Abstract
The medicinal herb parasitic loranthus in a screen was found to inhibit fatty acid synthase (EC 2.3.1.85, FAS) and reduce body weight of rats in our previous study. Now we have determined the inhibitory characteristics and kinetic parameters of extracts of parasitic loranthus [Taxillus chinensis (DC.) Danser]. The parasitic loranthus extracts (PLE) inhibits FAS reversibly and irreversibly and with an IC50 value of 0.48 μg/ml, appears to be the most potent inhibitor reported to date. PLE contains various potent inhibitors and may react with different sites on FAS. The irreversible inhibition exhibits a time-dependent biphasic process including a speedy fast-phase during the initial several minutes. The fast-phase inhibition seems to be caused by some potent but low-concentration component(s) in the extracts. In addition, we have found that avicularin existing in this herb can potently inhibit FAS. This glycosylated flavonoid and quercetin play an effective role in inhibiting FAS by parasitic loranthus.
Introduction
Animal FAS is an important enzyme participating in energy metabolism. This multifunctional enzyme consists of two identical polypeptides, each carrying seven functional domains, that are juxtaposed to form two centers for the synthesis of palmitic acid from acetyl- and malonyl-CoA in the presence of NADPH Citation1-3.
Recent studies have reported that FAS is involved in various human disorders including obesity and cancer. Mice treated with C75, a synthetic FAS inhibitor [Citation4], have reduced feeding and dramatic loss in weight. This is due to inhibition of FAS that results in accumulation of the substrate malonyl-CoA, the latter appearing to inhibit the expression of neuropeptide Y that promotes ingestion [Citation5,Citation6]. Thus, FAS has been proposed as a potential target for appetite and weight control [Citation5,Citation7]. It has also been reported that high levels of FAS are expressed in human cancer cells and inhibition of FAS is cytotoxic to human cancer cells [Citation8,Citation9].
Based on the above studies, FAS inhibitors obviously have positive effects on weight-loss therapy and antineoplastic treatment. A paucity of FAS inhibitors has been discovered so far. C75 and the natural product cerulenin are the early reported inhibitors which can irreversibly inhibit the ketoacyl synthase of FAS [Citation2,Citation4,Citation10,Citation11]. Wang et al. found that gallated catechins from green tea inhibited FAS reversibly and irreversibly, mainly by targeting the β-ketoacyl reductase domain [Citation12,Citation13]. However, their inhibitory potency is not very great, with an IC50 value of around 20 μg/ml. Another type of inhibitor are the flavonoid compounds, the strongest among which showed a IC50 value of around 1 μg/ml [Citation14]. However, they lack the irreversible inhibition component and the weight-loss functions. Weak inhibitory potency has limited the application of these FAS inhibitors.
Recently some herbal extracts have been screened as natural FAS inhibitors [Citation15]. Parasitic loranthus, a traditional herbal medicine, is commonly used in anti-obesity prescriptions and also used to lower blood pressure [Citation16]. Our previous report showed that parasitic loranthus exhibited weight-reducing effects on rats and could strongly inhibit FAS [Citation15], which focussed our attention on this herb for further studies.
In this paper, we have determined the inhibitory characteristics and kinetic parameters to explain how FAS is potently inhibited by extracts of Taxillus chinensis (DC.) Danser, which is the official species of parasitic loranthus according to the Pharmacopoeia [Citation17]. The inhibition by PLE consists of a potent reversible and a fast irreversible inhibition. The IC50 value is 0.48 μg/ml, so that its inhibitory ability is higher than any of the known FAS inhibitors. PLE contains various potent FAS inhibitors and may react with different sites on FAS. In addition, we have discovered that avicularin, a major component in this herb, can potently inhibit FAS.
Materials and methods
Materials
The herb parasitic loranthus was collected in Yunnan Province of southwestern China during August and September 2003. The herb was identified as Taxillus chinensis (DC.) Danser (LORANTHACEAE) by senior botanists at Kunming Institute of Botany, Chinese Academy of Sciences. Conforming to the pharmacopoeia [Citation17], this plant was authenticated for medical use. Avicularin, quercitrin, hyperoside and isoquercitrin were purchased from Delta Information Center for Natural Organic Compounds (Anhui, China) and was dissolved in ethanol-DMSO solvent (V:V = 1:1). Acetyl-CoA, malonyl-CoA and NADPH were purchased from Sigma-Aldrich Co. (St. Louis, Mo., USA). All other chemicals and reagents were local products of analytical grade.
Preparation of plant extracts
The stems of parasitic loranthus were ground into a powder. The powder sample was extracted with 50% ethanol in the ratio 1:20 (W/V) at room temperature for 4 h. Fifty percent ethanol was found as the optimum solvent for extraction of parasitic loranthus for provision of the most potent inhibitory ability on FAS. The extracts were centrifuged at 4000 × g for 15 min, and the 50% ethanolic supernatant was used in the subsequent experiments as PLE. The dry weight of 1 ml PLE was 13.0 ± 0.42 mg.
Preparation of FAS and substrates
The preparation, storage and use of FAS from duck liver were performed as described previously [Citation18]. The purified FAS was homogeneous on polyacrylamide gel electrophoresis in the presence and absence of SDS. The enzyme and substrate concentrations were determined by spectrophotometry following the extinction coefficients referred to in a previous report [Citation18].
Assay of FAS activity
Overall reaction (OA) activity of FAS was determined with an Amersham Pharmacia Ultrospec 4300 pro UV–Vis spectrophotometer at 37°C by detecting the decrease in NADPH absorption at 340 nm. The assay system contained 100 mM KH2PO4–K2HPO4 buffer (pH 7.0), 1 mM EDTA, 1 mM dithiothreitol, 3 μM acetyl-CoA, 10 μM malonyl-CoA and 35 μM NADPH in a total volume of 2.0 ml, and the reaction was initiated by the addition of 20 nM FAS as previously described [Citation18,Citation19].
The ketoacyl reduction (KR) activity of FAS was also determined at 37°C by measuring the change in NADPH absorption at 340 nm. The KR reaction mixture contained 40 mM ethyl acetoacetate, 35 μM NADPH, 1 mM EDTA, 1 mM dithiothreitol and 20 nM FAS in 100 mM KH2PO4–K2HPO4 buffer (pH 7.0) [Citation20].
Assay of reversible and irreversible inhibition
Reversible inhibition was determined by adding the inhibitors to the reaction system before FAS initiated the reaction. FAS activities in the presence and absence of inhibitors were assigned as Ai and A0 respectively. Ai/A0 was the relative activity (R.A.). The final concentration of ethanol did not exceed 0.5% (V/V) in the reaction mixture and thus did not affect the FAS activity.
Time-dependent inhibition was determined as follows: the enzyme solution was mixed with the inhibitor, and aliquots of the mixture were used to measure the R.A. at predetermined time intervals. 50% ethanol was used instead of the inhibitor as the control. To determine the irreversible inhibition, aliquots of the mixture were loaded on a prepared 1.2 ml Sephedex G25 (coarse) column and centrifuged at 2000 × g for 45 s, then the fraction with the enzyme was collected. The free inhibitors, which are much smaller than FAS, stayed on the column. Then the R.A. was assayed as outlined above. All measurements except the assay for activity were carried out at 25°C.
Results
Reversible inhibition of FAS by PLE
PLE exhibited potent reversible inhibition on FAS. shows that 0.48 ± 0.07 μg/ml of PLE inhibited 50% of the OA activity of FAS; while 2.85 ± 0.38 μg/ml of PLE inhibited 50% of the KR activity of FAS. As FAS is a multifunctional enzyme which has seven functional domains [Citation21], we studied the inhibition kinetics and several kinetic parameters of PLE to identify its possible reaction sites on FAS. The double-reciprocal plot (See ) illustrated that PLE was a competitive inhibitor to acetyl-CoA (Ki value of 0.20 μg/ml). PLE and acetyl-CoA may compete for the same binding site of FAS. The inhibition with respect to the substrate malonyl-CoA was in a mixed competitive and non-competitive manner (See ). In addition, the IC50 value for KR activity was about six-fold higher than that of OA activity of FAS. The kinetic results showed that PLE inhibited KR of FAS in a competitive manner with respect to NADPH (Ki value of 0.92 μg/ml), whereas it inhibited OA of FAS in a mixed competitive and noncompetitive manner (See and ).
Figure 1 Inhibitory effects of PLE on FAS activity. The inhibition of the OA (•) and the KR (♦) of FAS in the presence of various concentrations of PLE were measured. The IC50 values were means of three experiments obtained from the figures.
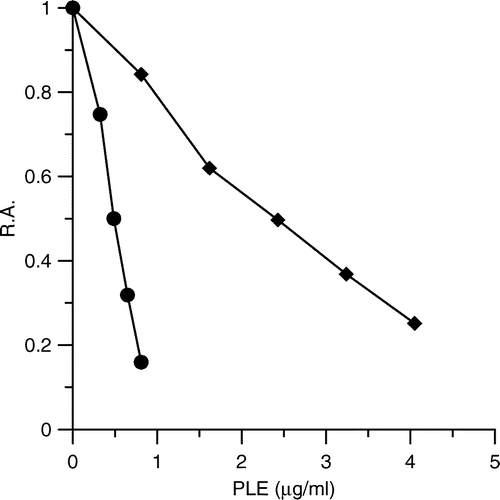
Figure 2 Double-reciprocal plots for inhibition of FAS by PLE. (A) The OA of FAS was measured. Acetyl-CoA was the variable substrate. The concentrations of malonyl-CoA and NADPH were fixed at 0.37 mM and 1.34 mM. The concentrations of PLE were: 0 μg/ml (•); 0.33 μg/ml (♦); 0.49 μg/ml (▴); 0.81 μg/ml (+) respectively. (B) The KR of FAS was measured. NADPH was the variable substrate. The concentrations of Acetyl-CoA and malonyl-CoA were fixed at 0.192 mM and 0.37 mM. The concentrations of PLE were: 0 μg/ml (•); 0.98 μg/ml (♦); 1.95 μg/ml (▴); 2.93 μg/ml (+) respectively.
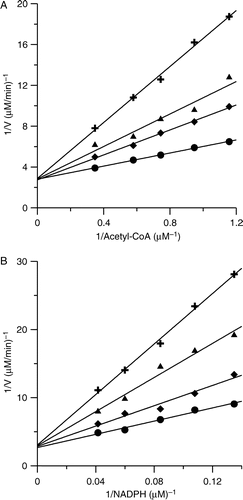
Table I. Inhibition pattern and inhibition parameters for the inhibition of FAS by PLE and avicularin with different substrates.
Irreversible inhibition of FAS by PLE
Our previous study revealed that PLE displayed a time-dependent change in its effect on the initial velocity of FAS [Citation15], implying that PLE inactivated the enzyme on the time scale of FAS turnover. The assay of time-dependent inhibition might be disguised by the initial potent reversible inhibition. In order to avoid this interference, a centrifuge chromatography was performed to remove the free inhibitors in the solution when measuring the irreversible inhibition. The irreversible inhibition of the OA activity of FAS exhibited a time-dependently biphasic process in the presence of 18 μg/ml of PLE. Under this concentration, ethanol did not affect FAS activity (data not shown). The semi-logarithmic plot showed two phases, and the apparent first-order rate constant kobs of 0.602 min− 1 for the fast phase (the contribution of slow phase was subtracted) and kobs of 6.03 × 10− 3 min− 1 for the slow phase were calculated (See ).
Figure 3 Semi-logarithmic plot of time-dependent irreversible inhibition of FAS in the presence of PLE. PLE (18 μg /ml) was mixed with FAS solution (0.15 μM) and aliquots of the mixture were loaded on a prepared 1.2 ml Sephedex G25 (coarse) column and centrifuged at predetermined time intervals when the enzyme fraction was collected to measure the R.A. of FAS. The plot represents Ln (R.A.) versus time. The fast-phase (▴) inhibition occurred from 0 to 5 min (the contribution of slow phase was subtracted), and the slow-phase (•) ranged from 5 to 80 min. The linear slopes of the two lines gave the apparent first-order rate constants.
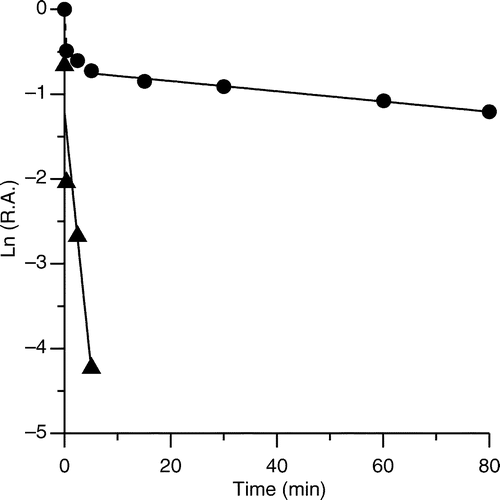
We have especially studied the fast-phase inactivation due to its extremely high inactivation velocity. The R.A. at 0.37 min and 2.5 min (with and without gel filtration) obviously declined as the concentration of PLE increased from to 18 μg/ml to 27 μg/ml (See ). While keeping PLE at 18 μg/ml and varying FAS concentration, the R.A value showed a correlated change (See ). Therefore, the degree of fast-phase irreversible inhibition was PLE and FAS concentration-dependent.
Figure 4 Degree of FAS inhibition by different concentration of PLE at 0.37 min and 2.5 min. (A) FAS solutions (0.15 μM) were mixed with PLE at 18 μg/ml and 27 μg/ml respectively. Aliquots of the mixture with or without gel filtration on Sephedex G25 were taken to assay the R.A. of FAS. FAS mixed with the solvent was used as control (white bar). The R.A. at 0.37 min (black bar) and 2.5 min (forward slash bar) are shown respectively. FG = Gel Filtration; NOGF = No Gel Filtration. (B) PLE (18 μg/ml) was mixed with FAS solutions of 0.15 μM and 0.82 μM. The R.A. without gel filtration at 0.37 min (black bar) and 2.5 min (forward slash bar) are shown. FAS mixed with the solvent was used as control (white bar). Each histogram was the mean of three experiments.
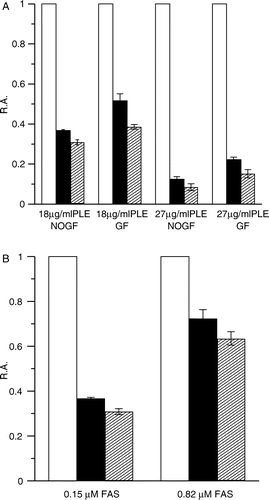
Furthermore, we performed substrate protection experiments and found that the irreversible inhibition for the OA of FAS was not protected by pre-adding any of the three substrates (data not shown), which indicated that the reaction sites of PLE for irreversible inhibition were not the binding sites of these substrates.
Inhibition of FAS by some glycosylated flavonoids
According to the literature, flavonoids such as quercetin and avicularin, are the major components of parasitic loranthus [Citation22]. shows that avicularin was a strong FAS inhibitor with an IC50 value of 6.15 ± 0.29 μM (2.67 ± 0.12 μg/ml) for the OA of FAS. Through kinetic studies, its inhibition was characterized as a competitive type with respect to acetyl-CoA and mixed type (competitive and noncompetitive pattern) to both malonyl-CoA and NADPH (See ). The inhibition of KR of FAS by avicularin was found to be very weak with an IC50 value < 200 μM. It is strongly implied that the effects of avicularin on FAS are unrelated to the inhibition of the β-ketoacyl reductase domain. In addition, we also measured the inhibition by some other common quercetin-derived glycosides. Quercitrin, hyperoside and isoquercitrin possess a rhamnose, a galactose and a glucose at the C3 of quercetin, respectively (See ). The reversible inhibition on the OA of FAS by these quercetin-derived glycosides was less potent than that by avicularin.
Table II. The IC50 values of some glycosylated flavonoids on the OA and KR of FAS.
Discussion
Our study demonstrates that PLE is a very potent inhibitor with reversible and time-dependent irreversible inhibition of FAS in vitro. The present work shows that the IC50 value of PLE is 0.48 μg/ml, which is about 40-fold lower than that of cerelenin (20 μg/ml) [Citation10] and epigallocatechin gallate (24 μg/ml) [Citation12]. Due to the potential of FAS as a drug target, FAS inhibitors show a valuable application in the therapy of obesity and cancer. The utility as a drug of cerelenin and epigallocatechin gallate has been limited due to their high IC50 values. Though as a herbal extract, PLE is the first reported inhibitor with an IC50 value less than 1 μg/ml. This great inhibitory potential on FAS makes PLE a favorable candidate as an anti-obesity or anti-cancer drug.
The IC50 value of 0.48 μg/ml for extracts is equivalent to 1.83 μg/ml for dry herb weight, which is the lowest among the traditional Chinese herb extracts that we examined. We have previously published that one species of parasitic loranthus inhibited FAS with the IC50 value of 12 μg/ml [Citation15]. This difference results from the different botanical sources of parasitic loranthus. We have found that different species of parasitic loranthus exhibited different inhibitory abilities on FAS and the species from the genus Taxillus were the best inhibitors (data not published).
We have further investigated the reversible inhibition of PLE by kinetic studies. PLE is assumed to contain multi-inhibitors that bind to different reaction sites on FAS. To the substrate acetyl-CoA, PLE showed competitive inhibition on the OA of FAS. To NADPH, PLE inhibited the NADPH-dependent KR step of FAS in a competitive manner, whereas it inhibited OA of FAS in a mixed competitive and noncompetitive manner. The competitive component may rely on the competition with NADPH mainly in the β-ketoacyl reductase domain, and the noncompetitive component may result from the influence of the reaction in those acetyl-CoA binding sites. These results suggest that PLE primarily acts on those sites where acetyl-CoA binds; and also act on the NADPH binding site of the β-ketoacyl reductase domain of FAS, which is an important but not dominant site. This conclusion is substantiated by the fact that the Ki value of PLE to NADPH in KR was about five-fold higher than that for acetyl-CoA in OA. This gap in Ki value is in accord with the gap in IC50 value between the OA and KR of FAS. Compared with FAS inhibitors reported previously, such as C75 [Citation4], epigallocatechin gallate [Citation12], galangal extracts [Citation23] and black tea preparations [Citation24], the kinetic type of PLE is different from that of these inhibitors.
PLE also shows irreversible inhibition on FAS based on the observation that most of the lost FAS activity could not be recovered after gel filtration. This inhibition exhibits a biphasic time course. The inactivation velocity in the fast-phase is very speedy and the kobs/[I] value at 18 μg/ml of PLE is 33.4 min− 1 mg− 1 ml, which is about eight-fold faster than that of C75 [Citation12]. Furthermore, the degree of fast-phase inhibition is dependent on PLE and FAS concentration. A higher concentration of PLE increases the degree of fast-phase inhibition, while a higher concentration of FAS decreases the degree of fast-phase inhibition. This implies that the speedy fast-phase inhibition results from the depletion of some component(s) which constitutes a small percentage of PLE content but contribute(s) greatly to the potent inhibition on FAS. The sites on FAS reacting with PLE for its irreversible inhibition are independent from that for the reversible inhibition, which is confirmed by the substrate protection experiments.
Parasitic loranthus has been recorded to be abundant in flavonoids, the main of which are quercetin and avicularin [Citation17,Citation25]. Avicularin shows potent reversible inhibition on FAS, and its potency is similar to that of quercetin [Citation14]. Neither exhibit irreversible inhibition of FAS. Therefore, avicularin and quercetin are, in part, the effective constitutes in PLE that inhibit FAS. This conclusion is also supported by kinetic results that show that the inhibition types of PLE to FAS are identical to that of avicularin and similar to that of quercetin (See ) [Citation14]. However, when considering the smaller IC50 value and the presence of irreversible inhibition by PLE, two possibilities arise: firstly, in PLE there is some unidentified component(s) that may have stronger inhibitory potency than avicularin and quercetin and can irreversibly inhibit FAS; secondly, various components with different reaction sites co-contribute to the inhibition of FAS.
We have examined the reversible inhibition by several quercetin-3-o-glucosides as well. Quercetin can inhibit FAS, but quercetin-3-o-rutinoside (rutin) cannot [Citation14]. Avicularin with a five-membered sugar ring connecting to quercetin, exhibits similar inhibitory ability to FAS as quercetin; whereas other glycosylated quercetins possessing a six-membered sugar ring show much weaker inhibitory ability. This suggests that the 3-OH in the C ring of flavonoids is not indispensable for inhibition against FAS and the size of the glycoside substituent at the 3-position seems to be crucial. The larger size of this group may result in a steric block that consequently undermines inhibitory ability.
In this paper, we describe the potency of PLE in inhibiting FAS, point out the possible sites on FAS reacting with PLE and analyze some components responsible for the inhibitory effects. The high inhibitory potency of PLE on FAS as well as the obvious weight-reducing effects [Citation15] present a very promising perspective on the application of parasitic loranthus as an anti-obesity drug.
Abbreviations | ||
FAS: | = | fatty acid synthase |
PLE: | = | parasitic loranthus extracts |
OA: | = | overall reaction |
KR: | = | ketoacyl reduction |
Acknowledgements
This work is supported by Grant 30270324 of China National Science Foundation and the Procter & Gamble Company (Mason, OH, USA). We appreciate the assistance of Prof. Liangwei Zhong (Graduate University of Chinese Academy of Sciences, Beijing, China) in the completion of this manuscript.
References
- Wakil SJ, Stoops JK, Joshi VC. Fatty acid synthesis and its regulation. Annu Rev Biochem 1983; 52: 537–579
- Joshi AK, Witkowski A, Smith S. The malonyl/acetyltransferase and beta-ketoacyl synthase domains of the animal fatty acid synthase can cooperate with the acyl carrier protein domain of either subunit. Biochemistry 1998; 37(8)2515–2523
- Smith S. The animal fatty acid synthase: One gene, one polypeptide, seven enzymes. FASEB J 1994; 8(15)1248–1259
- Kuhajda FP, Pizer ES, Li JN, Mani NS, Frehywot GL, Townsend CA. Synthesis and antitumor activity of an inhibitor of fatty acid synthase. Proc Natl Acad Sci USA 2000; 97(7)3450–3454
- Loftus TM, Jaworsky DE, Frehywot GL, Townsend CA, Ronnett GV, Lane MD, Kuhajda FP. Reduced food intake and body weight in mice treated with fatty acid synthase inhibitors. Science 2000; 288(5475)2379–2381
- Bouchard C. Inhibition of food intake by inhibitors of fatty acid synthase. New Engl J Med 2000; 21: 1888–1889
- Mobbs CV, Makimura H. Block the FAS, lose the fat. Nat Med 2002; 8(4)335–336
- Kuhajda FP, Jenner K, Wood FD, Hennigar RA, Jacobs LB, Dick JD, Pasternack GR. Fatty acid synthesis: A potential selective target for antineoplastic therapy. Proc Natl Acad Sci USA 1994; 91(14)6379–6383
- Kuhajda FP. Fatty-acid synthase and human cancer: new perspectives on its role in tumor biology. Nutrition 2000; 16(3)202–208
- Vance D, Goldberg I, Mitsuhashi O, Bloch K. Inhibition of fatty acid synthatase by the antibiotic cerulenin. Biochem Biophys Res Commun 1972; 48: 649–655
- Shu IW, Lindenberg DL, Mizuno TM, Roberts JL, Mobbs CV. The fatty acid synthase inhibitor cerulenin and feeding, like leptin, activate hypothalamic pro-opiomelanocortin (POMC) neurons. Brain Res 2003; 985(1)1–12
- Wang X, Tian W. Green tea epigallocatechin gallate: A natural inhibitor of fatty-acid synthase. Biochem Biophys Res Commun 2001; 288(5)1200–1206
- Wang X, Song KS, Guo QX, Tian WX. The galloyl moiety of green tea catechins is the critical structural feature to inhibit fatty-acid synthase. Biochem Pharmacol 2003; 66(10)2039–2047
- Li BH, Tian WX. Inhibitory effects of flavonoids on animal fatty acid synthase. J Biochem (Tokyo) 2004; 135(1)85–91
- Tian WX, Li LC, Wu XD, Chen CC. Weight reduction by Chinese medicinal herbs may be related to inhibition of fatty acid synthase. Life Sci 2004; 74(19)2389–2399
- Xie YM, Wong WL. Reducing fat with traditional Chinese medicine. Symposium International Conference of Weight Reducing, Beijing 2000; 132
- National Pharmacopoeia Committee. Pharmacopoeia of the People's Republic of China2nd ed. Chemical Industry Press, Beijing 2000; 246
- Tian WX, Hsu RY, Wang YS. Studies on the reactivity of the essential sulfhydryl groups as a conformational probe for the fatty acid synthetase of chicken liver. Inactivation by 5,5′-dithiobis-(2-nitrobenzoic acid) and intersubunit cross-linking of the inactivated enzyme. J Biol Chem 1985; 260(20)11375–11387
- Soulie JM, Sheplock GJ, Tian WX, Hsu RY. Transient kinetic studies of fatty acid synthetase. A kinetic self-editing mechanism for the loading of acetyl and malonyl residues and the role of coenzyme A. J Biol Chem 1984; 259(1)134–140
- Tian WX, Cai H. The denaturation and inactivation of fatty acid synthase from duck liver in guanidine hydrochloride. Acta Biophys Sinica (Chinese) 1994; 10(1)6–12
- Wakil SJ. Fatty acid synthase, a proficient multifunctional enzyme. Biochemistry 1989; 28(11)4523–4530
- Zhou HH, Wu JR. Preliminary studies of Sang Ji Sheng and Hu Ji Sheng. J Guiyang Col Trad Chin Med 1999; 21(4)59–60
- Li BH, Tian WX. Presence of fatty acid synthase inhibitors in the rhizome of Alpinia officinarum hance. J Enz Inhib Med Chem 2003; 18(4)349–356
- Du YT, Wang X, Wu XD, Tian WX. Keemun Black tea extract contains potent fatty acid synthase inhibitor and reduces food intake and body weight of rats via oral intubation. J Enz Inhib Med Chem 2005, in press
- A Dictionary of Chinese Traditional Medicines. Shanghai Science and Technology Publishing House. 1997; Vol 3: 1970–1973