. There is also significant regional variation in pathology. Japanese encephalitis, leptospirosis, scrub typhus and Streptococcus suis are important causes of CNSI in South-east Asia but rarely encountered in Europe or North America [Citation8]. Meningococcal meningitis predominates in the meningitis belt of Africa extending from Senegal to Ethiopia with epidemics of meningococcal meningitis occurring in the dry season with attack rates as high as 1% of the population in major epidemics [Citation9]. In much of Africa, HIV has drastically altered CNSI epidemiology with cryptococcal and tuberculous meningitis now being the most commonly-encountered pathogens in the region [Citation10,Citation11]. However, the prevalence of other central nervous system opportunistic infections associated with advanced HIV disease such as Toxoplasma gondii and JC virus is largely unknown outside of resource-rich settings. Patients with CNSIs who do not receive a diagnosis have an unacceptably high mortality. Data from Botswana demonstrated a 40% mortality at 10 weeks in patients with a CSF pleocytosis but no confirmed microbiological diagnosis after investigation with conventional techniques, indicating the presence of widespread pathology that needs enhanced diagnostic infrastructure in place to inform treatment decisions and improve outcomes [Citation12].
Standard diagnostic investigations for suspected CNSIs in resource-limited settings are usually restricted to CSF microscopy, culture and sensitivity, Gram, Ziehl-Neelsen, and India ink stains, and cryptococcal antigen (CrAg) testing. However, in many locations even these routine investigations may be unavailable. CSF culture requires significant technical skill from the operator and considerable resources including dedicated laboratory space, specialized agars, centrifuges, and incubators to be performed effectively. In addition, culture techniques can take up to a week for fungal and bacterial culture and up to 6 weeks for culture of Mycobacteria tuberculosis and therefore have limited impact on immediate clinical decision-making. Whilst these standard investigations have a role in the diagnosis of meningitis, with the notable exception of CrAg, they all have significant limitations; there is an urgent need to improve diagnosis of CNSI in resource-limited settings.
Rapid molecular diagnostic platforms tailored to local epidemiology have the potential to significantly enhance diagnostic capabilities and are well suited for use on cerebrospinal fluid (CSF) samples. Contamination of CSF during sample collection is relatively uncommon, disease processes are usually caused by a single pathogen (other than in patients with significant immunosuppression) and common inhibitors of nucleic acid amplification are usually not present in CSF [Citation13]. As a result their implementation in high-resource settings has redefined diagnostic strategies for CNSI [Citation14]. However, in resource-limited settings there are significant challenges to widespread implementation of molecular diagnostics. In sub-Saharan Africa, due to high HIV-prevalence, cryptococcal and tuberculous meningitis are the most common causes of CNSI. Cryptococcal meningitis is not reliably diagnosed through current nucleic acid amplification techniques (NAAT) as most fungi have dense cell walls that are difficult to breakdown resulting in decreased yield from traditional DNA extraction techniques [Citation15]. Due to low bacillary burdens in CSF, tuberculous meningitis has historically been challenging to diagnose using molecular platforms but some more modern platforms have improved sensitivity. Co-infections remain rare but have been increasingly reported in immunosuppressed patients, requiring a broader range of targeted investigations for suspected CNSI [Citation11,Citation16,Citation17]. There are additional structural challenges such as unreliable power supplies, limited access to cold chain shipping and storage, and cost considerations. Despite these challenges, there are examples of successful implementation of novel diagnostic platforms for the investigation of suspected CNSI in resource-limited settings. Cryptococcal antigen testing is now the leading modality used to diagnose cryptococcal meningitis and Xpert MTB/RIF Ultra has emerged as an increasingly useful tool in the investigation of tuberculous meningitis (TBM), although it remains insufficiently sensitive to rule out TBM [Citation17].
One of the primary barriers to widespread adoption of molecular diagnostics in resource-limited settings is financial. Although the cost of molecular diagnostics has decreased over the recent years, and discretionary pricing is available in resource-limited settings for some platforms such as Xpert MTB/RIF Ultra, they remain relatively expensive to implement and maintain. Understanding the advantages and limitations of these platforms, and how they can be optimally implemented as part of locally-appropriate diagnostic algorithms, is crucial to maximize their clinical- and cost-effectiveness.
To conduct this narrative review a list of low- and middle-income countries defined by the world bank was compiled, termed ‘LMIC.’ PubMed/Medline (1946 to 2023) were searched for: [LMIC] AND [meningitis.mp. or exp Meningitis/or exp Encephalitis/or encephalitis.mp. or meningoencephalitis.mp. or exp Meningoencephalitis/or central nervous system infections.mp. or exp Central Nervous System Infections/] AND [exp Molecular Diagnostic Techniques/or exp Rapid Diagnostic Tests/or polymerase chain reaction.mp. or exp Polymerase Chain Reaction/or exp Metagenomics/or metagenomic sequencing.mp or sequencing.mp or diagnostics.mp or diagnosis.mp]
This review aims to describe novel and molecular platforms used in the diagnosis of CNSI that are applicable to resource-limited settings. To provide context for their optimal use in clinical settings, existing evidence highlighting the advantages and limitations of available platforms is reviewed, covering conventional CSF investigations, soluble markers of fungal and mycobacterial infection, and antigen detection techniques. The focus is on pathogen-specific investigations of particular importance in resource-limited settings either due to significant variations in CNSI epidemiology from resource-rich settings or due to increased prevalence in patients with immunosuppression. Finally, commercially available multiplex PCR platforms are discussed, focusing on their use in resource-limited settings.
2. Conventional CSF investigations
2.1. Microscopy
It provides a cell count and differential that gives initial guidance on the underlying pathogen based on the predominant white blood cell type seen in the CSF. Neutrophilic pleocytosis is typically seen more in bacterial meningitis and lymphocytic pleocytosis in viral and tuberculous meningitis [Citation18]. The test requires laboratory infrastructure and training and must be performed quickly to prevent degeneration of cells. Interpretation is not always straightforward as there is often overlap in patterns of cell differential, for example late presentations of bacterial meningitis can be lymphocytic and early presentations of TBM neutrophilic. In addition, CSF white cell counts can vary with age, race, and immune status [Citation19,Citation20]. Despite its limitations cell count and differential remain crucial first-line investigations that allow other results to be contextualized.
2.2. Gram stain
It is used to classify bacteria based on morphology and cell wall properties. It is quick, relatively inexpensive, and less affected by antimicrobial administration than culture. However, the sensitivity is poor (10–90% depending on organism) and is highly operator dependent [Citation21,Citation22].
2.3. India ink stain
It is used to identify encapsulated yeasts, primarily Cryptococcus neoformans. Like gram stain it is rapid, inexpensive and highly operator dependent. The sensitivity is superior to gram stain, around 70–80% [Citation23], but it cannot distinguish between active and past treatment as it will stay positive even after successful treatment. Therefore positive results must be interpreted in the correct clinical context.
2.4. Culture
CSF culture for bacteria can yield a result in 24–48 hours depending on the organism and pathogen burden, but results can take over a week. Time to result is usually longer in fungal cultures and most cultures are incubated for up to 10 days. Culture remains the gold standard in diagnosis of bacterial meningitis although it is only positive in 70–85% of bacterial meningitis cases [Citation24]. It is currently the only readily available diagnostic modality that provides reliable information on antimicrobial susceptibility from CSF samples. Culture sensitivity is affected by prior antimicrobial administration and sterile CSF has been reported within the first 2 hours of administration of antibiotics in meningococcal meningitis and 4 hours in pneumococcal meningitis [Citation25]. However, depending on the pathogen culture may still be beneficial up to 2 days after antibiotics [Citation14].
2.5. CSF protein
Raised CSF protein is a nonspecific finding that can be suggestive of CNSI. However, There are multiple non-infectious causes of raised CSF protein such as hemorrhage, malignancy, and Guillain-Barre syndrome. Neonates and children under 1 year old also have higher CSF protein levels than adults. Access to biochemistry in resource limited settings is often limited to larger hospitals.
2.6. CSF glucose
Ideally used as a ratio with plasma glucose where a low ratio or absolute value is suggestive of active infection. Classically TBM causes extremely low glucose levels. Access to plasma glucose is often restricted in resource-limited settings but bedside tests are also available.
3. Soluble markers
3.1. Beta-D-glucan
Beta-D-glucan (BDG) is a polysaccharide component of the cell wall of multiple fungi including Candida species, Histoplasma species, Coccidioides species and Aspergillus species. It is widely used on blood in high-resource settings to diagnose or monitor treatment progress in invasive fungal infections. However, it is not specific to a particular fungal species and several factors can lead to false-positive in serum such as hemodialysis, antibiotics, and surgical gauzes although limited data exists on false-positive in CSF [Citation26,Citation27]. A systematic review evaluated its use on CSF and found sensitivity varied by species and organism-specific testing was still warranted in patients with a raised BDG [Citation28]. Its main strengths are that it has a faster turnaround time than fungal cultures and has the potential to monitor treatment progress in BDG-producing fungi.
3.2. Galactomannan
Galactomannan is most commonly found in the cell wall of Aspergillus species and is used in a similar fashion to BDG in the diagnosis and monitoring of aspergillosis mostly in serum. False-positive from antibiotics are seen in serum and slow-growing fungal infections can lead to false-negative. Based on serum cutoff values, CSF galactomannan was shown to have a sensitivity of 100% in the diagnosis of CNS aspergillosis with a specificity of 70% [Citation29]. It is not widely used in CNSI diagnostics in resource-limited settings.
3.3. Adenosine deaminase
Adenosine deaminase (ADA) is an enzyme involved in the metabolism of purines that plays a crucial role in the differentiation and proliferation of lymphocytes. Increased ADA activity is associated with conditions that involve a cell-mediated response including TB. ADA testing to diagnose TBM has been widely considered in low-resource settings but its utility remains unclear due to an inability to determine optimal cutoff values for use on CSF and a significant number of infectious and noninfectious conditions apart from TBM causing elevated ADA [Citation30–32].
4. Antigen testing
4.1. Cryptococcal meningitis
Cryptococcal antigen (CrAg) is a polysaccharide found on the cell wall of Cryptococcus species. There are several commercially available CrAg lateral flow assays (LFA) but by far the most used is the IMMY CrAg LFA; an immunochromatographic dipstick that has revolutionized the diagnosis of cryptococcal meningitis worldwide. It is cheap, does not require significant laboratory capacity and can give results in 15 minutes. In HIV-positive patients it has a sensitivity of 98.8% in CSF; superior to both India ink and culture [Citation33,Citation34]. Data from HIV-negative patients is limited but the incidence of cryptococcal meningitis in HIV negative patients is increasing mostly due to more widespread use of immunosuppressive therapies. A recent systematic review demonstrated a similar sensitivity in CSF in HIV-negative patients, 99% [Citation33]. A key limitation to CSF CrAg testing is that it is unable to differentiate between active and treated infection. Newer CrAg LFAs allow semi quantification of CrAg titer that may predict disease burden and culture positivity thereby mitigating one of the limitations of CrAg LFA. Further studies are needed to determine if semi quantification of CrAg titers could also be useful to determine disease severity and potentially inform management [Citation35]. False-negative in both CrAg LFA and semiquantitative CrAg LFA can occur in high fungal burdens due to the prozone effect, although this can be overcome with serial dilutions.
4.2. Bacterial meningitis
Latex agglutination tests have been employed to detect common bacteria causing CNSI. They provided only a small increase in sensitivity above culture and required significant laboratory infrastructure and cold chains which restricted their use in resource-limited settings [Citation22]. Similar lateral flow immunochromatographic technologies to CrAg testing have been developed to detect the presence of a number of bacteria. Primarily these target Streptococcus pneumoniae and Neisseria meningitidis and have been used more widely than latex agglutination tests. The Abbott BinaxNOW Streptococcus pneumoniae antigen card and BioSpeedia Pneumospeed both have sensitivities and specificities over 90% for the detection of S.pneumoniae [Citation36]. Rapid diagnostic tests that can detect N. meningitidis are crucial for surveillance and early detection of meningococcal disease in the meningitis belt as despite the introduction of large-scale vaccination programmes outbreaks of meningococcal meningitis still occur [Citation37]. The LFA BioSpeedia Meningospeed detects the five serogroups in currently licensed meningococcal vaccines, serogroups A, C, W, X and Y. It has a sensitivity of 92.7% and can partially differentiate between serotypes, which is potentially beneficial for monitoring of vaccination strategy success [Citation37].
5. Mycobacterial testing
Tuberculous meningitis (TBM) is the most severe form of tuberculosis (TB) and is a leading cause of meningitis in resource-limited, high HIV-prevalence settings [Citation4,Citation17,Citation38,Citation39]. A modeling study estimated that 164,000 people developed tuberculous meningitis in 2019, with 70% of the global incidence occurring in South-East Asia and Africa. TBM occurs in 1.5% of tuberculosis cases in HIV-negative patients and up to 5% of cases in HIV-positive adults and carries a significant mortality with approximately 78,000 deaths globally in 2019. Early diagnosis is crucial to avoid poor outcomes but due to inadequacies in current diagnostics approximately 59% of patients with TBM died without a confirmed diagnosis and therefore patients are often either treated empirically on clinical suspicion alone with no understanding of potential antimicrobial resistance or do not receive appropriate anti-tuberculous therapy at all [Citation4,Citation40].
5.1. Conventional diagnostics for tuberculous meningitis
The routinely available investigations in resource-limited settings to detect Mycobacterium tuberculosis in CSF are microscopy with Ziehl-Neelsen (ZN) stain and culture for M. tuberculosis. Microscopy with ZN staining is the cheapest and most rapid form of smear microscopy available to identify M. tuberculosis. It can be performed with less laboratory infrastructure than culture for M. tuberculosis and is widely used for pulmonary samples in both high-resource and resource-limited settings. However, the sensitivity of ZN staining on paucibacillary samples such as CSF is poor with estimates on CSF as low as 9% [Citation41,Citation42]. However considerably higher sensitivity of microscopy with ZN staining has been reported in centers where samples are analyzed by skilled microscopists for a minimum of 30 minutes suggesting the sensitivity is highly operator dependent [Citation43].
TB culture using solid or liquid media remains the gold standard for TBM diagnosis despite significant limitations. The limit of detection for TB culture is approximately 10 CFU/ml and has a sensitivity of 30–60% [Citation17,Citation44]. To produce a positive result, it requires viable bacteria to be present in a sample and can take up to 6 weeks to produce a positive result meaning it cannot inform immediate treatment decisions [Citation17,Citation43].
5.2. Molecular diagnostics for mycobacterium tuberculosis
In 2010, the WHO approved Xpert MTB/RIF (Cepheid, U.S.A.) for use in pulmonary and extrapulmonary samples [Citation38]. Xpert MTB/RIF is a cartridge-based, fully automated system that allows the rapid detection of M. tuberculosis and associated rifampicin resistance through nucleic acid amplification of the rpoB gene, a region that has been demonstrated to be highly predictive of rifampicin resistance [Citation17]. The test provides a marked improvement in accessibility to TB diagnostics and turnaround time when compared to culture with results available in under 2 hours. However, the sensitivity of Xpert MTB/RIF is dependent on multiple factors including sample type, CSF volume, prior centrifugation and the patient’s HIV status [Citation44]. A large multicentre study in 2018 performed across Indonesia, South Africa and Vietnam evaluated the performance of Xpert MTB/RIF, Ziehl-Neelsen stain and CSF culture of 618 adults with suspected TB meningitis [Citation4]. Xpert MTB/RIF was positive in 95 out of 379 patients with diagnosis of TB based on a uniform case definition [Citation4]. The sensitivity of Xpert MTB/RIF assay was 25.1% (95% CI 21.0–29.7%) and specificity 72.3% (95% CI not reported) when compared with culture [Citation4]. A meta-analysis from 2021 included studies performed in both resource-limited and resource-rich settings and compared the use of Xpert MTB/RIF on CSF against a composite reference standard. 14 studies were included with a total of 2203 participants and it demonstrated a pooled sensitivity 42.3% (95% Cl 32.1 to 52.8) and pooled specificity of 99.8% (95% Crl 99.3 to 100.0) [Citation45]. As such, whilst Xpert MTB/RIF provided some improvements in TBM diagnosis above conventional investigations there remained significant limitations and it was insufficiently sensitive to be implemented as a reliable standalone test for the diagnosis of TBM.
Xpert MTB/RIF Ultra is now the current initial diagnostic test for TBM recommended by WHO in 2017 [Citation38]. Similar to Xpert MTB/RIF, Xpert MTB/RIF Ultra provides semiquantitative categories and information on rifampicin resistance. Xpert MTB/RIF Ultra has incorporated two additional PCR targets (IS6110 and IS1081), alongside a fully nested real time PCR assay and a DNA reaction chamber twice the size of Xpert MTB/RIF. These changes combine to allow Xpert MTB/RIF Ultra to have a lower limit of detection than Xpert MTB/RIF, 16 CFU/ml vs 114 CFU/ml respectively, an almost a 7-fold improvement [Citation17,Citation38]. Furthermore, the melting temperature-based analysis increases the accuracy of rifampicin resistance detection using four probes that identify rifampicin resistance mutations in the rpoB gene. The entire process takes less than one and a half hours thus producing faster and more accurate results [Citation17,Citation38,Citation43].
Most data surrounding the use Xpert MTB/RIF Ultra in resource-limited settings have been generated from two large prospective trials. The first study from Vietnam randomized 205 adults with suspected TBM to testing with either Xpert MTB/RIF and Xpert MTB/RIF Ultra [Citation43]. Using a uniform case definition as a reference standard Xpert MTB/RIF Ultra was not superior to Xpert MTB/RIF, sensitivities were 39.6% (95% CI 27.6 to 53.1) and 47.2% (95% CI 34.4 to 60.3) for Xpert MTB/RIF and Xpert MTB/RIF Ultra respectively. The sensitivity of both assays was higher in HIV positive patients but there remained no improvement of Xpert MTB/RIF Ultra over Xpert MTB/RIF. Xpert MTB/RIF Ultra did perform better in samples with a lower bacillary load and in those who had started treatment [Citation43].
The second study conducted in Uganda analyzed CSF samples from 204 patients with Xpert MTB/RIF Ultra, Xpert MTB/RIF and mycobacterial growth indicator tube culture 196 of whom were HIV positive [Citation17]. The sensitivity was significantly higher in Xpert MTB/RIF Ultra compared to Xpert MTB/RIF and MGIT culture against ‘probable’ and ‘definite’ categories within the uniform clinical definition. The sensitivities were 55.6% (95% CI 44.0 to 70.4%) for Xpert MTB/RIF, 61.4% (95% CI 45.5 to 75.6) for MGIT culture and 76.5% (95% CI 62.5 to 96.2) for Xpert MTB/RIF Ultra [Citation17]. Individuals with ‘possible’ TBM based on a uniform case definition were not included as this category is less specific in patients with HIV co-infection due to the broad differential in patients with advanced immunosuppression. Importantly 36% of positive Xpert MTB/RIF Ultra were in the lowest semiquantitative detection category which correlates to < 100 CFUs/ml. This would be below the limit of detection for Xpert MTB/RIF and potentially also TB culture highlighting a potential population in whom Xpert MTB/RIF Ultra is of particular benefit.
The difference in performance of Xpert MTB/RIF Ultra in these studies is likely due to difference in sample handling, CSF volume submitted for analysis, HIV co-prevalence and study design (specimens were divided for use across all modalities in the Uganda study and randomized to individual analysis in the Vietnam study) [Citation46]. A meta-analysis including both these studies and two others demonstrated a pooled sensitivity of 62.7% (95% CI 45.7 to 77.0) and 42.3% (95% CI 32.1 to 52.8) against a composite reference standard for Xpert MTB/RIF Ultra and Xpert MTB/RIF respectively34.
These studies all suggest that although Xpert MTB/RIF Ultra is a significant improvement it remains insufficiently sensitive to exclude TBM with a negative result. The Uganda study reported nine adults with a negative Xpert MTB/RIF Ultra that had clinical features and CSF results highly suggestive of TBM with one adult having macroscopic features suggestive of TBM on postmortem [Citation17]. As such Xpert MTB/RIF Ultra results should be interpreted in the context of clinical presentation, surrogate investigations suggestive of TBM such as routine CSF analysis, imaging and local TB epidemiology.
5.3. Lateral flow lipoarabinomannan assays
Xpert MTB/RIF Ultra does have some additional limitations beyond its imperfect sensitivity. These include the need for a reliable power source, laboratory infrastructure and staff training. Lateral flow lipoarabinomannan assays (LAM) are well established for use in the urine to detect a constituent part of the cell wall of the TB bacillus in patients with TB. A recent meta-analysis demonstrated that a newly developed TB-LAM assay, SILVAMP TB-LAM, has a superior sensitivity compared to the most widely used assay Alere Determine TB LAM, when used to diagnose pulmonary and extrapulmonary TB in HIV-positive adults [Citation47]. SILVAMP TB-LAM combines a pair of high-affinity monoclonal antibodies for M. tuberculosis-specific LAM epitopes and has a silver-amplification step to improve band visibility and detect LAM at concentrations 30 times lower than Alere Determine TB LAM. Previous trials using Alere Determine TB LAM on CSF to diagnose TBM have not been promising but there is encouraging data from Uganda investigating the use of SILVAMP TB-LAM [Citation48]. In a prospective cohort study of 101 adults, including 95 HIV-positive patients, 34 participants had definite TBM and 24 had probable TBM. Amongst the 58 individuals with definite or probable TBM, 30/58 tested positive with SILVAMP TB-LAM compared to 32/58 for Xpert MTB/RIF Ultra. Against a uniform case definition, SILVAMP TB-LAM had a sensitivity comparable to Xpert MTB/RIF Ultra of 52% (95% CI 38–65%) and 55% (95% CI 42–68%) respectively [Citation49]. However, due to manufacturing challenges the availability of this test has been severely limited. A newer version has been recently released but is awaiting validation.
5.4. Truenat MTB and MTB plus
Truenat MTB assay (Molbio Diagnostics, India) is a chip-based nucleic acid amplification platform originally developed to meet the requirements of Indian primary health centers for near point-of-care TB testing [Citation50]. It is portable and battery operated therefore useful in remote locations, and able to operate at temperatures up to 40°C. A second-generation platform is now in circulation, Truenat MTB Plus. The test takes approximately 2 hours but is broken down in to three discrete states, DNA extraction, M. tuberculosis detection and rifampicin resistance testing. A study conducted in 2018 in India compared Truenat MTB Plus with Xpert MTB/RIF Ultra using CSF. Truenat MTB Plus had an overall sensitivity of 78.7%, while Xpert MTB/RIF Ultra had an overall sensitivity of 67.6% in diagnosing probable and definite TBM [Citation50]. Although the sensitivity was comparable to Xpert MTB/RIF Ultra in the study, rifampicin resistance detection was inferior, with 10 rifampicin resistant cases missed by Truenat MTB Plus [Citation50].
6. Targeted testing for selected single pathogens
6.1. Progressive multifocal leukoencephalopathy
John Cunningham Virus (JCV) is the cause of progressive multifocal leukoencephalopathy (PML) a demyelinating disorder that prior to the rollout of ART in North American and European communities, affected up to 5% of patients with advanced HIV. PML is usually caused by the reactivation of latent JCV in immunocompromised patients due to conditions such as HIV or the use immunomodulatory agents [Citation51–53]. Alongside classic PML, immune reconstitution inflammatory syndromes (IRIS) associated with PML are increasingly recognized. This results from the reversal of profound immunosuppression, such as a rapid rise in CD4 following ART initiation or cessation of immunosuppressive drugs such as natalizumab in multiple sclerosis [Citation54]. The clinical features of PML are highly heterogenous and diagnosis can only be confirmed with a positive JCV PCR from CSF or brain biopsy alongside supportive imaging and clinical features, or typical neuropathologic findings. Whilst older PCRs had a sensitivity of approximately 75% modern PCRs have a sensitivity of over 95% and have prognostic value, with higher CSF JCV DNA loads shown to be associated with poorer outcomes [Citation53,Citation55]. Despite improvements in PCR sensitivity, it is still possible to have a negative PCR with clinical and radiological features compatible with PML. As such PML diagnosis remains challenging and consensus guidelines have suggested repeating a lumbar puncture and JCV PCR if the first is negative. Even with adequate resources the diagnosis of PML is often delayed with the median time to diagnosis reported as 74 days in a single US center [Citation55,Citation56].
The epidemiology of PML in resource-limited settings is poorly understood. Prevalence estimates are more difficult to establish as molecular diagnostics are less readily available and therefore the diagnoses of PML are often based on clinical or radiological features which are less specific than JCV PCR [Citation57,Citation58]. This has led to significant variability in the frequency of reported PML in previous studies performed in resource-limited settings. Retrospective data from India and Brazil described PML in 2.8% and 0.3% of HIV positive patients respectively [Citation59]. Whilst these differences might be related to genetic or viral factors it more likely reflects the limitations in availability of reliable diagnostics. When JCV PCR testing has been implemented programmatically on CSF samples it has demonstrated similar or higher PML prevalence when compared to pre-ART studies from U.S.A./Europe. Over 15% of CSF (19/121) samples in South Africa and 6% (20/331) of samples in Zambia were positive for JCV on PCR [Citation10,Citation60]. To allow emerging treatment options for PML to be best utilized in resource-limited settings expanded JCV testing capacity or inclusion in multiplex PCR panels will be necessary [Citation61].
6.2. Cerebral toxoplasmosis
Toxoplasma gondii is an obligate intracellular protozoan which causes toxoplasmosis, a common self-limiting condition in immunocompetent individuals. However, in patients with immunosuppression toxoplasma can reactivate; most commonly causing cerebral toxoplasmosis in patients not receiving ART and appropriate toxoplasma prophylaxis [Citation62,Citation63].
Approximately 30% of the world’s population is estimated to be infected with T. gondii and the highest burden of T. gondii infection in individuals with HIV is in lower income countries with 87.1% of HIV and T. gondii co-infections being in sSA [Citation64]. In resource-limited settings cerebral toxoplasmosis is usually diagnosed presumptively based on compatible clinical features and imaging, and positive toxoplasma serology when available. PCR techniques exist but their use is mostly limited to resource-rich settings. The sensitivity estimates of available PCRs is broad due to the highly heterogenous PCR methods used for the detection of toxoplasma, with estimates between 50–98% [Citation65]. These includes using different primer sets, DNA targets, and hybridization probes [Citation66]. Furthermore, pre-analytic steps such as different extraction techniques and administration of anti-toxoplasma treatment also influence assay performance making comparisons between PCR modalities challenging. PCR targets have evolved over recent years with the most recently proposed target being the GRA7 gene. This region is expressed during all infectious stages of the parasite’s lifecycle and has been shown to have superior sensitivity to previous targets [Citation67]. A study in Zambia using an older target, B1 gene, identified no cases of Toxoplasma amongst 331 HIV positive adults [Citation10]. Accurate, reliable and more accessible platforms are needed to diagnose toxoplasma. Loop-mediated isothermal amplification (LAMP) techniques to detect T. gondii have been developed with some promise but there is limited data from CSF. They present a more portable, rapid diagnostic test that requires less infrastructure and may be practical in resource-limited settings.
6.3. Japanese encephalitis
Japanese encephalitis virus (JEV) is a vector borne Flavivirus transmitted by Culex spp mosquitoes. JEV is endemic in most of Asia and some areas in the Western Pacific with more recent detection of the virus in Australia [Citation68,Citation69]. It is the most important cause of viral encephalitis in Asia with an estimated 68,000 cases each year with 20,000 deaths [Citation68]. The majority of JEV infections occur in children living in rural areas when JEV-infected mosquitoes feed on humans rather than swine, their principal amplifying host.
Diagnosis is typically made through the detection of anti-JEV IgM antibodies in blood or CSF. However cross reactivity of immune-assays targeting flaviviruses is well-described [Citation70]. This is of particular relevance for dengue virus that is co-endemic in the region and exhibits similar seasonality to JEV. In some cases, vaccination against JEV can lead to prolonged IgM detection further challenging diagnostic certainty when relying on immune-assay testing alone [Citation71]. Direct detection of JEV RNA would provide a valuable indication of infection as it would be able to overcome some limitations of immune-assay based testing and allow improved specificity. However widespread use of JEV PCR assays has been challenging due to the low sensitivity of currently available techniques, a short period of JEV viremia and low CSF concentrations of JEV in infected humans [Citation70,Citation71].
6.4. Neurosyphilis
The WHO estimated the prevalence of syphilis to be 1.1% in Asia and 4–6.5% in Africa in 2016 [Citation72]. Neurosyphilis is relatively rare but is more commonly seen in patients living with HIV with neurosyphilis described in 3% of all cases of syphilis [Citation73,Citation74]. The laboratory diagnosis of neurosyphilis remains imperfect and relies upon a high index of clinical suspicion alongside the presence of a CSF pleocytosis or elevated protein and a combination of non-treponemal or treponemal tests [Citation75]. The introduction of a PCR for T. pallidum was hoped to improve syphilis diagnostics however sensitivity estimates so far have been relatively modest. A systematic review reported the sensitivity of PCR for definite neurosyphilis of between 40–70% compared to reference testing for neurosyphilis [Citation76]. A commercially available immunochromatographic test, DPP Chembio syphilis assay, has been trialed with some early success demonstrating a sensitivity of 80% against a reference standard of reactive CSF-Venereal Disease Reference Laboratory that improved with treatment [Citation77]. At present the diagnosis of neurosyphilis remains reliant on a combination of multiple testing modalities and careful clinical interpretation.
7. Multiplex PCR assays
Multiplex polymerase chain reaction (PCR) panels can reduce the time to diagnosis, test for a number of pathogens that would not be detected through routine analysis alone and increase sensitivity above conventional techniques whilst using smaller sample volumes. Until recently the only commercially available multiplex PCR platform for use on CSF was BioFIRE FilmArray-Meningitis/Encephalitis panel (bioMerieiux, France) which has been proven to be effective in the diagnosis of CNSI in a number of large-scale trials in resource-rich settings [Citation78,Citation79]. In 2022 QIAGEN introduced the QIAstat-Dx meningitis/encephalitis panel that has recently been shown to have a comparable sensitivity to BioFIRE FilmArray-Meningitis/Encephalitis (FilmArray-ME) for analytes included in both panels [Citation80]. Both panels use 200 µL of neat CSF with no need for prior extraction and results are available in under 1.5 hours; analytes included in both panels are described in [Citation81].
Table 1. List of pathogens detected in BioFIRE FilmArray-Meningitis/Encephalitis and QIAstat-dx meningitis/Encephalitis panel.
Studies from resource-limited settings have solely used the FDA-approved FilmArray-ME that tests for 14 common CNS pathogens, the major studies are outlined in [Citation11,Citation16,Citation82–86]. FilmArray-ME was successfully implemented in multiple laboratories which often had little to no experience of using molecular diagnostics. In all studies FilmArray-ME increased the diagnostic yield of CSF analysis and in some cases viral pathogens were detected in the CSF for the first time in routine clinical practice. The number of bacterial meningitis diagnoses made was also increased above traditional culture techniques. FilmArray-ME has also been used to differentiate cerebral malaria by excluding other CNSI in pediatric febrile neurological presentations in malaria endemic areas [Citation16,Citation87]. Whilst the overall sensitivity of FilmArray-ME was high in all studies there was some reported intrapanel variation between the sensitivity of analytes with the performance of Cryptococcus neoformans/gattii being poorer than other analytes in FilmArray-ME, particularly in low fungal burden cryptococcal meningitis [Citation16]. There was only one positive Cryptococcus neoformans/gattii tested in the comparison of QIAstat-Dx meningitis/encephalitis to FilmArray-ME, so no reliable conclusions regarding diagnostic sensitivity for this pathogen can yet be made [Citation52]. In contrast to high-resource settings where the diagnosis of cryptococcal meningitis is often opportunistic the sensitivity of the cryptococcus analyte is of particular importance in high HIV-prevalence settings where cryptococcal meningitis is the most common cause of CNSI. Multiplex PCR testing should not be performed in isolation in patients with suspected cryptococcal meningitis due superior accuracy of CrAg testing.
Table 2. Major studies implementing BioFIRE FilmArray-ME in resource-limited settings in which data on BioFIRE FilmArray-ME could be disaggregated. Diagnostic yield displayed excludes PCR detection of Cryptococcus neoformans that was concordant with conventional techniques (e.g. CSF CrAg testing) with higher sensitivity. This was to display a more representative diagnostic yield as some of the larger studies in Uganda included a high proportion of HIV-positive patients with cryptococcal meningitis.
The cost of multiplex PCR panels will be a barrier to more widespread implementation but data from Ethiopia suggests that multiplex PCR testing decreased antibiotic use which would mitigate some of the increased cost [Citation83]. Furthermore, diagnostic yield varied between studies which had heterogenous inclusion criteria suggesting the use of these platforms could be targeted to higher yield groups as part of a clinically- and cost-effective diagnostic algorithm.
8. Metagenomic sequencing
Most novel diagnostics used in CNSI are pathogen-specific and therefore rely on the current paradigm of the treating clinician instigating investigations for a specific pathogen. In contrast, sequencing and identification of all nucleic acid in a clinical sample, termed metagenomic sequencing for DNA sequencing and metatranscriptomic sequencing for RNA, offers an unbiased, hypothesis-free diagnostic approach for diagnosing neuroinvasive pathogens which has been employed in resource-rich settings [Citation88,Citation89]. Data is now emerging from resource-limited settings where it has been used to detect pathogens that would have been missed through conventional diagnostic approaches [Citation90,Citation91].
The majority of metagenomic sequencing has been performed on short-read sequencing platforms manufactured by Illumina, focusing on DNA fragments of less than 250 bp. However, long-read sequencing technologies are now becoming more commonplace with sequencers manufactured by Oxford Nanopore Technologies (ONT) and Pacific Biosciences sequencing long fragments of DNA (1,000 to 100,000 base pairs) [Citation92]. ONT sequencers are of particular interest in resource-limited settings as they have potentially faster turnaround times, are easily transportable and the smaller sequencers have relatively low power consumption [Citation93]. While short read sequencing remains the highest throughput sequencing technology with the lowest error rate, recent improvements in long-read sequencing mean that there are now comparable error rates in the data of both [Citation94]. A previous advantage of short-read sequencing was the easy-to-use computational tools that were dedicated to the analysis of short read data only. However, pipelines such as Chan Zuckerberg ID (CZ ID) can now analyze data from ONT thereby simplifying the interpretation of the data and detection of pathogens, making this technology more accessible to countries without high-level analytical support [Citation95].
The cost of metagenomic sequencing remains prohibitively high for routine diagnostics in resource-limited settings. However it has fallen over recent years with the expansion of DNA sequencing technologies and will continue to do so. The COVID-19 pandemic, and external funding opportunities, also greatly increased the sequencing capacity in many resource-limited countries. Therefore, despite financial challenges, targeted use of metagenomic sequencing on CSF samples to identify rare or novel pathogens could be a crucial tool to inform routine PCR diagnostics and pathogen selection for multiplex panels.
9. Conclusion
Resource-limited settings are disproportionally affected by CNSI and prompt, accurate diagnosis is required to reduce morbidity and mortality. The diagnosis of CNSI in resource-limited settings is challenging due to an often poor understanding of regional epidemiology, high HIV-prevalence resulting in a broad differential and a reliance on traditional techniques that have poor sensitivity.
Molecular testing is widely used for CNSI diagnosis in resource-rich settings. Whilst these tests have markedly improved CNSI diagnosis certain tests have important limitations, such as the inability of currently available TB diagnostics to exclude TBM with a negative test. These need to be fully understood by clinicians to be successfully implemented.
The majority of molecular testing for CNSI in resource-limited settings is performed in trial settings and access outside of this is limited. This is mostly due to the cost of the platforms and cost-effectiveness data is lacking to support more widespread implementation. Cost-effectiveness data and involvement of policymakers is needed to identify how best these platforms can be used to improve the diagnosis of CNSI in resource-limited settings [Citation12].
10. Expert opinion
CNSIs remain a major cause of morbidity and mortality in resource-limited settings and improved diagnostic platforms are urgently needed to provide rapid actionable results that can inform immediate treatment decisions and improve outcomes. Technologies exist that could improve diagnosis of CNSI in resource-limited settings but many are not widely used. Whilst there are multiple challenges unique to resource-limited settings that prevent widespread rollout of novel and molecular diagnostics, there are a number of success stories that demonstrate it is feasible. The primary constraint to greater adoption in resource-limited settings is financial; optimal use of these platforms potentially lies in clinically- and cost-effective algorithms tailored to local CNSI epidemiology. One example of an algorithm that would be relevant to high HIV-prevalence settings would be initial CSF testing with CrAg, if this is negative then analysis with Xpert MTB/RIF Ultra, and multiplex PCR testing performed on those samples still without a diagnosis. Whilst selective testing of patients as part of a diagnostic pathway that stops once a positive result is received is cost-effective there is a potential for missed co-infections and further data are therefore needed to identify which patients could be safely excluded. If diagnostic algorithms for investigation of CNSI were introduced, more extensive testing at sentinel sites as part of surveillance networks would still be required to identify changes in local CNSI epidemiology that may impact the clinical effectiveness of diagnostic algorithms. These networks would also be able to provide important epidemiological data that could inform future multiplex PCR panel adjustments to allow inclusion of analytes of local epidemiological importance. For example, the addition of JCV and Toxoplasma gondii in high HIV-prevalence settings and M. tuberculosis in TB endemic areas to multiple PCR panels would likely increase their clinical utility and cost-effectiveness.
Significant advancements in CNSI diagnostics have been made, but a number of pathogen-specific investigations still remain imperfect. In particular, the diagnosis is TBM remains challenging. Whilst Xpert MTB/RIF Ultra represents a notable progression it cannot be used in isolation and is unable to exclude TB. As such continued research and development in new diagnostics is crucial to reduce mortality from CNSI worldwide.
Article highlights
Central nervous system infections continue to cause significant morbidity and mortality in low-resource settings with a substantial proportion of patients never receiving a confirmed diagnosis largely due to limitations in access to novel and molecular diagnostics. There is an urgent need to implement improved diagnostic platforms tailored to local epidemiology in resource-limited settings.
Multiplex PCR platforms have the potential to improve diagnostic capability in resource-limited settings and have been successfully implemented in laboratories with little to no experience of using molecular diagnostics but financial constraints often limit their use.
Tuberculous meningitis (TBM) is a leading cause of central nervous system infections in resource-limited settings however limitations in the diagnosis in TBM persist. Although Xpert MTB/RIF Ultra is a notable advancement in the diagnosis of TBM it remains insufficiently sensitive to exclude TBM.
Although metagenomic sequencing costs remain high they have decreased in recent years and the COVID-19 pandemic has resulted in significantly expanded sequencing capacity. Strategic, targeted use of metagenomic sequencing on CSF samples has the potential to identify rare or novel pathogens and guide analyte selection in multiplex PCR panels deployed in resource-limited settings.
Declaration of interest
J Milburn and JN Jarvis have received investigator-initiated funding from BioMérieux. The authors have no other relevant affiliations or financial involvement with any organization or entity with a financial interest in or financial conflict with the subject matter or materials discussed in the manuscript apart from those disclosed.
Reviewer disclosures
Peer reviewers on this manuscript have no relevant financial or other relationships to disclose.
Correction Statement
This article has been corrected with minor changes. These changes do not impact the academic content of the article.
Additional information
Funding
References
- Wunrow HY, Bender RG, Vongpradith A, et al. Global, regional, and national burden of meningitis and its aetiologies, 1990–2019: a systematic analysis for the global burden of disease study 2019. Lancet Neurol [Internet]. 2023 Aug;22(8):685–711. Available from: https://linkinghub.elsevier.com/retrieve/pii/S1474442223001953
- Wang H, Zhao S, Wang S, et al. Global magnitude of encephalitis burden and its evolving pattern over the past 30 years. J Infect. 2022 Jun 1;84(6):777–787. doi: 10.1016/j.jinf.2022.04.026
- Rajasingham R, Govender NP, Jordan A, et al. The global burden of HIV-associated cryptococcal infection in adults in 2020: a modelling analysis. Lancet Infect Dis [Internet]. 2022;22(12):1748–1755. doi: 10.1016/S1473-3099(22)00499-6
- Dodd PJ, Osman M, Cresswell FV, et al. The global burden of tuberculous meningitis in adults: a modelling study. PLoS Glob Public Health. 2021 Dec 8;1(12):e0000069. doi: 10.1371/journal.pgph.0000069
- Zunt JR, Kassebaum NJ, Blake N, et al. Global, regional, and national burden of meningitis, 1990–2016: a systematic analysis for the global burden of disease study 2016. Lancet Neurol. 2018 Dec 1;17(12):1061–1082. doi: 10.1016/S1474-4422(18)30387-9
- Sulaiman T, Salazar L, Hasbun R. Acute versus subacute community-acquired meningitis: analysis of 611 adult patients. Open Forum Infect Dis. 2016;3(suppl_1). doi: 10.1093/ofid/ofw172.878
- Tenforde MW, Mokomane M, Leeme T, et al. Epidemiology of adult meningitis during antiretroviral therapy scale-up in southern Africa: results from the Botswana national meningitis survey. J Infect. 2019;79(3):212–219. doi: 10.1016/j.jinf.2019.06.013
- Dittrich S, Rattanavong S, Lee SJ. et al. Orientia, rickettsia, and Leptospira pathogens as causes of CNS infections in Laos: a prospective study. Lancet Glob Health. 2015 Feb;3(2):e104–12
- Lingani C, Bergeron-Caron C, Stuart JM, et al. Meningococcal meningitis surveillance in the African meningitis belt, 2004–2013. Clin Infect Dis. 2015 Nov 15;61(suppl_5):S410–5. doi: 10.1093/cid/civ597
- Siddiqi OK, Ghebremichael M, Dang X, et al. Molecular diagnosis of central nervous system opportunistic infections in HIV-infected Zambian adults. Clinl Infect Dis. 2014 Jun 15;58(12):1771–1777. doi: 10.1093/cid/ciu191
- Ellis J, Bangdiwala AS, V CF, et al. The changing epidemiology of HIV-Associated adult meningitis, Uganda 2015–2017. Open Forum Infect Dis. 2019 Oct 1;6(10). doi: 10.1093/ofid/ofz419
- Tenforde MW, Gertz AM, Lawrence DS, et al. Mortality from HIV‐associated meningitis in sub‐Saharan Africa: a systematic review and meta‐analysis. J Int AIDS Soc. 2020 Jan;23(1). doi: 10.1002/jia2.25416
- DeBiasi RL, Tyler KL. Molecular methods for diagnosis of viral encephalitis. Clin Microbiol Rev. 2004;17(4):903–925. doi: 10.1128/CMR.17.4.903-925.2004
- McGill F, Heyderman RS, Michael BD, et al. The UK joint specialist societies guideline on the diagnosis and management of acute meningitis and meningococcal sepsis in immunocompetent adults. J Infect. 2016 Apr 1;72(4):405–438. doi: 10.1016/j.jinf.2016.01.007
- Karakousis A, Tan L, Ellis D. et al. An assessment of the efficiency of fungal DNA extraction methods for maximizing the detection of medically important fungi using PCR. J Microbiol Methods. 2006 Apr;65(1):38–48
- Bridge S, Huppler K, Nerima C, et al. Evaluation of the BioFire Ò FilmArray Ò Meningitis/Encephalitis panel in an adult and pediatric Ugandan population. J Med Mycol. 2021;31(3):101170. doi: 10.1016/j.mycmed.2021.101170
- Cresswell FV, Tugume L, Bahr NC, et al. Xpert MTB/RIF ultra for the diagnosis of HIV-associated tuberculous meningitis: a prospective validation study. Lancet Infect Dis. 2020 Mar 1;20(3):308–317. doi: 10.1016/S1473-3099(19)30550-X
- Scarborough M, Thwaites GE. The diagnosis and management of acute bacterial meningitis in resource-poor settings. Lancet Neurol. 2008;7(7):637–648. doi: 10.1016/S1474-4422(08)70139-X
- Jarvis JN, Meintjes G, Williams A, et al. Adult meningitis in a setting of high HIV and TB prevalence: findings from 4961 suspected cases. BMC Infect Dis. 2010;10(1):10. doi: 10.1186/1471-2334-10-67
- Tenforde MW, Meintjes G, Mokomane M, et al. Low-cerebrospinal fluid white cell counts and mortality in HIV-associated pneumococcal meningitis. AIDS. 2019 Jul 15;33(9):1539–1541.
- Tunkel AR, Hartman BJ, Kaplan SL, et al. Practice guidelines for the management of bacterial meningitis. Clinl Infect Dis. 2004;1October129(9):1267–1284. doi: 10.1086/425368
- Poplin V, Boulware DR, Bahr NC. Methods for rapid diagnosis of meningitis etiology in adults. Biomarker Med. 2020;14(6):459–479. doi: 10.2217/bmm-2019-0333
- Jarvis JN, Bicanic T, Loyse A. et al. Determinants of mortality in a combined cohort of 501 patients with HIV-associated cryptococcal meningitis: implications for improving outcomes. Clinl Infect Dis. 2014 Mar;58(5):736–745
- Bohr V, Rasmussen N, Hansen B. et al. 875 cases of bacterial meningitis: diagnostic procedures and the impact of preadmission antibiotic therapy part III of a three-part series. J Infect. 1983 Nov;7(3):193–202
- Kanegaye JT, Soliemanzadeh P, Bradley JS. Lumbar puncture in pediatric bacterial meningitis: defining the time interval for recovery of cerebrospinal fluid pathogens after parenteral antibiotic pretreatment. Pediatrics. 2001 Nov;108(5):1169–1174. doi: 10.1542/peds.108.5.1169
- Tschopp J, Brunel AS, Spertini O, et al. High false-positive rate of (1,3)-β-D-Glucan in onco-hematological patients receiving immunoglobulins and therapeutic antibodies. Clinl Infect Dis. 2022 Aug 25;75(2):330–333. doi: 10.1093/cid/ciab1028
- Kanamori H, Kanemitsu K, Miyasaka T, et al. Measurement of (1-3)-.BETA.-D-Glucan derived from different gauze types. Tohoku J Exp Med. 2009;217(2):117–121. doi: 10.1620/tjem.217.117
- Davis C, Wheat LJ, Myint T, et al. Efficacy of cerebrospinal fluid beta-D-glucan diagnostic testing for fungal meningitis: a systematic review. J Clin Microbiol [Internet]. 2020;58(4). doi: 10.1128/jcm.02094-19
- Forster J, Hoenigl M, Suerbaum S, et al. Serologic biomarkers in Candida and aspergillus infections of the central nervous system: a comparison of galactomannan, mannan and β-1,3-D-gucan testing from serum and cerebrospinal fluid. Mycoses. 2022 Jul 1;65(7):709–714.
- Song J, Kim SH, Jung YR, et al. 10-year retrospective review of the etiologies for meningitis with elevated adenosine deaminase in cerebrospinal fluid: etiologies other than TB. Front Cell Infect Microbiol. 2022 Jul 5;12. doi: 10.3389/fcimb.2022.858724
- Ekermans P, Dusé A, George J. The dubious value of cerebrospinal fluid adenosine deaminase measurement for the diagnosis of tuberculous meningitis. BMC Infect Dis. 2017 Dec 31;17(1):104.
- Mishra O. Cerebrospinal fluid adenosine deaminase activity for the diagnosis of tuberculous meningitis in children. J Trop Pediatr. 1996 Jun 1;42(3):129–132.
- Macrae C, Ellis J, Keddie SH, et al. Diagnostic performance of the IMMY cryptococcal antigen lateral flow assay on serum and cerebrospinal fluid for diagnosis of cryptococcosis in HIV-negative patients: a systematic review. BMC Infect Dis. 2023 Apr 6;23(1):209. doi: 10.1186/s12879-023-08135-w
- Temfack E, Rim JJB, Spijker R, et al. Cryptococcal antigen in serum and cerebrospinal fluid for detecting cryptococcal meningitis in adults living with human immunodeficiency virus: systematic review and meta-analysis of diagnostic test accuracy studies. Clinl Infect Dis. 2021 Apr 8;72(7):1268–1278. doi: 10.1093/cid/ciaa1243
- Tadeo KK, Nimwesiga A, Kwizera R, et al. Evaluation of the diagnostic performance of a semiquantitative cryptococcal antigen point-of-care assay among HIV-Infected persons with cryptococcal meningitis. J Clin Microbiol. 2021 Jul 1;59(8). doi: 10.1128/JCM.00860-21
- Feagins AR, Ronveaux O, Taha MK. et al. Next generation rapid diagnostic tests for meningitis diagnosis. J Infect. 2020 Nov;81(5):712–718
- Haddar C, Terrade A, Verhoeven P. et al. Validation of a new rapid detection test for detection of Neisseria meningitidis A/C/W/X/Y antigens in cerebrospinal fluid. J Clin Microbiol. 2020 Feb;58(3):e01699–19
- WHO. Meeting report of a technical expert consultation: non-inferiority analysis of xpert MTB/RIF ultra compared to xpert MTB/RIF [Internet]. 2017. Available from: http://apps.who.int/bookorders
- Heemskerk AD, Donovan J, Thu DDA, et al. Improving the microbiological diagnosis of tuberculous meningitis: A prospective, international, multicentre comparison of conventional and modified Ziehl–Neelsen stain, GeneXpert, and culture of cerebrospinal fluid. J Infect. 2018 Dec 1;77(6):509–515. doi: 10.1016/j.jinf.2018.09.003
- Seid G, Alemu A, Dagne B, et al. Microbiological diagnosis and mortality of tuberculosis meningitis: systematic review and meta-analysis. PLoS One. 2023 Feb 1;18(2):e0279203.
- Chen P, Shi M, Feng GD. et al. A highly efficient Ziehl-Neelsen stain: Identifying de novo intracellular Mycobacterium tuberculosis and improving detection of extracellular M. tuberculosis in cerebrospinal fluid. J Clin Microbiol. 2012 Apr;50(4):1166–1170
- Stadelman AM, Ssebambulidde K, Buller A, et al. Cerebrospinal fluid AFB smear in adults with tuberculous meningitis: a systematic review and diagnostic test accuracy meta-analysis. SSRN Electron J. 2021. doi: 10.2139/ssrn.3770634
- Donovan J, Thu DDA, Phu NH, et al. Xpert MTB/RIF ultra versus xpert MTB/RIF for the diagnosis of tuberculous meningitis: a prospective, randomised, diagnostic accuracy study. Lancet Infect Dis. 2020 Mar 1;20(3):299–307. doi: 10.1016/S1473-3099(19)30649-8
- Bahr NC, Nuwagira E, Evans EE, et al. Diagnostic accuracy of Xpert MTB/RIF Ultra for tuberculous meningitis in HIV-infected adults: a prospective cohort study. Lancet Infect Dis. 2018 Jan 1;18(1):68–75. doi: 10.1016/S1473-3099(17)30474-7
- Kohli M, Schiller I, Dendukuri N, et al. Xpert MTB/RIF ultra and xpert MTB/RIF assays for extrapulmonary tuberculosis and rifampicin resistance in adults. Cochrane Database Syst Rev. 2021 Jan 15;1(1):CD012768. doi: 10.1002/14651858.CD012768.pub3. PMID: 33448348; PMCID: PMC8078545.
- Donovan J, Cresswell FV, Thuong NTT, et al. Xpert MTB/RIF ultra for the diagnosis of tuberculous meningitis: a small step forward. Clin Infec Dis. 2020 Nov 5;71(8):2002–2005. doi: 10.1093/cid/ciaa473
- Broger T, Nicol MP, Szekely R, et al. Diagnostic accuracy of a novel tuberculosis point-of-care urine lipoarabinomannan assay for people living with HIV: a meta-analysis of individual in- a nd outpatient data. PLoS Med. 2020;17(5):1–16. doi: 10.1371/journal.pmed.1003113
- Siddiqi OK, Birbeck GL, Ghebremichael M, et al. Prospective cohort study on performance of cerebrospinal fluid (CSF) xpert MTB/RIF, CSF lipoarabinomannan (LAM) lateral flow assay (LFA), and urine LAM LFA for diagnosis of tuberculous meningitis in Zambia. J Clin Microbiol. 2019;57(8). doi: 10.1128/JCM.00652-19
- Quinn CM, Kagimu E, Okirworth M, et al. Fujifilm SILVAMP TB LAM assay on cerebrospinal fluid for the detection of tuberculous meningitis in adults with human immunodeficiency virus. Clinl Infect Dis. 2021;73(9):e3428–34. doi: 10.1093/cid/ciaa1910
- Sharma K, Sharma M, Modi M, et al. Comparative analysis of TruenatE MTB plus and XpertW Ultra in diagnosing tuberculous meningitis. Int J Tuberc Lung Dis. 2021 Aug 1;25(8):626–631. doi: 10.5588/ijtld.21.0156
- Bossolasco S, Calori G, Moretti F, et al. Prognostic significance of JC virus DNA levels in cerebrospinal fluid of patients with HIV-Associated progressive multifocal leukoencephalopathy [Internet]. Available from: https://academic.oup.com/cid/article/40/5/738/365769
- Nakamichi K, Kawamoto M, Ishii J. et al. Improving detection of JC virus by ultrafiltration of cerebrospinal fluid before polymerase chain reaction for the diagnosis of progressive multifocal leukoencephalopathy. BMC neurol. 2019 Oct 25;19(1). doi: 10.1186/s12883-019-1476-2
- Ferretti F, Bestetti A, Yiannoutsos CT, et al. Diagnostic and prognostic value of JC virus DNA in plasma in progressive multifocal leukoencephalopathy. Clinl Infect Dis. 2018 Jun 18;67(1):65–72. doi: 10.1093/cid/ciy030
- Tan IL, McArthur JC, Clifford DB, et al. Immune reconstitution inflammatory syndrome in natalizumab-associated PML. Neurology. 2011 Sep;77(11):1061–1067. doi: 10.1212/WNL.0b013e31822e55e7
- Berger JR, Aksamit AJ, Clifford DB, et al. VIEWS & REVIEWS PML diagnostic criteria consensus statement from the AAN neuroinfectious disease section [Internet]. 2013. Available from: www.neurology.org
- Miskin DP, Ngo LH, Koralnik IJ. Diagnostic delay in progressive multifocal leukoencephalopathy. Ann Clin Transl Neurol. 2016;3(5):386–391. doi: 10.1002/acn3.301
- Sharma SK, Soneja M, Ranjan S, et al. Progressive multifocal leucoencephalopathy in HIV/AIDS: observational study from a tertiary care centre in northern India. Indian J Med Res. 2013;138(JUL 2013):72–77.
- Arora S, Ahmad FMH, Deshwal R, et al. Study of clinical profile and outcomes in progressive multifocal leukoencephalopathy in acquired immunodeficiency syndrome patients in the highly active antiretroviral therapy era - an observational study. Indian J Sex Transm Dis AIDS. 2022;43(2):156–160. doi: 10.4103/ijstd.IJSTD_29_18
- Vidal JE, de OA, MCDS F, et al. Aids-related progressive multifocal leukoencephalopathy: a retrospective study in a referral center in São Paulo, Brazil. Rev Inst Med Trop Sao Paulo. 2008 Aug;50(4):209–212. doi: 10.1590/S0036-46652008000400004
- Schutte CM, Ranchhod N, Kakaza M, et al. AIDS-related progressive multifocal leukoencephalopathy (PML): a retrospective study from Pretoria, South Africa. S Afr Med J. 2013;103(6):399–401. doi: 10.7196/SAMJ.6386
- Sim BZ, Smith LK, Yarwood T, et al. Successful treatment of HIV-associated progressive multifocal leukoencephalopathy with pembrolizumab. AIDS. 2022 Mar 1;36(3):483–485.
- Safarpour H, Cevik M, Zarean M, et al. Global status of Toxoplasma gondii infection and associated risk factors in people living with HIV. AIDS. 2020 Mar 1;34(3):469–474. doi: 10.1097/QAD.0000000000002424
- Bokharaei-Salim F, Esteghamati A, Khanaliha K, et al. Evaluation of a PCR assay for diagnosis of toxoplasmosis in serum and peripheral blood mononuclear cell among HIV/AIDS patients. J Parasitic Dis. 2020 Mar 1;44(1):159–165. doi: 10.1007/s12639-019-01176-6
- Wang ZD, Wang SC, Liu HH, et al. Prevalence and burden of Toxoplasma gondii infection in HIV-infected people: a systematic review and meta-analysis. Lancet HIV. 2017;4(4):e177–88. doi: 10.1016/S2352-3018(17)30005-X
- Liu Q, Wang ZD, Huang SY, et al. Diagnosis of toxoplasmosis and typing of Toxoplasma gondii. Parasites Vectors. 2015 Dec 28;8(1):292. doi: 10.1186/s13071-015-0902-6
- Roux G, Varlet-Marie E, Bastien P, et al. Evolution of toxoplasma-PCR methods and practices: a French national survey and proposal for technical guidelines. Int J Parasitol. 2018;48(9–10):701–707. doi: 10.1016/j.ijpara.2018.03.011
- Wesołowski R, Pawłowska M, Smoguła M, et al. Advances and challenges in diagnostics of toxoplasmosis in HIV-Infected patients. Pathogens. 2023 Jan 9;12(1):110. doi: 10.3390/pathogens12010110
- Campbell GL, Hills SL, Fischer M, et al. Estimated global incidence of Japanese encephalitis: a systematic review. Bull World Health Organ. 2011;89(10):766–774. doi: 10.2471/BLT.10.085233
- Mackenzie JS, Williams DT, van den Hurk AF, et al. Japanese encephalitis virus: the emergence of genotype IV in Australia and its potential endemicity. Viruses. 2022 Nov 9;14(11):2480.
- Bharucha T, Sengvilaipaseuth O, Vongsouvath M, et al. Development of an improved RT-qPCR assay for detection of Japanese encephalitis virus (JEV) RNA including a systematic review and comprehensive comparison with published methods. PLoS One. 2018 Mar 1;13(3):e0194412. doi: 10.1371/journal.pone.0194412
- Bharucha T, Shearer FM, Vongsouvath M, et al. A need to raise the bar — A systematic review of temporal trends in diagnostics for Japanese encephalitis virus infection, and perspectives for future research. Inter J Infect Dis. 2020 Jun 1;95:444–456.
- Rowley J, Vander HS, Korenromp E, et al. Chlamydia, gonorrhoea, trichomoniasis and syphilis: global prevalence and incidence estimates, 2016. Bull World Health Organ. 2019 Aug 1;97(8):548–562P. doi: 10.2471/BLT.18.228486
- Marks M, Jarvis JN, Howlett W, et al. Neurosyphilis in Africa: a systematic review. PLoS Negl Trop Dis. 2017 Aug 1;11(8):e0005880. doi: 10.1371/journal.pntd.0005880
- de Voux A, Kidd S, Torrone EA. Reported cases of neurosyphilis among early syphilis cases—United States, 2009 to 2015. Sex Transm Dis. 2018 Jan;45(1):39–41. doi: 10.1097/OLQ.0000000000000687
- Ropper AH, Neurosyphilis, Longo DL editor, et al. Neurosyphilis. N Engl J Med [Internet]. 2019 Oct 3;381(14):1358–1363. doi: 10.1056/NEJMra1906228
- Marks M, Lawrence D, Kositz C. et al. Diagnostic performance of PCR assays for the diagnosis of neurosyphilis: a systematic review. Sex Transm Infect. 2018 Dec;94(8):585–588
- Gonzalez H, Koralnik IJ, Huhn GD, et al. A dual-platform point-of-care test for neurosyphilis diagnosis. Sex Transm Dis. 2021 May 1;48(5):353–356. doi: 10.1097/OLQ.0000000000001308
- Leber A, Everhart K, Balada-Llasat J, et al. Multi-center clinical evaluation of a multiplex Meningitis/Encephalitis PCR panel for simultaneous detection of bacteria, yeast, and viruses in cerebrospinal fluid specimens. J Clin Microbiol. 2016;54(9):2251–2261. doi: 10.1128/JCM.00730-16
- Tansarli GS, Chapin KC. Diagnostic test accuracy of the BioFire® FilmArray® meningitis/encephalitis panel: a systematic review and meta-analysis. Clin Microbiol Infect. 2020;26(3):281–290. doi: 10.1016/j.cmi.2019.11.016
- Humisto A, Antikainen J, Holma T, et al. Evaluation of the novel CE-IVD-Marked multiplex PCR QIAstat-dx Meningitis/Encephalitis panel. Microbiol Spectr. 2023;11(3):1–11. doi: 10.1128/spectrum.05144-22
- QIAGEN. Sample to insight QIAstat-dx ® Meningitis/Encephalitis (ME) panel instructions for use (handbook) 6. 2022.
- Rhein J, Bahr NC, Hemmert AC, et al. Diagnostic performance of a multiplex PCR assay for meningitis in an HIV-infected population in Uganda. Diagn Microbiol Infect Dis. 2016 Mar;84(3):268–273. doi: 10.1016/j.diagmicrobio.2015.11.017
- Bårnes GK, Gudina EK, Berhane M, et al. New molecular tools for meningitis diagnostics in Ethiopia - a necessary step towards improving antimicrobial prescription. BMC Infect Dis. 2018;18(1):1–14. doi: 10.1186/s12879-018-3589-4
- Debbagh F, Harrar S, Babokh F, et al. The contribution of multiplex polymerase chain reaction in the diagnosis of central nervous system infections in intensive care units. Cureus. 2023;15(2). doi: 10.7759/cureus.35338
- Tarai B, Das P. FilmArray® meningitis/encephalitis (ME) panel, a rapid molecular platform for diagnosis of CNS infections in a tertiary care hospital in North India: one-and-half-year review. Neurol Sci. 2019;40(1):81–88. doi: 10.1007/s10072-018-3584-y
- Peñata A, Mesa S, Leal A, et al. Molecular diagnosis of meningitis and meningoencephalitis with an automated real-time multiplex polymerase chain reaction in a tertiary reference complex in medellín, Colombia. Rev Inst Med Trop Sao Paulo. 2020;62:e77. doi: 10.1590/s1678-9946202062077
- Brisset J, Angendu Baki K, Watier L, et al. Non-traumatic coma in young children in Benin: are viral and bacterial infections gaining ground on cerebral malaria? Infect Dis Poverty. 2022;11(1):1–10. doi: 10.1186/s40249-022-00956-2
- Simner PJ, Miller S, Carroll KC. Understanding the promises and hurdles of metagenomic next-generation sequencing as a diagnostic tool for infectious diseases. Clinl Infect Dis. 2018;66(5):778–788. doi: 10.1093/cid/cix881
- Wilson MR, Sample HA, Zorn KC, et al. Clinical metagenomic sequencing for diagnosis of meningitis and encephalitis. N Engl J Med. 2019;380(24):2327–2340. doi: 10.1056/NEJMoa1803396
- Hong NTT, Anh NT, Mai NTH, et al. Performance of metagenomic next-generation sequencing for the diagnosis of viral meningoencephalitis in a resource-limited setting. Open Forum Infect Dis. 2020;7(3):1–10. doi: 10.1093/ofid/ofaa046
- Ramachandran PS, Ramesh A, Creswell FV, et al. Integrating central nervous system metagenomics and host response for diagnosis of tuberculosis meningitis and its mimics. Nat Commun. 2022;13(1):1–12. doi: 10.1038/s41467-022-29353-x
- Houlihan CF, Bharucha T, Breuer J. Advances in molecular diagnostic testing for central nervous system infections. Curr Opin Infect Dis. 2019;32(3):244–250. doi: 10.1097/QCO.0000000000000548
- Hong NTT, Anh NT, Mai NTH, et al. Performance of metagenomic next-generation sequencing for the diagnosis of viral meningoencephalitis in a resource-limited setting. Open Forum Infect Dis. 2020 Mar 1;7(3). doi: 10.1093/ofid/ofaa046
- Wick RR, Judd LM, Holt KE, et al. Assembling the perfect bacterial genome using oxford nanopore and Illumina sequencing. PLoS Comput Biol. 2023 Mar 1;19(3):e1010905. doi: 10.1371/journal.pcbi.1010905
- Hu T, Chitnis N, Monos D, et al. Next-generation sequencing technologies: an overview. Hum Immunol. 2021;82(11):801–811. doi: 10.1016/j.humimm.2021.02.012