ABSTRACT
Introduction
Functional neurological disorder (FND) is a neuropsychiatric disorder that manifests in a broad array of functional motor, sensory, or cognitive symptoms, which arise from complex interactions between brain, mind, body, and context. Children with FND make up 10%–20% of presentations to neurology services in children’s hospitals and up to 20% of adolescents admitted to hospital for the management of intractable seizures.
Areas Covered
The current review focuses on the neurobiology of pediatric FND. The authors present an overview of the small but growing body of research pertaining to the biological, emotion-processing, cognitive, mental health, physical health, and social system levels.
Expert opinion
Emerging research suggests that pediatric FND is underpinned by aberrant changes within and between neuron-glial (brain) networks, with a variety of factors – on multiple system levels – contributing to brain network changes. In pediatric practice, adverse childhood experiences (ACEs) are commonly reported, and activation or dysregulation of stress-system components is a frequent finding. Our growing understanding of the neurobiology of pediatric FND has yielded important flow-on effects for assessing and diagnosing FND, for developing targeted treatment interventions, and for improving the treatment outcomes of children and adolescents with FND.
1. Introduction
Functional neurological disorder (FND) is a neuropsychiatric disorder involving aberrant changes within and between neuron-glial (brain) networks as well as complex interactions between brain, mind, body, and context – the lived experience of the child and the family. Children (including adolescents) with FND present with a broad range of functional neurological symptoms: motor symptoms that affect the limbs (weakness or loss of function, tics, tremors, unusual gaits, dystonia); functional seizures (including syncope-like events); loss of sensory function (loss of hearing, vision, or sense of touch); dizziness and balance difficulties; motor symptoms affecting the voice (aphonia, dysphonia), digestive tract (globus sensation, difficulty swallowing, rumination), or bladder (urinary retention, irritable bladder); and changes in cognitive function or consciousness. In the hospital setting, presentations with FND are common. Children with FND make up to 10% of children presenting to pediatric general neurology clinics [Citation1], up to 20% of children presenting to epilepsy clinics [Citation2], and up to 20% of adolescents admitted to hospital for the management of suspected (convulsive) status epilepticus [Citation3]. Population-based studies suggest that the incidence and prevalence of functional symptoms peak in adolescence and early adulthood [Citation4–6]. In civilian settings girls present more frequently than boys [Citation4,Citation6–9]. As children in our society become more stressed – in the context of cumulative natural disasters and increasing academic pressures, political tensions, and eco stress – presentations with functional somatic symptoms are likely to increase (see Box 1).
Box 1. The Relationship Between Sociocultural Stress and FND.
In his celebrated book – Psychiatry Around the Globe: A Transcultural View – psychiatrist Julian Leff stated that the incidence of functional somatic symptoms (then hysteria) had declined in Western cultures because patients in the West, during the twentieth century, had gradually become more adept at expressing underlying conflicts and anxieties in psychological terms – that is, via language [Citation10]. Leff contrasted this development to more primitive societies where individuals gave expression to emotional disorders in somatic terms.
Leff’s work assessed the situation in England and Wales in 1949–78, a post-WWII period with a window of stability, safety, and increasing socioeconomic prosperity. If we look at the century that came before, however, we see that periods of war or sociocultural stress saw a rise in the incidence of functional somatic symptoms (including FND): the French Revolution (Cardiorespiratory Neurosis, Idiotism, and Syndrome du Vent du Boulet [syndrome of the wind of the cannon ball]); American Civil War (Irritable Heart or Da Costa’s Syndrome); Russian-Japanese War (War Neurosis); World War I (Shell Shock and Hypnose des batailles [battle hypnosis]) [Citation9,Citation11–14]. Akin to war settings, in high-risk occupations that engage in combat – such as the military or police – the occurrence of functional neurological symptoms (tunnel vision and changes in auditory processing) during states of high arousal, coupled with changes in pain processing (analgesia) and threat-related autonomic responses (expulsion of urine or feces) [Citation15], are common occurrences and are the subject of the training process [Citation16].
More recently, we have seen the relationship between sociocultural stress and incidence of FND played out during the COVID pandemic. In particular, during this period we have seen, worldwide, a sharp increase in the presentation of adolescent girls with functional tics [Citation17–26]. The increase in pediatric presentations with FND symptoms following the Boston Marathon Bombings is another case in point [Citation27].
FND is a clinical diagnosis. The clinician – usually a neurologist or pediatrician – utilizes the neurological examination to identify positive (rule-in) neurological signs of FND, and makes a positive diagnosis of FND (see ) [Citation28–30]. The message given to the child and family during this diagnostic process is that functional neurological symptoms have their own underlying neurobiology and that this neurobiology manifests in identifiable neurological signs that are elicited during the neurological examination.
Table 1. Common positive (rule-in) neurological signs found on physical examination in children with functional motor and sensory Symptomsa.
An important subtype of FND is functional seizures (FS). In most Western countries the gold standard for the diagnosis of FS is the video electroencephalogram (vEEG), in which the seizure events seen on the video are not accompanied by a wave-and-spike pattern on the EEG [Citation31]. In practice, however, vEEG is not always available. In this scenario neurologists who have clinical expertise in FS utilize clinical history, witnessed descriptions, home videos [Citation32,Citation33], and their knowledge of clinical signs that may accompany FS (see ) [Citation34–40] — usually accompanied by a routine EEG if available — to make a clinical diagnosis of FS.
Table 2. Clinically useful neurological signs for diagnosis of functional seizures.
The current review aims to summarize key advances in our understanding of the neurobiology of pediatric FND – based on research during the last two decades – and also the implications of these advances for the treatment of children and adolescents. Four overarching themes or frameworks shape this review.
First, because FND involves complex interactions between brain, mind, body, and context – the lived experience of the child and the family – we use systems (biopsychosocial) thinking and the associated levels of organization as an overarching structure. Each of the main headings within the review reflects a distinct system level. Systems thinking highlights that living systems have a tendency to form multileveled structures of systems within systems. At each level of organization – or system level – ‘the observed phenomena exhibit properties that do not exist at the lower level’ (p 28) [Citation41]. And although the information from different levels of organization is unique, it is neither more nor less fundamental than information from another level. Levels of organization are interrelated and interconnected. In the case of FND, the emergence of the symptoms is hypothesized to emerge from complex interactions within and between multiple system levels. As in any open system, a diversity of pathways may lead to the same outcome (called equifinality).
Second, we draw on the suggestion from evolutionary neuroscience that the brain’s functional networks are organized along a phylogenetic hierarchy that reflects stages in development [Citation42–45]. According to this framework each functional network corresponds to a particular level of arousal and reflects a distinct way of solving adaptive challenges [Citation44–46]. Networks mediating advanced processes – such as higher-order cognitive functions – become operational with maturation, involve activation of cortical brain regions, and function best when the child is calm and finds him- or herself in a safe context. By contrast, networks mediating reflexive modes of behavior – innate defensive functions that include energy regulation, autonomic activity, respiratory rhythms, processing of homeostatic and pain afferents, and activation of innate fear responses and other automatic behaviors – become operational when the child is in a state of physiological arousal. These reflexive modes of behavior involve activation of subcortical and limbic structures, phylogenetically older systems that especially likely to activate when the child finds him- or herself to be in a situation (real or perceived) that exceeds the child’s capacity to cope. The contemporary training of combat troops takes into account and makes use of these older, automatic systems that are activated by high arousal [Citation16]. Training drills serve to establish patterns of automatic behavior, enabling the trainee to function on automatic pilot during periods of high arousal when conscious thought is not possible and when the outcome likely depends on enactment of in-drilled motor responses (rather than motor responses such as motor paralysis that, though automatic, are not organized and, indeed, potentially fatal).
Third, we give central attention to stress, the stress system, and the impact of stress on body and brain systems. The stress system – encompassing the circadian clock, autonomic nervous system, inflammatory/immune system, HPA axis, and stress systems within the brain – plays a crucial role in homeostatic regulation, brain-body responses to threat, and the biological embedding of experience [Citation47,Citation48] (see ). While time-limited stress-system activation is advantageous for adaptation and survival, chronic activation or dysregulation is disadvantageous. Recurrent activation of the stress system in relation to early-life stress – particularly when the stress is cumulative or exceeds the child’s adaptive capacity – may oversensitize or undersensitize neuroendocrine responses to stress, leading to a dysregulated stress system over the life span [Citation47–51] and to an increased risk of physical, functional, and mental health disorders later in life [Citation52]. Importantly, children vary in their sensitivity to early-life stress, and this variation is influenced by interactions among all of the following: genetic/epigenetic factors; family, community, and school environments; and developmental timing [Citation53].
Figure 1. Circles metaphor of the stress-system model for functional somatic symptoms (including FND).
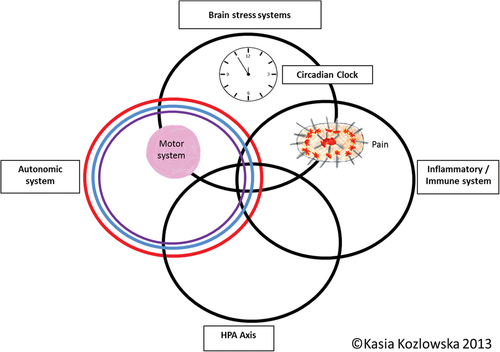
In the broader literature, the processes, including those mediated by the stress system, that enable a biological system to ‘meet perceived and anticipated demand’ (p. 646) while maintaining itself within parameters compatible with life are referred to as allostasis [Citation54]. Allostasis thus enables an organism to maintain ‘stability through change’ (p. 636) [Citation54]. Allostatic load refers to the biological cost of allostatic processes [Citation55]. As noted above, while time-limited stress-system activation is advantageous for adaptation and survival, chronic stress-system activation increases that system’s level of baseline activation, resulting in the ongoing, increased use of energy. The cumulative effect is ‘the loss of physiological coherence: the easy flow of life processes, harmony between body systems, and efficient utilization of energy’ (p. 242) [Citation56,Citation57].
Fourth, we draw upon the concepualization – from contemporary neuroscience – of the mind and brain as a generative system that models the world via prediction [Citation58–60]. From this perspective, ‘the brain has a model of the body and world’ (p. 544) that allows it to compare incoming information with existing information from past experience [Citation58–60]. ‘Feedback signals that do not match predictive coding create prediction error, which modifies the model so that predictive coding matches subsequent feedback’ (p. 544) [Citation58]. The brain’s predictive model generates predictions on multiple system levels of the biological system: implicit expectations (e.g. pertaining to energy needs); explicit expectations pertaining to physical sensations or symptoms (e.g. pain, sensation, and movement); explicit expectations pertaining to other elements of the self (e.g. felt sense of mastery); and explicit expectations pertaining to the world at large. States of high arousal affect predictive coding by prioritizing threat-related predictions and reflexive modes of behavior. Focus-of-attention has the effect of amplifying expectations – positive or negative – which, in turn, realigns the model itself. The interplay between predictive coding, arousal, and the child’s focus-of-attention contributes to the manner in which information from multiple sources is integrated within and across neural networks. ‘In functional neurological disorder, it is hypothesized that prediction error is not accurately updated, perpetuating dysfunction’ (p. 544) [Citation58].
On a final note, the focus of the current review is specifically on the neurobiology of FND as defined by DSM-5-TR [Citation61]. In this context, research studies of the following disorders frequently comorbid with FND are not included: chronic complex pain (most commonly, headache) [Citation62–65], functional abdominal pain, pain presentations in other parts of the body, and functional gut presentations (also known as disorders of brain-gut interaction) [Citation66–68]. Given their comorbidity with FND, it is to be expected that these various conditions have overlapping risk factors and neurobiological processes with FND (see conclusion). Finally, readers looking to develop a broader understanding of FND will potentially want to consult recent articles pertaining to evidence-based treatments for FND [Citation69,Citation70], including treatment approaches developed for the subgroup of children with FS [Citation71–74]. Also of note in this context are articles drawing attention to the need to address stigma regarding FND and to change the associated culture of care [Citation75,Citation76].
2. The biological system level: the somatic narrative
In this section we describe key research findings in children with FND on the biological system level. When talking to children and families, the clinician can refer to this system level as the somatic narrative [Citation75]. The somatic narrative refers to the ‘the language of the body and the story it is telling about what is happening to the child’ (p8) [Citation75]. As the reader will see, the child’s FND symptoms – and comorbid nonspecific functional symptoms (e.g. pain and fatigue) – reflect a multitude of neurophysiological processes. The ‘somatic narrative provides the pediatrician with valuable information about changes in the child’s body function in response to stress, threat, or danger, whether actual or perceived’ (p8) [Citation75]. Many of the advances on this biological system level have been made possible by the advent of new technologies and by looking at biological markers on a group-level of analysis – that is, the findings in a group of children with FND compared to healthy controls.
2.1. Children with FND present in a state of increased activation
A solid body of evidence shows that children with FND present in a state of increased activation. This state of activation appears to be most marked in children with FS.
Activation of the autonomic nervous system is associated with an increase in body arousal. In the heart – which is activated by the autonomic nervous system – the sinoatrial node sets a heart rate of about 100 bpm. Activation of the restorative parasympathetic component of the autonomic system (known as the parasympathetic [vagal] break) decreases heart rate, and the sympathetic component increases heart rate. Children with FND show a withdrawal of restorative (tonic) parasympathetic activity (lower heart rate variability and higher heart rates [up to 100 beats per minute]), sometimes an increase in sympathetic activity (higher heart rates [over the 100 beats per minute set by the sinoatrial node), and increased skin conductance due to increased sweating [Citation77–80]. A visual representation of autonomic data from our research group [Citation77,Citation81] can be found Savage and colleagues (2022), Appendix A, p197 [Citation71]. Clinically, autonomic arousal presents as a shift in heart rate to the right on the normative range. For example, the resting-state heart rate of an athletic adolescent, usually at 70 beats per minute or below, may be in the 80s or 90s, on the higher end of the normative curve. The child may also present with comorbid functional somatic symptoms that reflect activation or dysregulation of the autonomic nervous system (see ) [Citation82].
Figure 2. Common signs and symptoms associated with increased arousal © Kasia Kozlowska 2019, reproduced with permission.
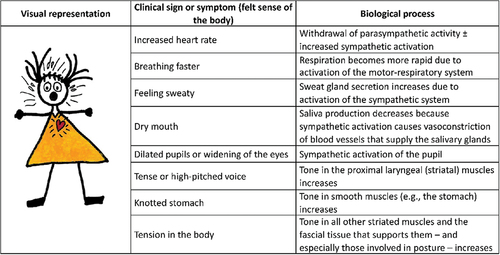
Because the autonomic and respiratory motor systems are coupled systems [Citation83], sympathetic arousal can be accompanied by an increase in respiratory rate. When the respiratory rate exceeds metabolic demand, it is called hyperventilation. The biomarker of hyperventilation is a low arterial carbon dioxide and an accompanying respiratory alkalosis. In a substantial proportion of children with FS, their seizure events are triggered by hyperventilation [Citation79,Citation82].
In the early stage of hyperventilation, the child’s cortical arousal increases – and with it, the arousal of neuron-glial networks [Citation84]. If the child’s hyperventilation continues, it induces respiratory alkalosis, vasoconstriction of cerebral and cardiac arteries, and a state of hypoxia that may contribute to changes in consciousness.
Clinically, hyperventilation presents as an increased respiratory rate (a shift to the right of the normative curve) or as respiratory rates outside of normative ranges [Citation85,Citation86] coupled with central or peripheral symptoms () [Citation45,Citation82,Citation87–94]. For children ≥6 years of age, respiratory rates > 25 breaths/minute are in the ≥ 97.5th centile, and respiratory rates > 30 breaths/minute are in the ≥ 99.9th centile [Citation85,Citation86]. In the clinic it can be helpful to point out to the child and family that the child’s high breathing rate is one way that the body communicates a state of increased activation. The increased breathing rate is part of the somatic narrative – the language of the body.
Table 3. Functional somatic symptoms caused by hyperventilation and the associated low PCO2 and respiratory alkalosis.
In sum, excessive activation of the autonomic nervous system and respiratory motor system can underpin a broad range of functional somatic symptoms and may contribute to cortical arousal and changes in consciousness (see and ). Comorbid functional somatic symptoms – some of which reflect the clinical signs of autonomic system activation and hyperventilation – are a recurrent finding in pediatric FND [Citation77,Citation95,Citation96]. Ongoing activation also consumes excess energy and is likely to contribute to the homeostatic emotion of fatigue.
2.2. Warning signs that precede functional seizures
In a prospective study of children with FS (n = 60; 70% girls), the mind-body team led by the first author highlighted the importance of paying attention to the warning signs that precede a FS: the symptoms and subjective sense of activation experienced by the child prior to the onset of an FS [Citation97,Citation98]. Warning signs are also referred to as prodromal FS symptoms or premonitory symptoms (see below) [Citation73,Citation79]. In the above study we also highlighted the importance of helping children notice their warning signs, with the consequence that interventions that calmed the brain and the body could be implemented – immediately in the here and now – to avert the onset of the FS. Most warning signs experienced by the child involve activation of the autonomic system (see ) and respiratory motor system (and associated cortical arousal and symptoms of hyperventilation) (see ), as well as activation of postural muscles that lie in the midline and that activate in the context of increased arousal. These midline muscles can yield a sense of tightness or pain in the body or head, and can activate the pain system (yielding headache, neckache, or back pain). Comorbid pain is a common nonspecific somatic symptom reported across studies [Citation77,Citation95,Citation96].
Building on this work, Sawchuk and colleagues (2020) found in their study of children with FS that over half of the 33 participants (n = 18; 55%) reported the subjective experience of prodromal FS symptoms during a hyperventilation task (while their percutaneous carbon dioxide [pCO2] levels were below 30 mm Hg) [Citation79]. A large majority of patients (n = 25; 89%) were able to correct their pCO2 values (to above 30 mm Hg) following a 10- to 20-minute period of biofeedback training with the assistance of a trained clinician. The biofeedback intervention provided them with a strategy for averting their FS.
Along the same lines, Stager and colleagues (2022) found in their study of children with FS that almost all of the 42 participants (n = 41; 98%) reported at least one premonitory symptom (average = 3.9 symptoms) signifying an impending FS [Citation73]. The most common premonitory symptom was headache (57%). Many of the other symptoms reflected autonomic system activation (nausea, increased heart rate, or temperature change [hot or cold]) or common central or peripheral symptoms of hyperventilation (blurry/double vision, stiffness, aura, dizziness/disorientation, or numbness).
2.3. Dysregulation of the HPA axis and circadian rhythm
Like many body processes, cortisol levels follow a circadian rhythm. They reach peak values within approximately 30 – 45 minutes of wakening [Citation99] – termed the cortisol awakening response (CAR) – and then gradually decline over the day and night, reaching the lowest levels in the early morning hours. The stability of the CAR is well established in healthy children and adolescents [Citation100,Citation101]. Exposure to acute stress is generally associated with an increase in CAR, and chronic, prolonged, uncontrollable, or traumatic stress is associated with an attenuation of CAR [Citation102].
In a study of children with a mix of FND symptoms (mixed FND, n = 32; 26 girls) characterized by high levels of functional impairment and admission to the Mind-Body Program [Citation70], healthy controls showed the expected increase in CAR, and children with FND showed a decrease [Citation103]. Within the FND group, 57% (n = 18) showed an attenuated CAR, and 43% (n = 14) showed an obliterated/reversed CAR (for a visual representation of the data see Savage and colleagues (2022), Appendix A, p196) [Citation71].
In controls, CAR showed no correlation with adverse childhood experiences (ACEs) and a positive correlation with subjective distress (total Depression Anxiety and Stress Scale score). This means that controls who were more distressed showed a greater rate of cortisol increase on waking (as expected in health and well-being). In the FND group, CAR was negatively correlated with ACEs and subjective distress. This means that FND participants who had a higher number of ACEs and who were more distressed had a more attenuated (or reversed) CAR. They were unable to mount a healthy cortisol response on awakening.
In sum, this group of children with mixed FND showed an abnormal CAR, reflecting dysregulation of the HPA axis. Based on the broader literature, one can hypothesize that their HPA axes had been activated recurrently in the context of cumulative stress (see introduction) [Citation47–51], yielding three implications for current health and well-being:
Attenuation of HPA-axis function prevents the child from mounting an adequate HPA response to current stress.
Past repeated activation of the HPA axis may have supported epigenetic programing processes – in which cortisol (a glucocorticoid) plays a key role – that sensitized various components of the stress system in the body and the brain to respond to subsequent stressors with an exaggerated response (autonomic system, immune-inflammatory system, pain system, and glial cells in the brain).
HPA dysregulation affects energy regulation, likely contributing to the homeostatic emotion of fatigue, a common comorbid symptom in children with FND.
2.4. Activation of the immune-inflammatory system
C-reactive protein (CRP) is a systemic marker of inflammation. Exposure to ACEs is known to contribute to a pro-inflammatory state [Citation104,Citation105]. A study looking at CRP in children with mixed FND (n = 79; 57 girls) found an upward shift in the distribution of CRP levels, with 66% of subjects (n = 52) having CRP titers ≥2 mg/L, consistent with a state of low-grade inflammation [Citation106]. Confounder analyses suggested that a history of maltreatment and a diagnosis of anxiety contributed to elevated CRP levels.
2.5. Electroencephalogram studies show increased brain arousal/activation, changes in connectivity in the resting state, and changes in brain connectivity during a functional seizure
In the brain, activation of neuron-glial networks can be measured using EEG technology. This methodology allows researchers to look at changes across five power bands: two slow-wave power bands (delta and theta) and three fast-wave power bands (alpha, beta, and gamma).
One study looked at EEG-derived markers in the resting state in adolescents with FS (n = 15) [Citation107]. Gamma power was higher in the left parietal regions and lower in the right temporal region (suggesting a loss of balance between the regions). In addition, decreased gamma band current source density was found in the right posterior parietal cortex, posterior cingulate cortex, and superior temporal gyrus. Connectivity analysis showed intracortical lagged coherence between right posterior cingulate gyrus and right middle temporal gyrus (trend level only) – that is, a disruption of normal connectivity. Taken together, the results suggested that in the resting state, the neuron-glial networks of adolescents with FS, compared to controls, shifts away from a healthy state, making the adolescents more prone to periods of dysregulation (manifesting as FS).
Another study, this time in children with mixed FND (n = 57; 72% girls) had excessive theta and delta power in electrode clusters corresponding to the default mode network, both anteriorly (dorsomedial prefrontal cortex) and posteriorly (posterior cingulate cortex, precuneus, and lateral parietal cortex) [Citation108]. They also had excessive theta and delta power in the premotor region/supplementary motor area, along with a trend toward increased theta and delta power in the salience network. LORETA – an analysis that tries to identify the source of the activity – localized neural sources to the dorsal anterior cingulate cortex/dorsomedial prefrontal cortex, mid cingulate cortex, posterior cingulate cortex/precuneus, and supplementary motor area. Importantly, all these regions have been implicated in the neurobiology of FND in adult imaging studies [Citation109–111]. Pain and arousal contributed to slow-wave power increases in all three networks, suggesting that all these processes – arousal, pain, and aberrant motor-sensory changes – are interrelated.
Yet another EEG study (n = 66; 71% girls) used a different mathematical approach called graph theory to study children in the resting state (i.e. while they were sitting quietly) [Citation112]. The children showed increases in gamma power, a high-frequency band that had not been looked at in the previous study [Citation108]. The increase in gamma power was greatest in children with FS, and it correlated with autonomic arousal. That is, the increase in body arousal in children with FS was accompanied by increased arousal in the brain (indexed by gamma power).
Task-based studies using the EEG also show that children with FND respond to stress stimuli with greater cortical arousal – an exaggerated response. One study with children with mixed FND (n = 41; 72% girls) used an auditory stressor: a tone of different pitch, duration, or loudness in the midst of a continuous series of identical tones at a relatively slow rate [Citation113]. Another study used hyperventilation as a stressor because hyperventilation is known to increase cortical arousal (see subsection above on hyperventilation) [Citation84]. The researchers in this study showed that, as expected, healthy children, children with epilepsy, and children with FS (n = 26, 77% girls) all demonstrated an increase in high-frequency power (the beta band) when they hyperventilated. But it was only children with FS who, once they were instructed to stop hyperventilating, demonstrated continued and sustained activation in the beta band. The brains of the other children returned to base-line function. In other words, when children with FS activate their brain networks via hyperventilation, these networks stay activated for a prolonged period (see ).
Figure 3. Sustained beta activation following hyperventilation in children with functional seizures.
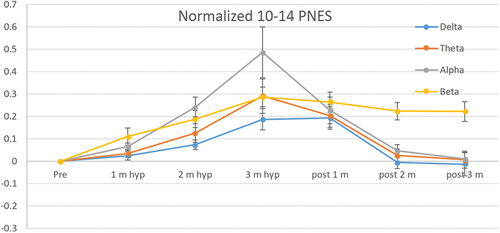
Because high-frequency power bands involve a high use of energy, the first author and colleagues have hypothesized that the brain’s energy-production systems are likely to be challenged to produce sufficient energy to maintain this state of neuron-glial network activation [Citation112]. We have also hypothesized that FS potentially reflected a neuron-glial network destabilization that occurs in response to ‘perturbations in energy flow or additional demands in the context of new threats, stress, or perceived threats or stress’ (p 14) [Citation112]. Put simply, we have suggested that brain networks disorganize (disconnect), resulting in an FS, when the brain is unable to provide sufficient energy to sustain this high level of activation. Under this hypothesis, epileptic seizures are the result of aberrant electrical activity; hypoxic seizures are the result of a loss of oxygen; and FS are the result of excessive energy demands in a system that is already overactivated at baseline.
A French research team examined the issue of neuron-glial network destabilization during FS directly using stereotactic EEG with two patients [Citation114]. Both patients in the study — one aged 19 and one aged 37 – suffered from childhood-onset, drug-resistant epilepsy comorbid with FS. The younger patient had a history of physical and psychological abuse at home, and the older had emigrated to France during the Kosovo War in 1999. The research team calculated global functional connectivity – background state, during an epileptic seizure, and during an FS – by computing pair-wise nonlinear correlations between signals from seven brain areas: amygdala, hippocampus, lateral temporal cortex, anterior insula, orbitofrontal cortex, prefrontal cortex, and lateral parietal cortex. They found that, compared to background activity, functional connectivity decreased globally during FS episodes – mostly affecting anterior insula and lateral parietal cortex (especially with orbitofrontal cortex, hippocampus, and prefrontal cortex) (see ). The functional connectivity profiles of the FS and the epileptic seizures were also significantly different.
Figure 4. Visual representation of the changes in connectivity in the resting state and during a functional seizure.
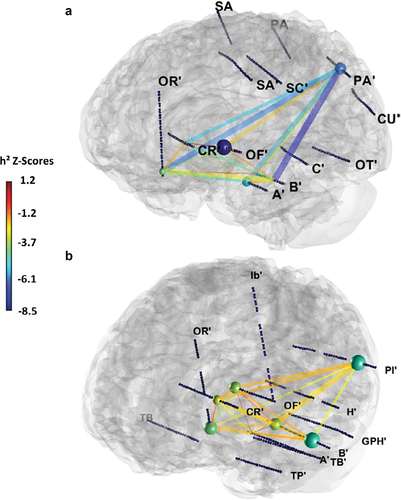
2.6. Magnetic resonance imaging studies suggest plasticity changes in the brain and aberrant change in brain networks
In daily clinical practice, brain-imaging studies are commonly obtained as part of the medical workup for children presenting with FND. The studies are typically reported as normal, or they show incidental findings unrelated to the child’s FND presentation. To look for structural or functional changes, researchers need to use high-resolution magnetic resonance imaging (MRI), collect a larger number of studies, and compare group results to those of healthy children matched for age and sex. A challenge for this sort of research is that children find it difficult to stay still in the MRI machine for any prolonged period. Consequently, few pediatric imaging studies have been completed.
In one such study – looking at brain structure in children with mixed FND (n = 25; 80% girls) – patients showed greater volumes in the left supplementary motor area and right superior temporal gyrus and dorsomedial prefrontal cortex [Citation115]. Greater supplementary motor area volumes correlated with faster reaction times in identifying emotions but not with clinical measures. As noted before, the dorsomedial prefrontal cortex and supplementary motor area have also been implicated in FND in adult studies [Citation109–111]. They (and also the superior temporal gyrus) are known to be involved in the perception of emotion and the modulation of motor responses. Based on the correlation analyses, our research team suggested that these larger volumes may reflect the early expression of an experience-dependent plasticity process associated with increased vigilance to others’ emotional states and also with enhanced motor readiness to organize self-protectively in the context of the long-standing relational stress that is characteristic of this disorder.
Another study, in a different group of children with mixed FND (n = 31; 77% girls) used a recently developed methodology – called whole-brain independent component analysis – to look at resting-state connectivity within and between neuron-glial (brain) networks [Citation95]. Children with FND showed wide-ranging connectivity changes in eight neuron-glial networks: language networks (IC6 and IC1), visual network, frontoparietal network, salience network, dorsal attention network, cerebellar network, and sensorimotor network. The subgroup of children with FS (vs. children with other FND symptoms) showed greater connectivity decreases in the frontoparietal and dorsal attentional networks. Children with FND (vs. controls) reported more subjective distress (total Depression Anxiety and Stress Scale score) and more ACEs across their life spans. Subjective distress (total Depression Anxiety and Stress Scale score), autonomic arousal (indexed by heart rate), and HPA dysregulation (attenuated/reversed CAR) contributed to changes in neural network connectivity.
2.7. Spectroscopy
One published pediatric study has used in vivo magnetic resonance spectroscopy (MRS) to investigate neurometabolic homeostasis in children with mixed FND (vs. healthy controls) in three regions of interest: supplementary motor area (SMA), anterior default mode network (aDMN), and posterior default mode network (dDMN) [Citation116]. Metabolites were referenced to creatinine, in line with adult studies. Multiple differences in neurometabolites were found. N-acetyl aspartate (NAA) – a marker of neural function – was lower in the SMA and pDMN. Myo-inositol (mI) – a marker of glial cell function – was lower (trend level) in the SMA and pDMN. Increased arousal (indexed by higher heart rate) was associated with lower mI in both regions (SMA and pDMN). γ-aminobutyric acid (GABA) – an inhibitor neurometabolite that is a marker of inhibitor neurotransmission – was lower in the SMA. Children with FS showed dysregulation (lowering) in excitatory/inhibitory balance – the relationship between excitatory neurometabolites (glutamate and glutamine) and GABA, in the aDMN. These findings suggest that glial cells work alongside neurons – via glia cell signaling and intercellular signaling in neuron-glia networks – to mediate neurometabolic alterations that contribute to brain network changes in FND. These findings suggest a need for a broader research lens, one that conceptualizes glial cells as key players within neuron-glia networks, thereby opening a door to further research and new treatment options. For example, the finding of lower mI – a marker of glial cell function – raises the intriguing possibility of using mI supplementation for the subgroup of patients with FND who trigger their FS by hyperventilation (a panic-like event, see section 2.1). Two randomized, controlled trials suggest that mI supplementation is helpful, for example, in managing treatment-resistant panic disorder [Citation117–120].
Interestingly, another body of work has identified glial cells as neuroimmune-sensors of stress: during development, glial cells are primed by stress (physical and psychological), making them prone to respond to subsequent stress with an amplified response [Citation121–124]. In FND this glial cell function may play a role in the subgroup of children with a history of cumulative stress. Also interesting is the body of work pertaining to chronic complex pain. This research suggests that chronic complex pain is maintained by reactive glial cells and interactions between glial cells and neurons [Citation125]. Since a significant proportion of children and adults with FND also report comorbid pain [Citation126] primed and activated glial cells, are likely to play an important role in both triggering and maintaining symptoms in both disorders.
2.8. Childhood physical abuse severity increases risk for aberrant change in neural network connectivity later in life
We include this study by Diez and colleagues (2020) because it documents how the experience of physical abuse in childhood leads to changes in neural network connectivity – via changes in gene expression – that increase vulnerability FND [Citation127]. The study looked at 30 adult patients (80% women) with motor FND. The researchers found that experience-dependent changes in gene expression (through childhood physical abuse) were coupled with aberrant changes in functional connectivity – between neural networks underpinning motor and sensory function and those underpinning attention, salience detection, arousal, and emotional state.
3. The emotion-processing system level: enhanced reaction times
In a study looking at emotion faces and reaction times – how quickly the children were able to identify the emotion in the face – our research team found that children with mixed FND (n = 57; 72% girls) performed better than controls [Citation128]. They had faster reaction times for all emotions (the raw scores for all emotions in this study can be found in of Kozlowska (2013) [Citation128]) For a visual representation of the data see Savage and colleagues (2022), Appendix A, p199 [Citation71]. There were also no differences in identification accuracy for any emotion. What these findings suggest is that children with FND allocate more resources to emotion stimuli – for example, emotion faces – than healthy controls, presumably as a means of checking the environment for the presence of threat. The findings also suggest that these children’s neurological systems have been primed, presumably by past experience, to respond to emotion stimuli faster.
4. The cognitive system level
In the clinic, children with FND commonly report that engagement in schoolwork is challenging because of problems with concentration or clarity of thought – what they sometimes refer to as ‘brain fog’ – or their struggles with fatigue or a general sense of dis-ease. In this section we summarize the findings from formal, laboratory-based assessments of cognitive function.
4.1. Overall neurocognitive function in children with mixed FND: a shift away from cognitive processing
In line with findings from MRI research – showing disruption within neural networks pertaining to cognitive functions - one study showed that children with mixed FND (n = 57; 72% girls) showed poorer performance (vs. healthy controls) on a battery of neurocognitive tests [Citation129]. The following domains were affected: attention, executive function, and memory. Interestingly, as the reader saw in the previous section, this same group of children had performed better (vs. healthy controls) on a face-recognition task: their reaction times were faster across all emotions. Together, these results suggest a shift of away from cognitive processing toward emotion and motor-sensory processing. The latter two modes of processing are privileged in the face of stress or threat, and in states of high arousal (see evolutionary theme discussed in the introduction). This shift is consistent with the fact that children with FND report more ACEs across the life span and that they perceive themselves to be more stressed and distressed on self-report measures [Citation95,Citation103]. A recent transdiagnostic reanalysis of this cognitive data using more sophisticated modeling showed that deficits in FND included executive function, working memory, cognitive flexibility, decision speed, and information-processing speed [Citation130].
4.2. Decreased sense of control in children with functional seizures
Sense of control refers to the extent to which the child perceives him- or herself to be in control [Citation131]. Children with FND report no control over their motor symptoms and over FS; they experience these symptoms as involuntary.
Stager and colleagues (2022) measured sense of control in children with FS (n = 26; 74% girls) using the magic and turbulence task [Citation73]. They assessed sense of control in three conditions: magic, lag, and turbulence. Children with FS had significantly poorer sense of control across tasks. Notably, in a study using Retraining and Control Therapy to treat FS, the children’s sense of control – that is, their awareness that control was being manipulated – increased over the eight weeks of the treatment intervention (n = 14 participated in follow-up) [Citation96]. The children’s improved sense of control was not solely attributable to improvements in FS frequency. The findings suggest that loss of sense of control may contribute to FS onset and FS maintenance, and that interventions that increase sense of control may be a critical element contributing to successful resolution of FS. Importantly, increasing sense of control is a key element across FS interventions available for children [Citation71,Citation72,Citation74].
An important caveat here is that sense of control – the sense of the movement being voluntary – is part of a child’s subjective experience of motor events that are largely automatic in nature. Hallett (2007) provides a comprehensive review and analysis of studies that examine the neurological substrate underpinning the generation of movement (an automatic process that occurs in the frontal lobe) and the subjective experience of the movement, along with the sense of ownership of control of the movement (a process that occurs in a neuron-glial network, specifically involving the parietal lobe and the insular cortex) [Citation132]. Decreased activation and connectivity of the parietal region – and more broadly, the temporo-parietal area – is a common finding in adult FND studies.
4.3. Abnormal focus-of-attention: selective attention and cognitive inhibition
FND symptoms increase when the child (or families) pay attention to the symptoms, and they decrease or disappear when attention is directed elsewhere [Citation56,Citation133]. During the initial clinical examination, neurologists use this feature of focus-of-attention in FND – symptom variability with distraction – in reaching a clinical diagnosis (see ) [Citation28–30]. In this context abnormal focus-of-attention is of particular interest.
Selective attention refers to the child’s ability to focus on a particular stimulus of his or her choice, and cognitive inhibition refers to the child’s ability to suppress attention to unwanted stimuli. The two functions are interrelated because selective attention to a stimulus requires that the child suppress attention to other stimuli.
Stager and colleagues (2022) used a modified, computerized Stroop task to assess both functions in 26 children (74% girls) [Citation73]. In the study’s baseline Stroop task involving congruent and incongruent tasks, no differences were found between children with FS and controls. When children with FS were presented with seizure-symptom words, however – the seizure-symptoms condition – they demonstrated longer reaction times. The delayed response time reflected their inability to selectively attend to the ink color and concurrently inhibit attention to the seizure-symptom words.
These findings of an abnormal attentional focus – to illness cues – is highly relevant to treatment. In Retraining and Control Therapy, for example, focus-of-attention is diverted away from the FS using a behavioral intervention (habit substitution): the child implements a physical response that is incompatible with FS symptoms (e.g. speaking or singing when speech is affected during episodes) [Citation72,Citation96]. In the Five-Step Plan used as part of a mind-body intervention for FS, focus-of-attention is diverted away from the warning signs of an FS to the action of implementing a regulation strategy [Citation71]. This process is intended to decrease body and brain activation since FS occur in states of activation but do not occur when the child is settled and calm. In the intervention used by Sawchuk and colleagues, attention is redirected to a biofeedback intervention that slows down the breathing rate and calms both the autonomic nervous system and the respiratory motor system (and associated cortical arousal and respiratory alkalosis; see section 2.2) [Citation74,Citation79]. And in hypnosis interventions for FND, changing focus-of-attention is a key part of the intervention [Citation134].
4.4. The role of illness-promoting cognitive processes
In a landmark paper, Gianaros and Wager (2015) outlined some of the brain-body pathways linking psychological stress to physical health problems [Citation135]. When working with children one can see these illness-promoting cognitive processes at work in front of one’s eyes. For example, one child with resolving leg paralysis was unable to walk (again) when the conflict with her parents was touched upon in a family session, activating intense feelings of distress. As another example, a perfectionistic boy recurrently activated his FS by beat-up-the-self thoughts – such as ‘that happened because I am an idiot,’ followed by an FS in front of his clinical team and parents. In a recent article – an international collaboration – we documented the illness-promoting cognitive processes that clinicians working with children with FND encounter daily in clinical practice [Citation75] and that then become the focus of the individual psychotherapy with the child [Citation70,Citation72,Citation74,Citation136].
In a recent study with 76 children with mixed FND (60 girls), the first author and colleagues worked with the young people – during their inpatient admission to the Mind-Body Program – to identify psychological processes that were part of their illness presentation and that needed to be addressed as part of the treatment process [Citation136]. ‘Illness-promoting psychological processes were identified in all children with FND. Most common were the following: chronic worries about schoolwork, friendships, or parental wellbeing (n = 64; 84.2%); attention to symptoms (n = 61; 80.3%); feeling sad (n = 58; 76.3%); experiencing a low sense of control (helplessness) in relation to symptoms (n = 44; 57.9%); pushing difficult thoughts out of mind (n = 44; 57.9%); self-critical rumination (n = 42; 55.3%); negative/catastrophic-symptom expectations (n = 40; 52.6%); avoidance of activities (n = 38; 50%); intrusive thoughts/feelings/memories associated with adverse events (n = 38, 50%); and pushing difficult feelings out of mind (n = 37; 48.7%)’ (p. 1) [Citation136].
5. The mental health system level
5.1. Comorbid mental health conditions
The largest study looking at comorbid mental health conditions in children with FND was run by Hansen and colleagues in Denmark [Citation137]. The researchers looked at a nationwide register – based cohort of children (n = 384; 82% girls) who had been registered with a diagnosis of FS, along with age- and sex-matched healthy controls, from 1 January 1996 to 31 December 2014. They found an elevated risk of psychiatric disorders in children with FS. Two-fifths (153; 39.8%) had a prevalent psychiatric diagnosis (a psychiatric disorder that had been diagnosed before the FS diagnosis) and two-fifths (150; 39.1%) had an incident psychiatric disorder (a psychiatric disorder that had been diagnosed in the two years after the FS diagnosis) (see ).
Table 4. Most common comorbid mental health diagnoses in the Danish health register study of children with functional seizures.
In the broader literature – across smaller cohorts of children with FND – rates of comorbid mental health disorders vary substantially from one cohort to another (22%−80%) [Citation69]. Rates tend to be higher in cohorts requiring an inpatient level of care and in cohorts where the comorbid mental health disorder has been diagnosed as part of a comprehensive clinical assessment using criteria from the Diagnostic and Statistical Manual of Mental Disorders (DSM) or International Classification of Diseases [Citation138]. Rates tend to be lower in cohorts treated in an outpatient setting and in cohorts where comorbid mental health disorders have been diagnosed using self-report tools [Citation72,Citation139,Citation140]. These differences reflect severity of illness – children requiring inpatient care tend to have higher levels of comorbidity – and the fact that self-report measures using psychological constructs are inferior to clinical assessment in children with FND. The latter is because children with FND – who somatize their distress – do not resonate with psychological constructs used by mental health clinicians, and they also frequently mask their underlying distress, with the consequence that rates of comorbid anxiety, depression, and distress are under-reported on self-report instruments [Citation139].
Identifying and addressing comorbid mental health issues is important. Outcome studies from the first author’s program found that outcomes are less favorable for children with FND whose comorbid mental health disorders do not resolve and for those who subsequently develop chronic mental health problems [Citation141]. From the neurobiology perspective, one can hypothesize that unresolved mental health issues, including the negative cognitive processes that characterize such presentations, contribute to stress-system activation and to dysregulation of neural networks [Citation142–145]. Unresolved mental health issues also negatively affect quality of life [Citation146–148]. Other factors contributing to less favorable outcomes include the following: chronic FND symptoms at presentation; comorbid epilepsy or other chronic health conditions; and other comorbid functional somatic symptoms that do not resolve [Citation141,Citation149].
5.2. Personality traits
Personality testing with children with FND (vs. healthy controls) has used the Brief Risk-Resilience Index for Screening (BRISC), the NEO-Five-Factor Inventory (NEO-FFI)], and the Millon Adolescent Clinical Inventory (MACI). On the BRISC, children with FND (n = 43; 31 girls) showed lower social skills and lower negativity bias scores on the BRISC (lower negativity bias scores mean the children see themselves and the world in a more negative light). On the NEO-FFI, children with FND (n = 56; 31 girls) showed higher neuroticism scores (neuroticism assesses the degree to which a person experiences the world as distressing, threatening, and unsafe). Using the MACI with children with FS (n = 20; 19 girls), Sawchuk and colleagues (2015) showed high levels of psychic tension, anxiety, depressive affect, and maladaptive personality traits, suggesting maladaptive patterns of coping [Citation150]. In that study, 85% of adolescents evidenced one or more maladaptive personality patterns, with inhibited, submissive, and introversive patterns (passive/avoidant coping strategies) being the most prevalent. Similar maladaptive personality traits were found in a latter study at the same treatment facility (n = 30, 21 girls) [Citation74]. The above-described pattern of findings is consistent with the illness-promoting psychological processes identified in children with FND during the treatment process (see section 4.4) [Citation136]. In a different study using the MACI with children with FS (n = 42; 31 girls), no differences were found between patients and controls [Citation140].
6. The physical health system level: comorbid physical health concerns
From a treatment perspective, the presence of comorbid epilepsy or other, less common brain disorders is the most important complicating medical condition. Epilepsy is a risk factor for the development of FS – presumably because brain networks are already compromised [Citation151,Citation152]. In adults, comorbid epilepsy is present in about 10%–30% of patients with FS [Citation153]. In children with FS, rates of concurrent epilepsy are less well documented. Yadav and colleagues found a rate of 21.1% in a sample from an epilepsy service [Citation149]. Hansen and colleagues (2020) found a rate of 14.2% in a Danish population sample [Citation6]. These researchers also found that comorbid epileptic seizures were associated with intellectual disabilities, school support, and a prolonged delay in diagnosing FS. From the perspective of the child and family, uncontrolled epileptic seizures reflect a chronic health condition, which involves ongoing physical stress (epileptic activity that dysregulates neural network function and increases risk for FS) and psychological stress associated with managing a chronic illness (see, e.g. the vignette of Hazel in Kozlowska et al. [2023] [Citation70]).
In this context, comorbid epilepsy complicates assessment and treatment in many ways and is likely to be associated with worse outcomes. Diagnostic delay – diagnosis once the FS are entrenched – is associated with worse outcomes [Citation141,Citation154]. Access to treatment may be compromised; because of safety concerns, the mental health clinicians running FS treatment programs may decline children with active epileptic seizures. Along the same lines, parents and teachers may struggle to tell the seizure types apart, making it difficult to implement an FS intervention. And finally, the scaffolding needed to implement an FS intervention in children with intellectual disability, children with autism, and children with other neurodevelopmental disorders is quite substantial and may exceed available resources [Citation98].
7. The social system level: family, friends, school, and socio-economic-political-ecological context
The social system level involves a complex interplay between the child and family’s lived experience, attachment, regulation capacity, and socio-economic-political-ecological context in which the family lives [Citation155–158]. As was highlighted in the introduction, sociocultural context intersects with individual vulnerability factors to yield sudden increases in FND presentations (see Box 1). Children from settled families (with a low occurrence of ACEs) living in adequate socio-economic-political-ecological contexts are more likely to have normative attachment relationships and better health outcomes. Children from unsettled families (with a high occurrence of ACEs) living in challenging socio-economic-political-ecological contexts are more likely to have at-risk attachment relationships and higher rates of adverse health outcomes. Below we consider some of the social determinants that contribute to the emergence of functional somatic symptoms (including FND). It is important to keep in mind that the effects of early childhood experiences are nonlinear and that the child’s state of health at any particular time can reflect many different developmental pathways – the idea of equifinality [Citation155–157].
7.1. Parental mental illness increases risk for functional somatic symptoms
In a large, prospective, longitudinal Danish study, Rask and colleagues looked at the associations between infant functioning in the first year of life (n = 1327; 50% girls) and at functional symptoms at ages 5–7 [Citation159]. They found that maternal psychiatric illness and the infant’s regulatory problems (feeding, sleeping, or tactile reactivity) during the first year of life were associated with impairing functional somatic symptoms at 5–7 years. Pain complaints (e.g. limb pain, headache, and stomachache) were the most frequent functional somatic symptoms in this age group.
In a subsequent study of children with FS (n = 386; 83% girls), Hansen and colleagues (2020) found that a fifth of the children had a parent with a history of psychiatric disorders [Citation6]. The presence of parental psychiatric disorders is a well-known ACE that affects the attachment relationship and the parent’s capacity to function as a psychobiological regulator – the capacity to help regulate the child’s stress system on a daily basis in the context of the repeating patterns of interactions that make up attachment relationships.
7.2. Quality of attachments
In a study using the Dynamic Maturational Model [Citation160,Citation161] to examine patterns of attachment in children with FND (n = 76; 53 girls), the children with FND were classified into at-risk patterns of attachment by blinded coders [Citation138]. By contrast, healthy controls were mostly classified into the normative patterns of attachment. The at-risk attachment patterns – or strategies – fell into two main clusters: Type A attachment strategies denoted by high subscripts (Type A+) and Type C attachment strategies denoted by high subscripts (Type C+). In addition, 75% of the children with FND had linguistic markers of unresolved loss and trauma (vs. 12% of controls). For a visual representation of the data see Savage and colleagues (2022), Appendix A, p195) [Citation71].
Children using the Type A+ strategies are good, compliant kids. Because their parents have been uncomfortable with the expression of negative affect – for example, fear, anger, or desire for comfort – these kids inhibit expressions of distress, and they do what their parents want them to do. They perform, they comply, and they please. By the school-age years, this group of kids take their parents’ perspective regarding what they should do, and they blame themselves for any problems. Because this pattern of emotional functioning becomes habitual, it can be very hard for these children to notice that their bodies are signaling distress, to know how they are feeling, and to be aware when they are not okay on the inside. It can also be hard for their parents (and clinicians) to tell from looking at them how they are feeling on the inside; their external signals function to reassure the parent that everything is okay. On clinical assessment the child may smile and may state that ‘everything is fine.’ The key is to look for discrepancies; for example, the child may say that she is fine, but her body is signaling a state of high arousal. From an attachment perspective, key therapeutic themes for this group of children are to help the child to read her body, to take note of her emotions, to communicate her emotions, and to practice these with the therapist and with her parents. In the family work, the likely goals are to help the child and parents to recognize potential stressors, to recognize and communicate distress, to take action to manage stress and distress, and so on.
Children using the Type C+ strategies are the coercive kids. In the context of family stress, their parents have been consistently inconsistent, so the children have learned to use exaggerated affect and also to alternate their displays of affect – in particular, anger, fear, and the desire for comfort – to ensure that their parents attend to them. By the school-age years, they take a ‘me, me, me’ perspective and blame others for their problems. An interesting finding in children with FND who use the Type C+ strategy was that they rarely signaled anger. It seemed that anger was not an acceptable emotion in the family system. Instead, the children signaled distress, a desire for comfort, being sick, or pain. The expression of anger was indirect and hidden. From an attachment perspective, the key therapeutic theme for this group of children is to establish a consistent, structured environment with explicit limits, clearly articulated expectations, assigned homework, and so on. The clinicians can expect passive resistance (helplessness and illness signals), which may need to be circumvented in creative ways. Rather than dwelling on body symptoms and emotions, the clinician may need to map and acknowledge these but then focus on skills to manage them better and put the responsibility for using the skills back on the child. In family sessions, it is important to help parents to respond to the child in clear and predictable ways, and to set clear and predictable expectations of what is expected.
7.3. The role of stress and adverse childhood experiences
In 2013, DSM-5 removed psychological stress from the FND diagnostic criteria. When working with children with FND, a wide lens for looking at stress is required. In children, a broad range of stressors can activate the brain and body stress systems – the final result being aberrant neural network function that manifests as symptoms of FND. In this context, while DSM-5 removed psychological stress from the FND diagnostic criteria [Citation162], stress during the developmental history or stress as a triggering or maintaining factor is typically part of the FND story in childhood [Citation6,Citation103,Citation150,Citation163–167]. The stress may be physical (e.g. illness, injury, excessive sports training, or medical procedures); psychological (e.g. moving schools or illness of a parent); relational (e.g. family conflict, peer difficulties, social rejection, or death of a pet); familial (e.g. mental health or physical illness of a parent); academic (difficulties with learning or pressure to perform academically or in sport); or cognitive (e.g. maladaptive cognitive processes that activate the stress system top-down, as in rumination, catastrophizing, or negative thoughts about the self) [Citation75,Citation135,Citation136]. The antecedent stress for the child’s presentation may be relatively minor – a fall, viral illness, or anxiety about examinations at school – but it may occur against a backdrop of cumulative ACEs [Citation103,Citation163]. More recently – exacerbated by ongoing climate change – ecological stress (e.g. exposure to bushfires, floods, drought, or heat) is increasingly common [Citation103].
Frequently, when the child denies any stress in his or her life, the body stress systems tell a different story: they are in a state of high activation (see and ). In other words, the somatic narrative – the story the body tells – may not be accompanied by a verbal narrative because the child and family have not made a connection between the ACEs suffered by the child (or the family in the current or previous generations) and the symptoms or because knowledge of the adverse experiences is kept out of mind.
7.4. Quality of life and quality of medical care
Stager and colleagues (2022) used the Pediatric Quality of Life Inventory to measure quality of life in children with FS (n = 84; 74% girls) before and after treatment with Retraining and Control Therapy [Citation73]. Initially, children with FS reported poorer quality of life than controls, including lower psychosocial health, physical functioning, emotional functioning, school functioning, and total health. Following the treatment intervention, quality of life improved significantly, an effect that was mediated by changes in FS frequency [Citation96]. These results highlight the important role of health clinicians in the health and well-being – quality of life – of children with FND [Citation73,Citation75,Citation168]. Accurate and early diagnosis and treatment of FND (including FS) is likely not only to reduce the stress caused by inappropriate medical investigations and health care interventions but to increase rates of remission and improve quality of life for children and their families.
8. Limitations
Research priorities set out by national statutory authorities reflect, in part, the beliefs and interests of clinicians, researchers, and the wider society – broadly known as the culture of care. In the field of FND, an outdated culture of care has long shaped resource allocation in both the clinical and research settings. The long-standing belief that ‘patients with FND are not deserving of health care resources’ (p. 2) [Citation75], coupled with a lack of interest from both neurologists and psychiatrists [Citation169], has resulted in a dearth of allocated research funding. The flow-on effect is that only a modest degree of research has been done on the neurobiology of pediatric FND. A potential limitation of this narrative review is that most of the studies reported here come from a handful of research teams: an American team in Alabama; an Australian team in Sydney; a Canadian team in Calgary; and a Danish team working across Aalborg and Aarhus.
9. Conclusion
The scientific findings presented in this review, coupled with a systemic (biopsychosocial) approach to patient care, suggest a broad range of potential interventions at different system levels – to be evaluated via future research. Mind strategies such as hypnosis could, in a subset of cases, facilitate top-down regulation in neuron-glial networks [Citation134]. Neuromodulation interventions of different types could potentially target any of the following: brain and body arousal, networks involved in motor or sensory processing, or excitability of neuron-glial networks. Virtual-reality interventions could potentially be used in any of the following ways: to shift focus-of-attention (during physiotherapy); to facilitate movement in limbs; to down-regulate (habituate) reactive glial cells and brain regions that underpin salience detection; or to correct aberrant predictions made by the brain’s predictive systems. Pharmacological interventions, potentially available in the future, could target neuroinflammatory processes involving glial cells or neuron-glial networks. And finally, clinical trials are needed to look at myo-inositol supplementation for the subgroup of patients with FND who trigger their FS by hyperventilation [Citation116]. The research opportunities abound.
10. Expert opinion
The neurobiology of pediatric FND is complex. It involves an elaborate interaction of factors from the biological, psychological, and socio-economic-political-ecological system levels. Keeping these complex interactions – and the multiple system levels – all in mind is difficult.
In understanding and caring for any particular child, what is required is a systems (biopsychosocial) approach involving different system levels – from the level of molecular processes, to the level of the mind, to the interaction with the child’s lived experience – and the relationships between them. To help the reader understand and hold in mind this complexity, we offer in this section a simple story of pediatric FND (as currently understood) and also two visual representations: a simple visual metaphor suitable for use with children and families, and a more complex visual representation that captures the complex multiple-system interactions in more detail.
The story of pediatric FND goes something like this:
The child who presents with FND has experienced stress after stress after stress. The origins may be physical, psychological, relational, familial, academic, cognitive (created by maladaptive cognitive processes that activate the child’s stress system top-down), or ecological.
When these stresses occur, they activate (each time) the stress systems in the body and brain. When activated repeatedly, various components of the stress system are sensitized (primed).
Stress causes HPA-axis activation – an increase in glucocorticoids (cortisol) secretion. Glucocorticoids increase the capacity for energy use in the short term. They also facilitate epigenetic changes in programing in the brain and in the stress system. They allow life experiences to be biologically embedded to change brain and body function, effectively preparing the child to mount an amplified, stronger response to future stress or threats.
Eventually, a stress event pushes the child’s system beyond its capacity to adapt. The immediate event itself may actually be relatively minor (a fall, sprain, or minor injury).
The response to the stressor – amplified by past priming – is excessive. Aberrant neuron-glial network changes are triggered, and FND symptoms emerge. Comorbidity with chronic/complex pain – a common feature of many presentations – implicates concurrent neuroinflammatory processes on the tissue, spinal cord, and brain system levels, including interactions between glial cells and neurons, and aberrant changes across and within neuron-glial networks.
Amplification of symptoms via attention and illness-promoting thoughts, feelings, and expectations – including implicit expectations pertaining to homeostatic processes (such as energy regulation) – implicates the ongoing role of the mind and brain in their coupled role as a generative system that models the world via prediction (see key themes in the introduction) [Citation58–60].
The child and family seek medical assessment. Prompt diagnosis allows for prompt treatment and good outcomes.
Delayed diagnosis leads to high levels of anxiety that further activate the child’s stress system. There is an increased focus-of-attention on the child’s symptoms because they are severe and because they impair the child’s function. The symptoms amplify when the parent attends to them or asks about them constantly. The family continues to seek medical attention to find an answer about the symptoms and to provide the child with treatment.
provides a simple visual representation of the FND story in visual form. We hope it will help the reader to link adverse life experiences, stress-system activation, and epigenetic/plasticity processes that increase vulnerability for FND. developed by Agorastos Agorastos provides a more comprehensive summary that researchers may find useful when considering important questions for future research [Citation47].
Figure 5. Visual representation linking adverse life experiences, stress system activation, and epigenetic/plasticity processes that increase vulnerability for FND. © Kasia Kozlowska 2021, reproduced with permission.
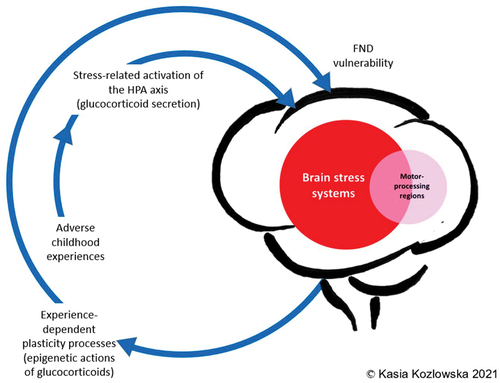
Figure 6. Conceptual model of developmental trajectories of early life stress leading to increased vulnerability to functional neurological disorder (FND) and other disparate health outcomes (including other comorbid functional conditions [e.g. chronic complex pain], comorbid mental health disorders, and concurrent physical health disorders). The model identifies the many different biological, psychological, and social factors that interact with adverse childhood experiences (ACEs) across development to alter allostatic processes and reduce adaptability to stress. Changed allostatic processes found in children with FND (discussed in this review) include the following: activation of autonomic nervous system, brain arousal systems, or immune system; dysregulation of HPA axis or sleep and circadian system; and, in the context of childhood abuse, epigenetic programming of genes modulating neuron development and brain network connectivity. The individual effects of ACEs on the organism depend on the specific genetic background and fetal programming (hit-1), the timing, duration, intensity, and type of ACEs (hit-2), and other later-life challenges, such as additional stressors, coping strategies, support availability, lifestyle, and aging (hit-3). Interactions among these factors explain inter-individual variations both in resilience and vulnerability to altered biopsychological functioning and in lifelong health outcomes.
![Figure 6. Conceptual model of developmental trajectories of early life stress leading to increased vulnerability to functional neurological disorder (FND) and other disparate health outcomes (including other comorbid functional conditions [e.g. chronic complex pain], comorbid mental health disorders, and concurrent physical health disorders). The model identifies the many different biological, psychological, and social factors that interact with adverse childhood experiences (ACEs) across development to alter allostatic processes and reduce adaptability to stress. Changed allostatic processes found in children with FND (discussed in this review) include the following: activation of autonomic nervous system, brain arousal systems, or immune system; dysregulation of HPA axis or sleep and circadian system; and, in the context of childhood abuse, epigenetic programming of genes modulating neuron development and brain network connectivity. The individual effects of ACEs on the organism depend on the specific genetic background and fetal programming (hit-1), the timing, duration, intensity, and type of ACEs (hit-2), and other later-life challenges, such as additional stressors, coping strategies, support availability, lifestyle, and aging (hit-3). Interactions among these factors explain inter-individual variations both in resilience and vulnerability to altered biopsychological functioning and in lifelong health outcomes.](/cms/asset/ebb5dfdb-0f71-4ab5-a8dc-9fb780c3a31a/iern_a_2333390_f0006_oc.jpg)
Finally, while the discussion of treatment is beyond the scope of this review, it is important to point out that our enhanced understanding of the neurobiology has had flow-on effects for diagnosis and treatment [Citation69–72,Citation74]. For example, since we know that symptoms amplify with attention and decrease when attention is directed elsewhere, the pediatric neurologist manipulates attention during the neurology assessment to yield, when appropriate, a positive diagnosis of FND [Citation28–30]. In both psychotherapy and physiotherapy, focus-of-attention is also a key element in working with the child, family, and school. Interventions that target down-regulation of the stress system – on multiple system levels – are likewise central to the treatment intervention. These interventions include ones that help children regulate their bodies better (bottom-up regulation strategies), that help children manage unhelpful feelings and cognitions (top-down regulation strategies), and that address real-life stressors in the family and school settings (whenever possible). The overarching aim of all these interventions is to facilitate increased regulation – a decreased state of arousal and better synchrony within the stress system and its components – to increase mastery and decrease subjective distress. The flow-on effect is the normalization of neural network function and the resolution of FND symptoms.
Article highlights
Pediatric FND is common: children with FND make up 10%–20% of presentations to neurology services in children’s hospitals.
FND is underpinned by aberrant changes within and between neuron-glial (brain) networks.
A variety of factors, on multiple system levels – physiological, emotional, cognitive, relational, and relating to adverse childhood experiences – contribute to brain network changes.
Activation or dysregulation of the stress system is a frequent finding in children with FND
Recent advances in understanding of neurobiology have led to the development of FND-specific treatment interventions.
Prompt diagnosis and treatment – presumably before neural network changes become entrenched – result in good outcomes.
Declaration of interest
The authors have no relevant affiliations or financial involvement with any organization or entity with a financial interest in or financial conflict with the subject matter or materials discussed in the manuscript. This includes employment, consultancies, honoraria, stock ownership or options, expert testimony, grants, or patents received or pending, or royalties.
Reviewer disclosures
Peer reviewers on this manuscript have no relevant financial or other relationships to disclose.
Acknowledgment
The authors would like to acknowledge all the children, adolescents, and families – from different parts of the world – that took part in the studies reported in this review. Without the donation of their time and good will, the current knowledge base in the field of pediatric FND would still be sparce.
Additional information
Funding
References
- Leary PM Conversion disorder in childhood-diagnosed too late, investigated too much. J R Soc Med. 2003;96:436–438 PubMed. doi: 10.1258/jrsm.96.9.436
- Operto FF, Coppola G, Mazza R, et al. Psychogenic nonepileptic seizures in pediatric population: A review. Brain Behav. 2019;9(12):e01406 PubMed. doi: 10.1002/brb3.1406
- Jungilligens J, Michaelis R, Popkirov S Misdiagnosis of prolonged psychogenic non-epileptic seizures as status epilepticus: epidemiology and associated risks. J Neurol Neurosurg Psychiatry. 2021;92(12):1341–1345. doi: 10.1136/jnnp-2021-326443
- Sigurdardottir KR, Olafsson E. Incidence of psychogenic seizures in adults: a population-based study in Iceland. Epilepsia. 1998;39(7):749–752. doi: 10.1111/j.1528-1157.1998.tb01161.x
- Villagrán A, Eldøen G, Duncan R, et al. Incidence and prevalence of psychogenic nonepileptic seizures in a Norwegian county: a 10‐year population‐based study. Epilepsia (Copenhagen). 2021;62(7):1528–535. doi: 10.1111/epi.16949
- Hansen AS, Rask CU, Rodrigo-Domingo M, et al. Incidence rates and characteristics of pediatric onset psychogenic nonepileptic seizures. Pediatr Res. 2020;88(5):796–803. doi: 10.1038/s41390-020-0945-z
- Kozlowska K, Nunn KP, Rose D, et al. Conversion disorder in Australian pediatric practice. J Am Acad Child Adolesc Psychiatry. 2007;46(1):68–75. doi: 10.1097/01.chi.0000242235.83140.1f
- Ani C, Reading R, Lynn R, et al. Incidence and 12-month outcome of non-transient childhood conversion disorder in the UK and Ireland. Br J Psychiatry. 2013;202:413–418 PubMed. doi: 10.1192/bjp.bp.112.116707
- Shephard B A war of nerves: soldiers and psychiatrists in the twentieth century. Cambridge Mass: Harvard University Press; 2001.
- Leff JP. Psychiatry around the globe: a transcultural view. 1st ed. (NY): Dekker; 1981.
- Da Costa JM On irritable heart; a clinical study of a form of functional cardiac disorder and its consequences. Am J Med Sci. 1871;121:2–52. doi: 10.1097/00000441-187101000-00001
- Kardiner A. The traumatic neuroses of war. Washington (DC): National Research Council; 1941.
- Crocq L Les Traumatismes Psychiques de Guerre. Paris: Odile Jacob; 1999.
- Kozlowska K, Scher S, Helgeland H. Historical context: terminology (online supplement 1.1). In: Dallos R Vetere A, editors Functional somatic symptoms in children and adolescents: the stress-system approach to assessment and treatment palgrave macmillan’s counselling and psychotherapy series. London: Palgrave Macmillan; 2020.
- Kozlowska K, Walker P, McLean L, et al. Fear and the defense cascade: clinical implications and management. Harv Rev Psychiatry. 2015;23(4):263–287. 10.1097/HRP.0000000000000065
- Grossman D. On combat: the psychology and physiology of deadly conflict in war and in peace. 1st ed. ed. Christensen LW, editor. Milstadt (Ill): PPCT Research Publications; 2004.
- Heyman I, Liang H, Hedderly T. COVID-19 related increase in childhood tics and tic-like attacks. Arch Dis Child. 2021;106(5):420–421. doi: 10.1136/archdischild-2021-321748
- Hull M, Parnes M. Tics and TikTok: functional tics spread through social media. Mov Disord Clin Pract. 2021;8(8):1248–1252. doi: 10.1002/mdc3.13267
- Pringsheim T, Ganos C, McGuire JF, et al. Rapid onset functional tic-like behaviors in young females during the COVID-19 pandemic. Mov Disord. 2021;36(12):2707–2713. doi: 10.1002/mds.28778
- Pringsheim T, Martino D. Rapid onset of functional tic-like behaviours in young adults during the COVID-19 pandemic. Eur J Neurol. 2021;28(11):3805–3808. doi: 10.1111/ene.15034
- Buts S, Duncan M, Owen T, et al. Paediatric tic-like presentations during the COVID-19 pandemic. Arch Dis Child. 2022;107(3):e17. doi: 10.1136/archdischild-2021-323002
- Muller-Vahl KR, Pisarenko A, Jakubovski E, et al. Stop that! It’s not Tourette’s but a new type of mass sociogenic illness. Brain. 2022;476–480. 145 2 10.1093/brain/awab316
- Paulus T, Baumer T, Verrel J, et al. Pandemic tic-like behaviors following social media consumption. Mov Disord. 2021;36(12):2932–2935. doi: 10.1002/mds.28800
- Han VX, Kozlowska K, Kothur K, et al. Rapid onset functional tic-like behaviours in children and adolescents during COVID-19: clinical features, assessment and biopsychosocial treatment approach. J Paediatr Child Health. 2022;58(7):1181–1187.10.1111/jpc.15932
- Nilles C, Pringsheim TM, Martino D. The recent surge of functional movement disorders: social distress or greater awareness? Curr Opin Neurol. 2022;35(4):485–493. doi: 10.1097/WCO.0000000000001074
- Martino D, Hedderly T, Murphy T, et al. The spectrum of functional tic-like behaviours: data from an international registry. Eur J Neurol. 2023;30(2):334–343. doi: 10.1111/ene.15611
- Guerriero RM, Pier DB, de Gusmao CM, et al. Increased pediatric functional neurological symptom disorders after the Boston marathon bombings: a case series. Pediatr Neurol. 2014;51(5):619–623. doi: 10.1016/j.pediatrneurol.2014.07.011
- Carson A, Lehn A, Ludwig L, et al. Explaining functional disorders in the neurology clinic: a photo story. Pract Neurol. 2015;16:56–61 PubMed. doi: 10.1136/practneurol-2015-001242
- Stone J. Functional neurological disorders: the neurological assessment as treatment. Pract Neurol. 2016;16(1):7–17. doi: 10.1136/practneurol-2015-001241
- Kozlowska K, Mohammad S. Functional neurological disorder in children and adolescents: assessment and treatment. In: Sivaswamy L, Kamat D, editors Symptom based approach to pediatric neurology. Switzerland AG: Springer Nature; 2022. p. 683–724.
- Viswanathan N, Benbadis SR. The diagnosis of functional seizures. VideoEEG in the context of a complete clinical picture remains the gold standard for diagnosis of functional seizures. Pract Neurol. 2022;March/April:63–66.
- Ricci L, Boscarino M, Assenza G, et al. Clinical utility of home videos for diagnosing epileptic seizures: a systematic review and practical recommendations for optimal and safe recording. Neurol Sci. 2021;42(4):1301–1309. doi: 10.1007/s10072-021-05040-5
- Beniczky SA, Fogarasi A, Neufeld M, et al. Seizure semiology inferred from clinical descriptions and from video recordings. How accurate are they? Epilepsy Behav. 2012;24(2):213–215. doi: 10.1016/j.yebeh.2012.03.036
- Avbersek A, Sisodiya S. Does the primary literature provide support for clinical signs used to distinguish psychogenic nonepileptic seizures from epileptic seizures? J Neurol Neurosurg Psychiatry. 2010;81(7):719–725. doi: 10.1136/jnnp.2009.197996
- Syed TU, LaFrance WC Jr., Kahriman ES, et al. Can semiology predict psychogenic nonepileptic seizures? A prospective study. Ann Neurol. 2011;69(6):997–1004. doi: 10.1002/ana.22345
- LaFrance WC Jr., Baker GA, Duncan R, et al. Minimum requirements for the diagnosis of psychogenic nonepileptic seizures: a staged approach: a report from the International league against epilepsy nonepileptic seizures task force. Epilepsia. 2013;54(11):2005–2018. doi: 10.1111/epi.12356
- Seneviratne U, Minato E, Paul E How reliable is ictal duration to differentiate psychogenic nonepileptic seizures from epileptic seizures? Epilepsy Behav. 2017;66:127–131 PubMed.10.1016/j.yebeh.2016.10.024
- Brigo F. Psychogenic nonepileptic seizures: we know what they are not. But do we know what they are? Epilepsy Behav. 2017;67:139. 10.1016/j.yebeh.2016.10.030
- Muthusamy S, Seneviratne U, Ding C, et al. Using semiology to classify epileptic seizures vs psychogenic nonepileptic seizures: a meta-analysis. Neurol Clin Pract. 2022;12(3):234–247. doi: 10.1212/CPJ.0000000000001170
- Popkirov S, Reuber M, Kozlowska K. Communicating diagnosis of Functional/Dissociative seizures. Functional/Dissociative seizure task force. Psychiatry Commission. International League Against Epilepsy (ILAE) 2022 Oct 8. Available from: https://www.youtube.com/watch?v=oq6JhqPq5d
- Capra F. The web of life: a new synthesis of mind and matter. London: Flamingo; 1997.
- Jackson JH. The Croonian Lectures on evolution and dissolution of the nervous system. Br Med J. 1884;1(1213):591–593. 10.1136/bmj.1.1213.591
- MacLean PD. The triune brain in evolution: role in paleocerebral functions. (NY): Plenum Press; 1990p. xxiv, 672 p.
- Knyazev GG. EEG delta oscillations as a correlate of basic homeostatic and motivational processes. Neurosci Biobehav Rev. 2012;36(1):677–95. doi: 10.1016/j.neubiorev.2011.10.002
- Arnsten AF. Stress weakens prefrontal networks: molecular insults to higher cognition. Nat Neurosci. 2015;18(10):1376–1385. doi: 10.1038/nn.4087
- Raut RV, Snyder AZ, Mitra A, et al. Global waves synchronize the brain’s functional systems with fluctuating arousal. Sci Adv. 2021;7(30). doi: 10.1126/sciadv.abf2709
- Agorastos A, Pervanidou P, Chrousos GP, et al. Developmental trajectories of early life stress and trauma: a narrative review on neurobiological aspects beyond stress system dysregulation. Front Psychiatry. 2019;10:118
- Pervanidou P, Chrousos GP. Early-life stress: from neuroendocrine mechanisms to stress-related disorders. Horm Res Paediatr. 2018;89(5):372–379. doi: 10.1159/000488468
- McEwen BS, Flier JS, Underhill LH. Protective and damaging effects of stress mediators. N Engl J Med. 1998;338(3):171–179. doi: 10.1056/NEJM199801153380307
- McEwen BS, Gray JD, Nasca C. 60 Years of neuroendocrinology: redefining neuroendocrinology: stress, sex and cognitive and emotional regulation. J Endocrinol. 2015;226(2):T67–T83. doi: 10.1530/JOE-15-0121
- Zannas AS, Chrousos GP. Epigenetic programming by stress and glucocorticoids along the human lifespan. Mol Psychiatry. 2017;22(5):640–646. doi: 10.1038/mp.2017.35
- Felitti VJ, Anda RF, Nordenberg D, et al. Relationship of childhood abuse and household dysfunction to many of the leading causes of death in adults. The adverse childhood experiences (ACE) study. Am J Prev Med. 1998;14(4):245–258. doi: 10.1016/S0749-3797(98)00017-8
- Boyce WT, Levitt P, Martinez FD, et al. Genes, environments, and time: the biology of adversity and resilience. Pediatrics. 2021;147(2). doi: 10.1542/peds.2020-1651
- Sterling P, Eyer J. Allostatis: a new paradigm to explain arousal pathology. In: Fisher S, Reason J, editors Handbook of life stress, cognition and health. Chichester: Wiley; 1988. p. 629–649.
- McEwen BS, Stellar E. Stress and the individual. Mechanisms leading to disease. Arch Intern Med. 1993;153(18):2093–2101. 10.1001/archinte.1993.00410180039004
- Kozlowska K, Scher S, Helgeland H. Functional somatic symptoms in children and adolescents: a stress-system approach to assessment and treatment. London: Palgrave Macmillan; 2020.
- McCraty R, Childre D. Coherence: bridging personal, social, and global health. Altern Ther Health Med. 2010;16(4):10–24.
- Hallett M, Aybek S, Dworetzky BA, et al. Functional neurological disorder: new subtypes and shared mechanisms. Lancet Neurol. 2022;21(6):537–550. doi: 10.1016/S1474-4422(21)00422-1
- Edwards MJ, Adams RA, Brown H, et al. A Bayesian account of ‘hysteria’. Brain. 2012;135(11):3495–3512. doi: 10.1093/brain/aws129
- Kleckner IR, Zhang J, Touroutoglou A, et al. Evidence for a large-scale brain system supporting allostasis and interoception in humans. Nat Human Behav. 2017;1(5). 10.1038/s41562-017-0069
- American Psychiatric Association. Diagnostic and statistical manual of mental disorders: DSM-5-TR. 5th edition, text revision. ed. Washington (DC): APA Publishing; 2022.
- Vachon-Presseau E, Centeno MV, Ren W, et al. The emotional brain as a predictor and amplifier of chronic pain. J Dent Res. 2016;95(6):605–612. doi: 10.1177/0022034516638027
- Polese D, Belli A, Esposito D, et al. Psychological disorders, adverse childhood experiences and parental psychiatric disorders in children affected by headache: a systematic review. Neurosci Biobehav Rev. 2022;140:104798. doi: 10.1016/j.neubiorev.2022.104798
- Raucci U, Boni A, Evangelisti M, et al. Lifestyle modifications to help prevent headache at a developmental age. Front Neurol. 2021;11:618375. doi: 10.3389/fneur.2020.618375
- Papetti L, Alaimo Di Loro P, Tarantino S, et al. I stay at home with headache. A survey to investigate how the lockdown for COVID-19 impacted on headache in Italian children. Cephalalgia. 2020;40(13):1459–1473. doi: 10.1177/0333102420965139
- Hyams JS, Di Lorenzo C, Miguel Saps M, et al. Childhood functional gastrointestinal disorders: child/adolescent. Gastroenterology. 2016;150(6):1456–68.e2. doi: 10.1053/j.gastro.2016.02.015
- Drossman DA, Hasler WL. Rome IV-Functional GI disorders: disorders of gut-brain interaction. Gastroenterology. 2016;150(6):1257–1261. doi: 10.1053/j.gastro.2016.03.035
- Raucci U, Borrelli O, Di Nardo G, et al. Cyclic vomiting syndrome in children. Front Neurol. 2020;11:583425. doi: 10.3389/fneur.2020.583425
- Vassilopoulos A, Mohammad S, Dure L, et al. Treatment approaches for functional neurological disorders in children. Curr Treat Options Neurol. 2022;24(2):77–97. doi: 10.1007/s11940-022-00708-5
- Kozlowska K, Chudleigh C, Savage B, et al. Evidence-based mind-body interventions for children and adolescents with functional neurological disorder. Harv Rev Psychiatry. 2023;31(2):60–82. doi: 10.1097/HRP.0000000000000358
- Savage B, Chudleigh C, Hawkes C, et al. Treatment of functional seizures in children and adolescents: a mind-body manual for health professionals: Australian Academic Press; 2022.
- Fobian AD, Long DM, Szaflarski JP. Retraining and control therapy for pediatric psychogenic non-epileptic seizures. Ann Clin Transl Neurol. 2020;7(8):1410–1419. doi: 10.1002/acn3.51138
- Stager L, Morriss S, McKibben L, et al. Sense of control, selective attention and cognitive inhibition in pediatric functional seizures: a prospective case-control study. Seizure. 2022;98:79–86 PubMed. doi: 10.1016/j.seizure.2022.03.021
- Sawchuk T, Buchhalter J, Senft B. Psychogenic nonepileptic seizures in children-prospective validation of a clinical care pathway & risk factors for treatment outcome. Epilepsy Behav. 2020;105:106971. doi: 10.1016/j.yebeh.2020.106971
- Kozlowska K, Sawchuk T, Waugh JL, et al. Changing the culture of care for children and adolescents with functional neurological disorder. Epilepsy & Behavior Reports. 2021;16:1004486. doi: 10.1016/j.ebr.2021.100486
- Popkirov S. Double vision. Brain. 2023;146(1):2–3. doi: 10.1093/brain/awac409
- Kozlowska K, Palmer DM, Brown KJ, et al. Reduction of autonomic regulation in children and adolescents with conversion disorders. Psychosom Med. 2015;77(4):356–70. doi: 10.1097/PSY.0000000000000184
- Chudleigh C, Savage B, Cruz C, et al. Use of respiratory rates and heart rate variability in the assessment and treatment of children and adolescents with functional somatic symptoms. Clin Child Psychol Psychiatry. 2019;24(1):29–39. doi: 10.1177/1359104518807742
- Sawchuk T, Buchhalter J, Senft B. Psychogenic non-epileptic seizures in children – psychophysiology & dissociative characteristics. Psychiatry Res. 2020;294:113544. doi: 10.1016/j.psychres.2020.113544
- Paredes-Echeverri S, Maggio J, Begue I, et al. Autonomic, endocrine, and inflammation profiles in functional neurological disorder: a systematic review and meta-analysis. J neuropsych clin neurosci. 2022;34(1):30–43. doi: 10.1176/appi.neuropsych.21010025
- Kozlowska K. The body comes to family therapy: utilizing research to formulate treatment interventions with somatising children and their families. Aust N Z J Fam Ther. 2016;37:6–29. doi: 10.1002/anzf.1133
- Kozlowska K, Rampersad R, Cruz C, et al. The respiratory control of carbon dioxide in children and adolescents referred for treatment of psychogenic non-epileptic seizures. Eur Child Adolesc Psychiatry. 2017;26(10):1207–1217. doi: 10.1007/s00787-017-0976-0
- Benarroch EE. Brainstem respiratory chemosensitivity: new insights and clinical implications. Neurology. 2007;68(24):2140–3. doi: 10.1212/01.wnl.0000266560.60371.98
- Braun M, Sawchuk T, Simpkins A, et al. Quantitative EEG during hyperventilation as a biomarker for pediatric psychogenic non-epileptic seizures (PNES). Poster session presented at the annual meeting of the American Epilepsy Society, Chicago, USA. 2021.
- Fleming S, Thompson M, Stevens R, et al. Normal ranges of heart rate and respiratory rate in children from birth to 18 years of age: a systematic review of observational studies. Lancet. 2011;377(9770):1011–1018. doi: 10.1016/S0140-6736(10)62226-X
- Wallis LA, Healy M, Undy MB, et al. Age related reference ranges for respiration rate and heart rate from 4 to 16 years. Arch Dis Child. 2005;90(11):1117–1121. doi: 10.1136/adc.2004.068718
- Sparing R, Dafotakis M, Buelte D, et al. Excitability of human motor and visual cortex before, during, and after hyperventilation. J Appl Physiol (1985). 2007;102(1):406–411. doi: 10.1152/japplphysiol.00770.2006
- Jensen O, Hari R, Kaila K. Visually evoked gamma responses in the human brain are enhanced during voluntary hyperventilation. Neuroimage. 2002;15(3):575–586. doi: 10.1006/nimg.2001.1013
- Carbon M, Wübbeler G, Trahms L, et al. Hyperventilation-induced human cerebral magnetic fields non-invasively monitored by multichannel ‘direct current’ magnetoencephalography. Neurosci Lett. 2000;287:227–230 PubMed. doi: 10.1016/S0304-3940(00)01192-7
- Stenkamp K, Palva JM, Uusisaari M, et al. Enhanced temporal stability of cholinergic hippocampal gamma oscillations following respiratory alkalosis in vitro. J Neurophysiol. 2001;85(5):2063 PubMed–9. doi: 10.1152/jn.2001.85.5.2063
- Engel GL, Ferris EB, Logan M. Hyperventilation: analysis of clinical symptomatology. Ann Intern Med. 1947;27:683–704 PubMed.
- Hauge A, Thoresen M, Walloe L. Changes in cerebral blood flow during hyperventilation and CO2-breathing measured transcutaneously in humans by a bidirectional, pulsed, ultrasound doppler blood velocitymeter. Acta Physiol Scand. 1980;110(2):167 PubMed–73. doi: 10.1111/j.1748-1716.1980.tb06647.x
- Kraaier V, van Huffelen AC, Wieneke GH. Changes in quantitative EEG and blood flow velocity due to standardized hyperventilation; a model of transient ischaemia in young human subjects. Electroencephalogr Clin Neurophysiol. 1988;70(5):377–387. doi:10.1016/0013-4694(88)90015-6
- Gibbs DM. Hyperventilation-induced cerebral ischemia in panic disorder and effect of nimodipine. Am J Psychiatry. 1992;149(11):1589–1591.
- Rai S, Foster S, Griffiths KR, et al. Altered resting-state neural networks in children and adolescents with functional neurological disorder. NeuroImage Clin. 2022;35:103110.
- Stager L, Mueller C, Morriss S, et al. Sense of control, selective attention, cognitive inhibition, and psychosocial outcomes after retraining and control therapy (ReACT) in pediatric functional seizures. Epilepsy Behav. 2023;142:109143–. doi: 10.1016/j.yebeh.2023.109143
- Kozlowska K, Chudleigh C, Cruz C, et al. Psychogenic non-epileptic seizures in children and adolescents: part I – Diagnostic formulations. Clin Child Psychol Psychiatry. 2018;23(1):140–159. doi: 10.1177/1359104517732118
- Kozlowska K, Chudleigh C, Cruz C, et al. Psychogenic non-epileptic seizures in children and adolescents: part II – explanations to families, treatment, and group outcomes. Clin Child Psychol Psychiatry. 2018;23(1):160–176. doi: 10.1177/1359104517730116
- Clow A, Hucklebridge F, Thorn L. The cortisol awakening response in context. Int Rev Neurobiol. 2010;93:153–175 PubMed.
- Baumler D, Kirschbaum C, Kliegel M, et al. The cortisol awakening response in toddlers and young children. Psychoneuroendocrinology. 2013;38(11):2485–2492. doi: 10.1016/j.psyneuen.2013.05.008
- Platje E, Vermeiren RR, Branje SJ, et al. Long-term stability of the cortisol awakening response over adolescence. Psychoneuroendocrinology. 2013;38(2):271–280. doi: 10.1016/j.psyneuen.2012.06.007
- Filaire E, Ferreira JP, Oliveira M, et al. Diurnal patterns of salivary alpha-amylase and cortisol secretion in female adolescent tennis players after 16 weeks of training. Psychoneuroendocrinology. 2013;38(7):1122–1132. doi: 10.1016/j.psyneuen.2012.11.001
- Chung J, Mukerji S, Kozlowska K. Cortisol and α-amylase awakening response in children and adolescents with functional neurological (conversion) disorder. Aust N Z J Psychiatry. 2022;57(1):115–129. doi: 10.1177/00048674221082520
- Baumeister D, Akhtar R, Ciufolini S, et al. Childhood trauma and adulthood inflammation: a meta-analysis of peripheral C-reactive protein, interleukin-6 and tumour necrosis factor-alpha. Mol Psychiatry. 2016;21(5):642–649. doi: 10.1038/mp.2015.67
- Jonker I, Rosmalen JGM, Schoevers RA. Childhood life events, immune activation and the development of mood and anxiety disorders: the TRAILS study. Transl Psychiatry. 2017;7(5):e1112. doi: 10.1038/tp.2017.62
- Kozlowska K, Chung J, Cruickshank B, et al. Blood CRP levels are elevated in children and adolescents with functional neurological symptom disorder. Eur Child Adolesc Psychiatry. 2019;28(4):491–504. doi: 10.1007/s00787-018-1212-2
- Umesh S, Tikka SK, Goyal N, et al. Aberrant gamma band cortical sources and functional connectivity in adolescents with psychogenic non-epileptic seizures: a preliminary report. Psychiatry Res. 2017;247:51–54. PubMed. doi: 10.1016/j.psychres.2016.11.003
- Kozlowska K, Spooner CJ, Palmer DM, et al. “Motoring in idle”: the default mode and somatomotor networks are overactive in children and adolescents with functional neurological symptoms. NeuroImage Clin. 2018;18:730–743. PubMed. doi: 10.1016/j.nicl.2018.02.003
- Vuilleumier P. Brain circuits implicated in psychogenic paralysis in conversion disorders and hypnosis. Neurophysiol Clin. 2014;44(4):323–337. doi: 10.1016/j.neucli.2014.01.003
- Voon V. Functional neurological disorders: imaging. Neurophysiol Clin. 2014;44(4):339 PubMed–42. doi: 10.1016/j.neucli.2014.07.003
- Perez DL, Nicholson TR, Asadi-Pooya AA, et al. Neuroimaging in functional neurological disorder: state of the field and research agenda. NeuroImage Clin. 2021;30:102623. doi: 10.1016/j.nicl.2021.102623
- Radmanesh M, Jalili M, Kozlowska K. Activation of functional brain networks in children and adolescents with psychogenic non-epileptic seizures. Front Hum Neurosci. 2020;14:339. doi: 10.3389/fnhum.2020.00339
- Kozlowska K, Melkonian D, Spooner CJ, et al. Cortical arousal in children and adolescents with functional neurological symptoms during the auditory oddball task. NeuroImage Clin. 2017;13:228–236 PubMed. doi: 10.1016/j.nicl.2016.10.016
- Madec T, Lagarde S, McGonigal A, et al. Transient cortico-cortical disconnection during psychogenic nonepileptic seizures (PNES). Epilepsia. 2020;61(8): PubMed e101–e106. doi: 10.1111/epi.16623
- Kozlowska K, Griffiths KR, Foster SL, et al. Grey matter abnormalities in children and adolescents with functional neurological symptom disorder. NeuroImage Clin. 2017;15:306–314 PubMed. doi: 10.1016/j.nicl.2017.04.028
- Charney M, Foster S, Shuklab V, et al. Neurometabolic alterations in children and adolescents with functional neurological disorder NeuroImage clinical. NeuroImage Clin. 2024;41:103557. doi: 10.1016/j.nicl.2023.103557.
- Zulfarina MS, Syarifah-Noratiqah SB, Nazrun SA, et al. Pharmacological therapy in panic disorder: Current guidelines and novel drugs discovery for treatment-resistant patient. Clin Psychopharmacol Neurosci. 2019;17(2):145–154. doi: 10.9758/cpn.2019.17.2.145
- Benjamin J, Levine J, Fux M, et al. Double-blind, placebo-controlled, crossover trial of inositol treatment for panic disorder. Am J Psychiatry. 1995;152(7):1084–1086.
- Palatnik A, Frolov K, Fux M, et al. Double-blind, controlled, crossover trial of inositol versus fluvoxamine for the treatment of panic disorder. J Clin Psychopharmacol. 2001;21(3):335–339. doi: 10.1097/00004714-200106000-00014
- Concerto C, Chiarenza C, Di Francesco A, et al. Neurobiology and applications of inositol in psychiatry: a narrative review. Curr Issues Mol Biol. 2023;45(2):1762–778. doi: 10.3390/cimb45020113
- Frank MG, Fonken LK, Watkins LR, et al. Microglia: neuroimmunesensors of stress. Semin Cell Dev Biol. 2019;94:176–185 PubMed. doi: 10.1016/j.semcdb.2019.01.001
- Frank MG, Weber MD, Watkins LR, et al. Stress-induced neuroinflammatory priming: a liability factor in the etiology of psychiatric disorders. Neurobiol Stress. 2016;4:62–70 PubMed. doi: 10.1016/j.ynstr.2015.12.004
- Brenhouse HC, Schwarz JM. Immunoadolescence: Neuroimmune development and adolescent behavior. Neurosci Biobehav Rev. 2016;70:288–299. doi: 10.1016/j.neubiorev.2016.05.035
- Brenhouse HC, Danese A, Grassi-Oliveira R. Neuroimmune impacts of early-life stress on development and psychopathology. Curr Top Behav Neurosci. 2019;43:423–447.
- Donnelly CR, Andriessen AS, Chen G, et al. Central nervous system targets: glial cell mechanisms in chronic pain. Neurotherapeutics. 2020;17(3):846–60. 10.1007/s13311-020-00905-7
- Perez DL, Aybek S, Popkirov S, et al. A review and expert opinion on the neuropsychiatric assessment of motor functional neurological disorders. J Neuropsychiatry Clin Neurosci. 2021;33(1):14–26. doi: 10.1176/appi.neuropsych.19120357
- Diez I, Larson AG, Nakhate V, et al. Early-life trauma endophenotypes and brain circuit–gene expression relationships in functional neurological (conversion) disorder. Mol Psychiatry. 2020:3817–3828. doi: 10.1038/s41380-020-0665-0. 26 8
- Kozlowska K, Brown KJ, Palmer DM, et al. Specific biases for identifying facial expression of emotion in children and adolescents with conversion disorders. Psychosom Med. 2013;75(3):272–280. doi: 10.1097/PSY.0b013e318286be43
- Kozlowska K, Palmer DM, Brown KJ, et al. Conversion disorder in children and adolescents: a disorder of cognitive control. J Neuropsychol. 2015;9(1):87–108. doi: 10.1111/jnp.12037
- Hilton RA, Tozzi L, Nesamoney S, et al. Transdiagnostic Neurocognitive Dysfunction in Children and adolescents with mental illness. Nat Mental Health. 2024;2(3):299–309. doi: 10.1038/s44220-023-00199-6
- Grob A, Little TD, Wanner B. Control judgements across the lifespan. Int J Behavioral Develop. 1999;23(4):833–854. doi: 10.1080/016502599383568
- Hallett M. Volitional control of movement: the physiology of free will. Clin Neurophysiol. 2007;118(6):1179–1192. doi: 10.1016/j.clinph.2007.03.019
- Edwards MJ, Fotopoulou A, Parees I. Neurobiology of functional (psychogenic) movement disorders. Curr Opin Neurol. 2013;26(4):442–447. doi: 10.1097/WCO.0b013e3283633953
- Helgeland H, Savage B, Kozlowska K. Hypnosis in the treatment of functional somatic symptoms in children and adolescents. In: Linden JH, De Benedittis G, Sugarman LJ, and Varga K, editors. The Routledge international handbook of clinical hypnosis: Routledge Taylor & Francis; 2024. p. 515–536.
- Gianaros PJ, Wager TD. Brain-body pathways linking psychological stress and physical health. Curr Dir Psychol Sci. 2015;24(4):313–321. doi: 10.1177/0963721415581476
- Kozlowska K, Schollar-Root O, Savage B, et al. Illness-promoting psychological processes in children and adolescents with functional neurological disorder. Children (Basel). 2023;10(11):1724. doi: 10.3390/children10111724
- Hansen AS, Rask CU, Christensen AE, et al. Psychiatric disorders in children and adolescents with psychogenic nonepileptic seizures. Neurology. 2021;97(5):e464–e75. doi: 10.1212/WNL.0000000000012270
- Kozlowska K, Scher S, Williams LM. Patterns of emotional-cognitive functioning in pediatric conversion patients: implications for the conceptualization of conversion disorders. Psychosom Med. 2011;73(9):775–788. doi: 10.1097/PSY.0b013e3182361e12
- Kozlowska K, Cruz C, Davies F, et al. The utility (or not) of self-report instruments in family assessment for child and adolescent conversion disorders? ANZ J Of Family Therapy. 2016;37:480–499. doi: 10.1002/anzf.1187
- Stager L, Morriss S, Szaflarski JP, et al. Psychiatric and personality factors in pediatric functional seizures: a prospective case-control study. Seizure. 2022;98:105–112 PubMed. doi: 10.1016/j.seizure.2022.04.006
- Kozlowska K, Gray N, Scher S, et al. Psychologically informed physiotherapy as part of a multidisciplinary rehabilitation program for children and adolescents with functional neurological disorder: physical and mental health outcomes. J Paediatr Child Health. 2021;57(1):73–79. doi: 10.1111/jpc.15122
- Breukelaar IA, Erlinger M, Harris A, et al. Investigating the neural basis of cognitive control dysfunction in mood disorders. Bipolar Disord. 2020;22(3):286–295. doi: 10.1111/bdi.12844
- Bryant RA, Andrew E, Korgaonkar MS. Distinct neural mechanisms of emotional processing in prolonged grief disorder. Psychol Med. 2021;51(4):587–595. doi: 10.1017/S0033291719003507
- Korgaonkar MS, Tran J, Felmingham KL, et al. Neural correlates of emotional processing in panic disorder. NeuroImage Clin. 2021;32:102902. doi: 10.1016/j.nicl.2021.102902
- Breukelaar IA, Bryant RA, Korgaonkar MS. The functional connectome in posttraumatic stress disorder. Neurobiol Stress. 2021;14:100321. doi: 10.1016/j.ynstr.2021.100321
- Scott JG, Mihalopoulos C, Erskine HE, et al. Childhood mental and developmental disorders. In: Patel V, Chisholm D, Dua T, Laxminarayan R, Medina-Mora M, editors. Disease control priorities, third edition: volume 4 mental, neurological, and substance use disorders: Washington (DC): World Bank; 2016.
- World_Health_Organization. Mental health of adolescents. 2021. cited 2023 Feb 25. Available from: https://www.who.int/news-room/fact-sheets/detail/adolescent-mental-health.
- Schlack R, Peerenboom N, Neuperdt L, et al. The effects of mental health problems in childhood and adolescence in young adults: results of the KiGGS cohort. J Health Monit. 2021;6(4):3–19. doi: 10.25646/8863
- Yadav A, Agarwal R, Park J. Outcome of psychogenic nonepileptic seizures (PNES) in children: a 2-year follow-up study. Epilepsy Behav. 2015;53:168–173 PubMed. doi: 10.1016/j.yebeh.2015.10.017
- Sawchuk T, Buchhalter J. Psychogenic nonepileptic seizures in children – psychological presentation, treatment, and short-term outcomes. Epilepsy Behav. 2015;52(Pt A):49–56. doi: 10.1016/j.yebeh.2015.08.032
- Paldino MJ, Chu ZD, Chapieski ML, et al. Repeatability of graph theoretical metrics derived from resting-state functional networks in paediatric epilepsy patients. Br J Radiol. 2017;90(1074):20160656 PubMed. doi: 10.1259/bjr.20160656
- Vaessen MJ, Braakman HM, Heerink JS, et al. Abnormal modular organization of functional networks in cognitively impaired children with frontal lobe epilepsy. Cereb Cortex. 2013;23(8):1997–2006. PubMed. doi: 10.1093/cercor/bhs186
- Liampas A, Markoula S, Zis P, et al. Psychogenic non-epileptic seizures (PNES) in the context of concurrent epilepsy – making the right diagnosis. Acta Epileptologica. 2021;3(1):1–11. doi: 10.1186/s42494-021-00057-x
- Reilly C, Jette N, Johnson EC, et al. Scoping review and expert-based consensus recommendations for assessment and management of psychogenic non-epileptic (functional) seizures (PNES) in children: a report from the Pediatric Psychiatric Issues Task Force of the International League Against Epilepsy. Epilepsia. 2023;64(12):3160–3195. doi: 10.1111/epi.17768
- Bowlby J. Attachment and loss: volume II: separation, anxiety and anger. London: Hogarth Press and Institute of Psycho-Analysis, 1973.
- Bowlby J. Developmental psychiatry comes of age. Am J Psychiatry. 1988;145(1):1–10.
- Sroufe LA, Coffino B, Carlson EA. Conceptualizing the role of early experience: lessons from the Minnesota longitudinal study. Dev Rev. 2010;30(1):36–51. doi: 10.1016/j.dr.2009.12.002
- Marmot M, Allen J, Bell R, et al. WHO European review of social determinants of health and the health divide. Lancet. 2012;380(9846):1011–1029.doi: 10.1016/S0140-6736(12)61228-8
- Rask CU, Ornbol E, Olsen EM, et al. Infant behaviors are predictive of functional somatic symptoms at ages 5–7 years: results from the Copenhagen child cohort CCC2000. J Pediatr. 2013;162:335–342 PubMed. doi: 10.1016/j.jpeds.2012.08.001
- Crittenden PM. Danger and development: the organization of self-protective strategies. Monographs Society Res Child. 1999;64(3):145–171. 10.1111/1540-5834.00037
- Farnfield S, Hautamaki A, Nørbech P, et al. DMM assessments of attachment and adaptation: procedures, validity and utility. Clin Child Psychol Psychiatry. 2010;15(3):313–328. doi: 10.1177/1359104510364315
- American Psychiatric Association. Diagnostic and statistical manual of mental disorders: dSM-5. 4th ed. Arlington (VA): American Psychiatric Association; 2013.
- Verrotti A, Agostinelli S, Mohn A, et al. Clinical features of psychogenic non-epileptic seizures in prepubertal and pubertal patients with idiopathic epilepsy. Neurol Sci. 2009;30(4):319–323. doi: 10.1007/s10072-009-0107-x
- Yang T, Roberts C, Winton-Brown T, et al. Childhood trauma in patients with epileptic vs nonepileptic seizures. Epilepsia. 2022.
- Yong K, Chin RFM, Shetty J, et al. Functional neurological disorder in children and young people: incidence, clinical features, and prognosis. Dev Med Child Neurol. 2023;65(9):1238–1246. doi: 10.1111/dmcn.15538
- Yeom JS, Bernard H, Koh S. Gender differences in risk factors and psychosocial functioning in children with psychogenic nonepileptic seizures. Epilepsy Behav. 2022;136:108884. doi: 10.1016/j.yebeh.2022.108884
- Lim N, Wood N, Prasad A, et al. COVID-19 vaccination in Young People with functional neurological disorder: a case-control study. Vaccines (Basel). 2022;10(12):2031. doi: 10.3390/vaccines10122031
- Rask CU, Bonvanie IJ, Garralda EM. Risk and protective factors and course of functional somatic symptoms in young people. In: Hodes M, Gau SS-F, De Vries PJ, editors. Understanding uniqueness and diversity in child and adolescent mental health: Academic Press; 2018. p. 77–113.
- Begue I, Nicholson TR, Kozlowska K, et al. Psychiatry’s modern role in functional neurological disorder: join the renaissance. Psychol Med. 2021:1–3. doi: 10.1017/S0033291721002038
- Duarte FV, Palmeira CM, Rolo AP. The role of microRnas in Mitochondria: small players acting wide. Genes (Basel). 2014;5(4):865–886. doi: 10.3390/genes5040865