Abstract
Tamarix gallica, a hepatic stimulant and tonic, was examined for its ability to inhibit thioacetamide (TAA)-induced hepatic oxidative stress, toxicity and early tumor promotion response in male Wistar rats. TAA (6.6 mmol/kg body wt. i.p) enhanced lipid peroxidation, hydrogen peroxide content, glutathione S-transferase and xanthine oxidase with reduction in the activities of hepatic antioxidant enzymes viz., glutathione peroxidase, superoxide dismutase and caused depletion in the level of hepatic glutathione content. A marked increase in liver damage markers was also observed. TAA treatment also enhanced tumor promotion markers, ornithine decarboxylase (ODC) activity and [3H] thymidine incorporation into hepatic DNA. Pretreatment of rats orally with Tamarix gallica extract (25 and 50 mg/kg body weight) prevented TAA-promoted oxidative stress and toxicity. Prophylaxis with Tamarix gallica significantly reduced the susceptibility of the hepatic microsomal membrane for iron-ascorbate induced lipid peroxidation, H2O2 content, glutathione S-transferase and xanthine oxidase activities. There was also reversal of the elevated levels of liver marker parameters and tumor promotion markers. Our data suggests that Tamarix gallica is a potent chemopreventive agent and may suppress TAA-mediated hepatic oxidative stress, toxicity, and tumor promotion response in rats.
Introduction
Thioacetamide (TAA), originally used as a fungicide, is considered to be one of the most potent hepatotoxicants and carcinogenic agents[Citation1]. It has widely been used to produce varying grades of liver damage in rats including nodular cirrhosis, liver cell proliferation, production of pseudolobules, and parenchymal cell necrosis [Citation2]. TAA is considered to trigger a series of biochemical events in the liver that are linked to its tumor promoting effects. Such events include the increase in hepatic DNA synthesis, interruption of cellular calcium homeostasis, disruption of membranes of the endoplasmic reticulum, failure of amino acid incorporation into liver proteins and free radical generation in the liver [Citation3]. Studies have shown that TAA S-oxide and TAA S-dioxide are very reactive products of TAA metabolism, which are obligatory for the hepatotoxic effect of this compound [Citation4].
Disturbance of normal prooxidant-antioxidant equilibrium in the body leads to oxidative stress, which is characterized by the increased generation of reactive oxygen species. These oxyradicals play a stimulatory role in several diseases such as carcinogenesis [Citation5]. Carcinogenesis is a multistep process, which comprises three stages, viz., initiation, promotion and progression with each stage probably involving both genetic and epigenetic changes. Free radicals are known to play an important role in both initiation and promotion of multistage carcinogenesis. These reactive oxygen species can act as initiators or promoters, cause DNA-damage [Citation6], activate carcinogen [Citation7], alter the cellular antioxidant defense system and phase II metabolizing enzymes [Citation8]. Recently, more attention has been devoted to plant derived drugs and crude extract for the treatment of various diseases, because of their low toxicity and rich antioxidant properties. Chemoprevention is one of the strategies used to avert the metabolic disorders by environmental pollutants and oxidants. It has been suggested that the use of antimutagens and anticarcinogens in everyday life will be the most effective strategy for preventing and delaying human cancer and genetic diseases [Citation9]. Many compounds that show promise in protecting against cancer have been identified in our diet and in plant-based material [Citation10]. Plants and their constituents may act as detoxificants, anticarcinogens and as antiinitiators. From our own laboratory, we have evaluated several herbs, which are used in traditional medicine (Ayurveda and Unani-Tibb) for their anticarcinogenic and antioxidant properties against environmental carcinogens in different animal model systems Citation11-13.
Tamarix gallica is a gregarious bushy shrub of the family Tamaricaceae, commonly known as Jhau, and is widely distributed along marshy land and riverside regions of the world [Citation14]. It is one of the important constituents of Liv 52, an ayurvedic herbal formulation [Citation15]. Tamarix gallica is reported to be a hepatic stimulant and tonic [Citation15]. Mainly galls and manna (gummy exudation) are used for therapeutic purposes. Galls mainly used as astringent are given internally in dysentery and diarrhoea; pulverized galls mixed with vaseline are useful in piles and anal fissure; decoction applied to foul and sloughing ulcers and infusions are used as a gargle for sore throat. Manna is mainly used as mild laxative and expectorant [Citation14].
The major chemical constituents of Tamarix gallica are tannin (50%), tamarixin, tamauxetin, troupin, 4-methylcoumarin and 3,3'-di-O-methylellagic acid [Citation14]. Keeping in view the anti-inflammatory and antioxidant properties of Tamarix gallica, the present study was designed to assess the chemopreventive potential of Tamarix gallica on TAA-induced carcinogenesis - associated biochemical alterations in rat liver. For the present study, a methanolic extract of the plant was prepared that contained tannic acid, 4-methylcoumarin and 3,3'-di-O-methylellagic acid as the major active constituents. Co-TLC with authentic samples under a UV lamp was used to confirm the presence of these active compounds.
Materials and methods
Animals
Male albino rats of Wistar strains weighing 180–200 gm were housed in groups of six in large spacious polypropylene cages. Lighting was regulated to provide equal hours of light and dark. Animals were obtained from the central animal house facility of Jamia Hamdard, New Delhi, India. The animals were acclimatized to standard laboratory conditions (temperature 25 ± 10°C, relative humidity 50 ± 15%) one week prior to the actual commencement of the experiment. They were provided with standard food pellets (Hindustan Lever Ltd., India) and tap water ad libitum.
Chemicals
Thioacetamide, ornithine, pyridoxal phosphate, 2-mercaptoethanol, dithiothreitol, Tween-80, phenylmethylsulfonyl fluoride (PMSF), glutathione reduced (GSH), glutathione oxidized (GSSG), glutathione reductase (GR), nicotinamide adenine dinucleotide phosphate reduced (NADPH), bovine serum albumin (BSA), 1,2-dithio-bis-nitrobenzoic acid (DTNB), 1-chloro-2, 4-dinitrobenzene (CDNB), dichloroindophenol (DCIP), flavin adenine dinucleotide (FAD), thiobarbituric acid (TBA), xanthine, trichloroacetic acid (TCA), β-nicotinamide adenine dinucleotide (NADH), sodium pyruvate, α-ketoglutarate, α L-Alanine were obtained from Sigma Chemical Co. (St.Louis,MO,USA). [3H]-thymidine (sp.activity 82 Ci/mmol) and [1–14C]-ornithine were purchased from Amersham Corporation (UK). All other chemicals used were of the highest purity commercially available.
Plant material extraction
Dried aerial parts of the plant Tamarix gallica were procured from Hamdard Herbal Garden, Jamia Hamdard and authenticated by Prof. M. Iqbal, Department of Environmental Botany, Jamia Hamdard, New Delhi, India. A reference specimen has been deposited in the herbarium of the Department of Medical Elementology and Toxicology. Freshly collected plant parts were shade-dried and coarsely powdered in a grinder. Powdered dried plant (500 g) was extracted with 2000 ml petroleum ether (60–80°C). The petroleum ether extract was discarded. The residue was then successively extracted with benzene, chloroform and acetone using a Soxhlet apparatus. Finally, the residue was extracted with methanol. The methanol extract was recovered and evaporated to dryness by distillation under reduced pressure in a rotatory evaporator (Buchi Rotavapour, Switzerland). The concentrated methanol fraction obtained (42 g) was stored at 4°C and was dissolved in distilled water to make the required doses. The preliminary testing of the extract was evaluated in vitro against calf thymus DNA - sugar damage and Cyt P450 (data not shown).
Experimental regimen
To study the effect of prophylactic administration of the methanolic extract of Tamarix gallica extract on TAA-mediated oxidative stress, 30 male wistar rats were randomly divided into five groups, each comprising six animals.
Group I animals served as negative control and were kept under normal conditions with pellet diet and water ad libidum.
Group II animals served as TAA-treated control were given intraperitoneally a single dose of TAA (6.6 mmol/kg body wt) freshly dissolved in 0.9% NaCl.
Group III animals served as an experimental group and were pretreated with Tamarix gallica extract at a doses of 25 mg/kg body weight through oral intubation (p.o) for seven consecutive days.
Group IV animals served as an experimental group and were pretreated with Tamarix gallica extract at a dose of 50 mg/kg body weight through oral intubation (p.o) for seven consecutive days.
Group V animals served as a plant treated control group and received only Tamarix gallica extract at a high dose level of 50 mg/kg body weight through oral intubation (p.o) for seven consecutive days.
The animals of group III and IV were given a single intraperitoneal injection of TAA (6.6 mmol/kg body wt) freshly dissolved in 0.9% NaCl forty-five min after the last dose of plant extract.
Twenty four hours after TAA treatment the overnight fasted animals were sacrificed by cervical dislocation. Blood was collected by cardiac puncture, allowed to clot in centrifuge tubes at 4°C and then centrifuged at 3000 × g for 15 min to separate the serum. The serum was then used for the enzyme assays (SGOT, SGPT, GGT and LDH). Liver was excised quickly washed in ice-cold saline (0.9%), kept in ice and processed for subcellular fractionation for the biochemical estimations.
To study the effect of prophylactic administration of the methanolic extract of Tamarix gallica extract on TAA-mediated hepatic ornithine decarboxylase (ODC) activity the experimental protocol was exactly similar to that described for oxidative stress. All the animals were sacrificed after 12 h of TAA injection by cervical dislocation and livers were removed and quickly processed for subcellular fractionation for the estimation of ODC activity.
To study the effect of prophylactic administration of the methanolic extract of Tamarix gallica extract on TAA –mediated [3H]-thymidine incorporation into hepatic DNA synthesis, the experimental protocol was exactly similar to that described for oxidative stress and ODC activity, except that animals were not killed at 24 h. 12 hours after TAA injection, the animals of all the groups were administered [3H]-thymidine (25 μCi/0.2 ml saline/100 g) as an i.p injection 2 h before sacrifice. Animals were sacrificed and livers were excised and homogenized in chilled distilled water for further processing, the quantitation of hepatic DNA synthesis and separation of DNA.
Postmitochondrial supernatant (PMS) and microsome preparation
Liver tissues from the post mortem animals of each group were quickly excised, washed in ice-cold saline (0.9%) and kept in ice. A 10% homogenate was prepared in chilled phosphate buffer (0.1 M, pH 7.4) containing KCl (1.17%) using a Potter Elvehjem homogenizer. The homogenate was filtered through muslin cloth, and was centrifuged at 800 g for 5 min at 4°C by an Eltek Refrigerated Centrifuge (model RC 4100 D) to separate the nuclear debris. The obtained aliquot was centrifuged at 12000 g for 20 min at 4°C to obtain PMS, which was used as a source of enzymes. A portion of the PMS was centrifuged at 100,000 × g for 1 h at 4°C in an ultracentrifuge (Beckman, L7-55). The pellet was washed with phosphate buffer (0.1 M, pH 7.4) containing KCl (1.17%). All the biochemical estimations were completed within 24 h of sampling.
Biochemical assays
Ornithine decarboxylase activity
ODC activity was determined by utilizing 0.4 ml hepatic 105,000 × g supernatant fraction per assay by measuring the release of 14CO2 from DL [14C]-ornithine by the method of O'Brien et al. [Citation16], as described by Athar et al. [Citation17]. The liver was homogenized in Tris-HCl buffer (pH 7.5,50 mM) containing EDTA (0.1 mM), pyridoxal phosphate (0.1 mM), PMSF (1.0 mM), 2-mercaptoethanol (1.0 mM), dithiothreitol (0.1 mM) and Tween 80 (0.15) at 4°C using a polytron homogenizer (Kinematica AGPT 3000). Homogenized samples were first centrifuged at 9000 × g for 20 min at 4°C in an Eltek Refrigerated Centrifuge (model RC 4100 D) and the resulting post-mitochondrial supernatant (PMS) then centrifuged at 100,000 × g for 1 h at 4°C in an ultracentrifuge (Beckman, L7-55) to obtain the cytosolic fractions.
In brief, the reaction mixture contained 400 μl enzyme and 0.095 ml co-factor mixture containing pyridoxal phosphate (0.32 mM), EDTA (0.4 mM), dithiothreitol (4.0 mM), ornithine (0.4 mM), Brit 35 (0.02%) and [14C] ornithine (0.05 μCi) in a total volume of 0.495 ml. After adding buffer and co-factor mixture to blank and other tube, the tubes were covered immediately with a rubber cork containing 0.2 ml ethanolamine and methoxyethanol mixture in the central well and kept in a water-bath at 37°C. After 1 h of incubation, the enzyme activity was arrested by injecting 1.0 ml citric acid solution (2.0M) along the sides of the glass tubes and the incubation was continued for 1 h to ensure complete absorption of 14CO2. Finally, the central wells were transferred to a vial containing 2 ml ethanol and 10 ml toluene-based scintillation fluid, followed by radioactive counting in a liquid scintillation counter (LKB-Wallace-1410). ODC activity was expressed as pmol 14CO2 released/h/mg protein. Protein in all samples was estimated by the method of Lowry et al. [Citation18] using bovine serum albumin as standard.
Estimation of hepatic DNA synthesis
The isolation of hepatic DNA and incorporation of [3H] thymidine in DNA was performed using the method of Smart et al. [Citation19], as described by Athar and Iqbal [Citation20]. The liver homogenate (105,w/v) prepared in ice cold distilled water was washed with chilled trichloroacetic acid (TCA) (5%) and incubated with chilled perchloric acid (PCA) (10%) at 4°C overnight. The incubate was then centrifuged and the precipitate washed with chilled PCA (5%) then dissolved in warm PCA (10%) followed by incubation in a boiling water-bath for 30 min, and filtered through Whatman 50. The filtrate was used for [3H] counting in a liquid scintillation counter (LKB-Wallace-1410) after adding the scintillation fluid. The amount of DNA in the filtrate was estimated by the diphenylamine method of Giles and Myers [Citation21]. The amount of [3H] thymidine incorporation was expressed as dpm/μg DNA.
GSH estimation
The GSH in liver was determined by the method of Jollow et al. [Citation22]. One ml of PMS fraction was mixed with 1 ml of sulphosalcylic acid (4%) and the samples incubated at 4°C for at least 1 h and then subjected to centrifugation at 1200 × g for 15 min at 4°C.The assay mixture contained aliquot, phosphate buffer 0.1 M, pH 7.4 and 4 mg/1 ml DTNB in a total volume of 3.0 ml. The yellow color developed was read immediately at 412 nm and was calculated as nmol GSH/g tissue.
Glutathione peroxidase activity
Glutathione perooxidase activity was assayed by the method of Mohandas et al. [Citation23], as described by Athar and Iqbal [Citation20]. The assay mixture consisted of phosphate buffer, 0.1 M, pH 7.4 1 mM EDTA, 0.2 mM NADPH, 1 mM sodium azide, 1.0 IU/ml glutathione reductase, 0.25 mM H2O2 and PMS (10%, w/v) in a total volume of 2.0 ml. The activity was recorded at 340 nm at 25°C and was calculated as nmol NADPH oxidized min− 1 mg protein− 1 by using a molar extinction coefficient of 6.22 × 103 /M/cm.
Glutathione S-transferase activity
Glutathione S-transferase (GST) activity was assayed by the method of Habig et al. [Citation24], as modified by Athar et al. [Citation25]. The assay mixture consisted of phosphate buffer 0.1M, pH 7.4, 1 mM GSH, 1 mM CDNB, and cytosolic fraction (10% w/v) in a total volume of 3.0 ml. Enzyme activity was recorded at 340 nm and the activity was calculated as nmol CDNB conjugate formed min− 1 mg protein− 1 using a molar extinction coefficient of 9.6 × 103 /M/cm.
Xanthine Oxidase activity
XO which catalyzes the conversion of xanthine to uric acid has a characteristic absorption at 290 nm. It was assayed by the method of Athar et al. [Citation26]. The reaction mixture, containing 0.2 ml PMS (10% w/v) diluted to 1 ml with phosphate buffer 0.1 M, pH 7.4 was incubated for 5 min at 37°C. The reaction was started by adding 0.1 ml xanthine and the reaction mixture was kept at 37°C for 20 min. and the reaction was terminated by addition of 0.5 ml ice-cold PCA (10%). After 10 min, 2.5 ml distilled water was added and the mixture was centrifuged at 4000 × g for 10 min. The absorbance of clear supernatant was read at 290 nm. The activity was expressed as uric acid formed /mg protein.
Lipid peroxidation estimation
Microsomal lipid peroxidation was estimated by following the method of Wright et al. [Citation27]. The assay mixture consisted of phosphate buffer 0.1M, pH 7.4, 100 mM ascorbic acid, 100 mM ferric chloride and microsomes (10% w/v). The reaction mixture was incubated at 37°C in a shaking water bath for 1 h. The reaction was stopped by the addition of 10% TCA following the addition of 0.67% TBA, all the tubes were placed in a boiling water bath for 20 min. The tubes were transferred to an ice bath and then centrifuged at 2500 × g for 10 min. The amount of TBARS formed in each sample was assessed by measuring the absorbance of the supernatant at 535 nm. The results were expressed as nmol TBARS formed/hr/gm tissue at 37°C by using a molar extinction coefficient of 1.56 × 103 /M/cm.
Hydrogen peroxide content
Hydrogen peroxide was assayed by H2O2 mediated horseradish peroxidase –dependent oxidation of phenol red by the method of Pick and Keisari [Citation28]. 2.0 ml of microsome was suspended in 1.0 ml of solution containing phenol red (0.28 nm), horse radish peroxidase (8.5 units), dextrose (5.5 nm) and phosphate buffer (0.05 M, pH 7.0) and incubated at 37°C for 60 min. The reaction was stopped by addition of 0.01 ml of NaOH (10 N) and then centrifuged at 800 × g for 5 min. The absorbance of the supernatant was recorded at 610 nm against a reagent blank. The quantity of H2O2 produced was expressed as nmol H2O2/g tissue/h.
Superoxide dismutase activity
Superoxide dismutase (SOD) activity was assayed by the method of Stevens et al. [Citation29]. The assay mixture consisted of glycine buffer 50 mM, pH 10.4, 20 mg/ml epinephrine solution, and cytosolic fraction (10% w/v) in a total volume of 1.0 ml. Enzyme activity was recorded at 480 nm and the activity was calculated as uM epinephrine oxidized /min/mg protein.
Lactate dehydrogenase (LDH) activity
LDH activity was estimated in serum by the method of Korenberg et al. [Citation30]. The assay mixture consisted of serum, 0.02 M NADH, 0.01 M sodium pyruvate, phosphate buffer 0.1 M, pH 7.4 and distilled water in a total volume of 3 ml. Enzyme activity was recorded at 340 nm and activity was calculated as nmol NADH oxidized/min/mg protein.
Serum oxaloacetate and pyruvate transaminase activity (SGOT & SGPT)
Serum oxaloacetate and pyruvate transaminase (SGOT and SGPT) activities were determined by the method of Reitman and Frankel [Citation31]. Each substrate (0.5 ml) (2 mM α-ketoglutarate and either 200 mM α L-Alanine or L- Aspartate) was incubated for 5 min at 37°C in a water bath. Serum (0.1 ml) was then added and the volume was adjusted to 1.0 ml with phosphate buffer 0.1M, pH 7.4. The reaction mixture was incubated for exactly 30 and 60 min for GPT and GOT, respectively. Then to the reaction mixture, 0.5 ml of 1 mM DNPH was added and left for another 30 min at room temperature. Finally, the color was developed by addition of 5.0 ml of NaOH (0.4 N) and product absorbance read at 505 nm.
γ-Glutamyl transpeptidase (GGT) activity
γ-Glutamyl transpeptidase activity (GGT) activity was determined by the method of Orlowski and Meister [Citation32] using γ-glutamyl p-nitroanilide as substrate. The reaction mixture contains 0.2 ml serum, which was incubated with 0.8 ml substrate mixture (containing 4 mM γ-nitroanilide, 40 mM glycylglycine and 11 mM MgCl2 in 185 mM Tris HCl buffer, pH 8.25) at 37°C. Ten minutes after initiation of the reaction, 1.0 mL 25% TCA was added and mixed to terminate the reaction. The solution was centrifuged and the supernatant fraction aborbance read at 405 nm. Enzyme activity was calculated as nmol p-nitroaniline formed/min/mg protein.
Protein estimation
Protein concentration in all samples was determined by the method of Lowry et al. [Citation18].
Stastical analysis
The level of significance between different groups was based on analysis of variance test (ANOVA) followed by the Dunnett's t-test.
Results
The effect of prophylactic treatment of animals with Tamarix gallica on TAA-mediated hepatic glutathione content and on the activities of its metabolizing enzymes i.e. glutathione peroxidase (GPx), glutathione S-transferase (GST) and xanthine oxidase (XO) is shown in . TAA treatment resulted in the depletion of hepatic glutathione and activity of glutathione peroxidase (GPx) to a level of 56% and 34% respectively and elevation in the activities of hepatic glutathione S-transferase (GST) and xanthine oxidase (XO) to a level of 144% and 396% respectively, compared with that of the saline treated control group. However, pretreatment of animals with Tamarix gallica at 25 and 50 mg/kg body wt. partially recovered glutathione content, glutathione peroxidase (GPx), glutathione S-transferase (GST) and xanthine oxidase (XO) levels to 17–45%, 6–19%, 12–27% and 34–60%, respectively as compared with TAA-treated control groups.
Table I. Effect of prophylactic treatment of animals with Tamarix gallica on TAA-induced alterations in hepatic glutathione content, glutathione metabolizing and antioxidant enzymes.
TAA treatment enhanced susceptibility of hepatic microsomal membrane for iron-ascorbate induced lipid peroxidation to about 165% and hydrogen peroxide content to 227% whereas it caused a reduction in the activity of superoxide dismutase (SOD) to 43% as compared with saline treated control (). However, Tamarix gallica pretreatment dose-dependently inhibited enhancement of TAA-mediated lipid peroxidation and H2O2 content and decreased the level of superoxide dismutase (SOD). At higher dose (50 mg/kg body wt.) lipid peroxidation was reduced to 39% and H2O2 content to 48% while the level of superoxide dismutase (SOD) was increased to 18% as compared with TAA-treated control. At lower dose (25 mg/kg body wt.) lipid peroxidation was reduced to 10% and H2O2 content to 29% while the level of superoxide dismutase (SOD) was increased to 11% as compared with the TAA-treated control.
Table II. Effect of prophylactic treatment of animals with Tamarix gallica on TAA-mediated alterations in hepatic lipid peroxidation, hydrogen peroxide content and superoxide dismutase enzyme.
The effect of pretreatment of animals with Tamarix gallica on TAA-mediated alterations in the activities of serum enzymes oxaloacetate and pyruvate transaminase (GOT and GPT), lactate dehydrogenase (LDH) and γ-glutamyl transpeptidase (GGT) are shown in (A and B). TAA treatment leads to about 174%, 259%, and 150% and 266% enhancement in serum GOT, GPT, LDH and GGT respectively as compared with a saline treated control. Pretreatment with T.gallica resulted in the 18–28%, 33–52%, 13–28% and 16–39% reduction in the values of serum GOT, GPT, LDH and GGT, respectively, as compared with the TAA-treated control at lower (25 mg/kg body wt.) and higher (50 mg/kg body wt.) doses of T.gallica extract.
Figure 1 (A) Effect of pretreatment of rats with Tamarix gallica (TG) on thioacetamide (TAA) mediated enhancement in serum oxaloacetate and pyruvate transaminase (GOT and GPT). (B) Lactate dehydrogenase (LDH) and γ-Glutamyl transpeptidase activity (GGT) activity in rats. (Each value represents means ± S.E.; n = 6. *P < 0.001 compared to corresponding value for saline treated control. #P < 0.1, ##P < 0.01 and ###P < 0.001 compared with the corresponding value for treatment with thioacetamide. TG -25 and TG- 50 represent oral administration of Tamarix gallica of 25 and 50 mg/kg body weight respectively.
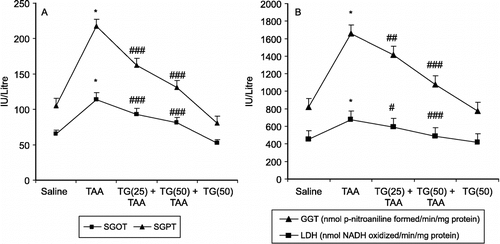
The effect of pretreatment of rats with Tamarix gallica on TAA-mediated induction of hepatic ornithine decarboxylase (ODC) activity is shown in (A). TAA treatment resulted in 449% induction in the ornithine decarboxylase (ODC) activity as compared with saline treated control. The pretreatment of rats with Tamarix gallica at a dose of 50 mg/kg body wt. caused 20% and at a dose of 100 mg/kg body wt caused 64% recovery of ornithine decarboxylase (ODC) activity as compared with the TAA-treated control group. The effect of prophylaxis of rats with Tamarix gallica on TAA-mediated enhancement in the incorporation of [3H] thymidine into hepatic DNA is shown in (B). Treatment with TAA alone caused 468% increase in the incorporation of [3H] thymidine into hepatic DNA. At the lower dose of Tamarix gallica (25 mg/kg body wt) the enhancement was reduced to 17% and at the higher dose (50 mg/kg body wt) the enhancement was reduced to 67% as compared with TAA-treated group.
Figure 2 (A) Effect of pretreatment of rats with Tamarix gallica (TG) on thioacetamide (TAA)-mediated enhancement in (A) ornithine decarboxylase (ODC) activity and (B) 3H incorporation in hepatic DNA in rats. (Each value represents means ± S.E.; n = 6.*P < 0.001 compared to corresponding value for saline treated control. #P < 0.05 and ##P < 0.001 compared with the corresponding value for treatment with thioacetamide. TG -25 and TG -50 represent oral administration of Tamarix gallica of 25 and 50 mg/kg body weight respectively.
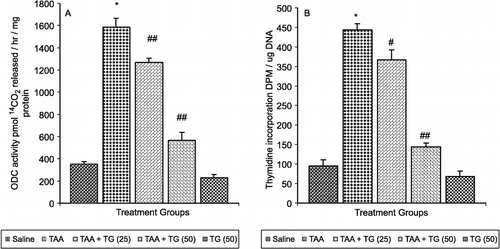
Discussion
Research has demonstrated that reactive oxygen species lead to damage in various cells in the body and destroy or inhibit their ability to function as normal cells. Therefore, there is a need to explore compounds which can limit the effects of these reactive oxygen species. For that reason various vegetables and fruits rich in antioxidants have been demonstrated to play key roles in reversing the effect of reactive oxygen species and restoring the proper function of tissues Citation33-34. Plants have played a significant role in the prevention and treatment of diseases since prehistoric times [Citation35]. At present there is substantial evidence to show that chemical mutagenesis and carcinogenesis can be inhibited by a large number of naturally occurring compounds of plant origin.
The chemical constituents and medicinal uses of Tamarix gallica have been studied widely. The phytochemical analysis of this plant shows that it is rich in antioxidants such as tannin (50%), tamarixin, tamauxetin, troupin, 4-methylcoumarin and 3,3'-di-O-methylellagic acid [Citation14]. The methanolic fraction was used in this study since preliminary in vitro tests have shown maximum protective effects when compared with other fractions of the plant. The present study clearly demonstrated that Tamarix gallica restored thioacetamide-induced alterations in enzyme activity which are considered to be responsible for oxidative stress and the tumor promotion response in the liver of wistar rats.
Oxidative stress plays an important role in TAA-induced liver injury. Protective agents such as plants, exert their action against oxidant- mediated damage by impairment of lipid peroxidation either through decreased production of free radical derivatives or due to the antioxidant activity of the protective agents. Tamarix gallica prevented the TAA – mediated inhibition of hepatic glutathione content. The fact that Tamarix gallica can maintain the level of reduced glutathione content in liver suggests that it acts by preventing the reversible binding of TAA to some important cellular protein, a mechanism similar to that involved in the protective action of cysteine [Citation36]. Tamarix gallica diminishes TAA – mediated inhibition of the activities of antioxidant enzymes such as superoxide dismutase (SOD) and glutathione peroxidase (GPx) and depletion of hydrogen peroxide content was observed that might have resulted in the degeneration of TAA-mediated reactive species. It may also act as a modifier of the oxidant response of liver and therefore its antioxidant potential may have a counteracting effect on oxidant-mediated generation of oxidative stress. There was a dose-dependent decrease in the TAA-mediated susceptibility of the hepatic microsomal membrane for iron-ascorbate induced lipid peroxidation through decreased production of free radicals as evidenced by ameliorated malanodialdehyde levels. The plant has also been shown to diminish glutathione S-transferase (GST) activity, which results in degradation of electrophilic metabolites generated by TAA.
An obvious sign of hepatic injury is leakage of cellular enzyme into the plasma [Citation37]. When the plasma membrane is damaged, a variety of enzymes normally located in the cytosol are released into the blood stream. Their estimation in the serum is a useful quantitative marker for the extent and type of hepatocellular damage [Citation38]. The methanolic extract of Tamarix gallica used in the study preserved the structural integrity of the hepatocellular membrane in a dose-dependent manner as evident from the protection provided as compared to the enzyme level in the hepatotoxin-treated rats.
Induction of ornithine decarboxylase (ODC), xanthine oxidase (XO) activities and DNA synthesis are used as a biochemical marker to evaluate the tumor-promoting potential of an agent. ODC is the rate – limiting enzyme of polyamine biosynthesis and the level of ODC is elevated at the time of acceleration of cell proliferation and development [Citation39]. An enhancement in both hepatic ODC activity and [3H]-thymidine incorporation suggests a strong proliferative and tumor promoting potential of TAA in liver. The inhibitory effect of Tamarix gallica at both doses reduced the TAA-mediated induction in ODC activity; DNA synthesis and xanthine oxidase (XO) activity suggesting the antitumor promoting potential of Tamarix gallica.
In summary, the data of this study suggest that Tamarix gallica facilitates a number of responses relevant to cancer chemoprevention. Tamarix gallica shows the presence of tannic acid (more than 50%) as major active principle. Tannins are a diverse group of compounds. Their antimutagenic and anticarcinogenic activity is variously described in the literature Citation40-41. Tannins have a beneficial physiological property as a scavenger of reactive oxygen intermediates [Citation42]. Tannic and ellagic acids are promising molecules among natural antioxidants Citation43-44. In particular, tannins are reported to exert antimutagenic and anticarcinogenic action through: (i) inhibition of procarcinogen metabolism [Citation45] and reduction of nitrosoamine-induced tumorigenesis in mice [Citation44] (ii) regulation of the cell cycle in progression and apoptosis in cancer cells (iii) scavenging of radical species induced by H2O2 in cultured cells [Citation46]. Coumarin and 4-methylcoumarin have been proven to be inhibitors of mammary carcinogenesis in the rat. Ellagic acid and 3,3'-di-O-methylellagic acid are well documented antioxidants and are known to protect against carbon tetrachloride induced liver toxicity [Citation47]. Based on these results it can be suggested that the tannins in addition to the other minor constituents (4-methylcoumarin, 3,3'-di-O-methylellagic acid, tamarixin, tamauxetin, troupin etc) are responsible for the protective effect of Tamarix gallica against TAA-induced hepatic toxicity as tannins interfere with the metabolic activation of TAA into reactive intermediates and they might contribute to the chemopreventive effect of Tamarix gallica. In conclusion, the mechanism by which Tamarix gallica inhibit oxidative damage involves intracellular and extracellular pathways by scavenging free radicals, preventing nucleophilic sites of DNA, inhibiting the uptake of mutagens or their precursors. The chemopreventive potential of Tamarix gallica can be attributed to its polyphenolic ingredients. Further, it is also effective in inhibiting ODC activity and DNA synthesis that are associated with tumor promotion.
Acknowledgements
Dr. Sarwat Sultana is thankful to the Indian Council of Medical Research (ICMR), New Delhi, India for providing the necessary funds to conduct this study.
References
- Fitzhugh OG, Nelson AA. Liver tumors in rats fed thiourea or thioacetamide. Science 1948; 108: 626–628
- Dwivedi Y, Rastogi R, Sharma SK, Garg NK, Dhawan BN. Picroliv affords protection against Thioacetamide-induced hepatic damage in rats. Planta Med 1991; 57: 25–28
- Dogru-Abbasoglu S, Kanbagli O, Balkan J, Cevikbas U, Aykac-Toker G, Uysal.Hum M. The protective effect of taurine against thioacetamide hepatotoxicity of rats. Hum Exp Toxicol 2001; 20: 23–27
- Ramaiah SK, Apte U, Mehendale HM. Cytochrome P4502E1 induction increases Thioacetamide liver injury in diet restricted rats. Drug Metab Dispos 2001; 29: 1088–1095
- Ceruti PA. Oxy-radicals and cancer. The Lancet 1994; 344: 862–863
- Sultana S, Perwaiz S, Iqubal M, Athar M. Crude extracts of hepatoprotective plant Solanum nigrum and Chicorium intybus inhibit free radical-mediated DNA damage. J Ethnopharmacol 1995; 45: 189–192
- Marnette LJ. The involvement of peroxyl free radicals in tumor initiation and promotion. Anticarcinogenesis and radiation protection, PA Cerruti, OF Nygaard, MG Simic. Plenum Press, New York 1987; 71
- Sun Y. Free radicals, antioxidant enzymes and carcinogenesis. Free Rad Biol Med 1990; 8: 5839–5899
- Ferguson LR. Antimutagens as cancer chemopreventive agents in the diet. Mutat Res 1994; 307: 395–410
- Szarka CE, Grana G, Engstrom PF. Chemoprevention of cancer. Curr Probl Cancer 1994; 18: 6–79
- Khan N, Sultana S. Inhibition of potassium bromate-induced renal oxidative stress and hyperproliferative response by Nymphaea alba in Wistar rats. J Enz Inhib Med Chem 1999; 18: 691–698
- Khan N, Sharma S, Alam A, Sultana S. Tephrosia purpurea ameliorates N-diethylnitrosamine and potassium bromate- mediated renal oxidative stress and toxicity in Wistar rats. Pharmacol Toxicol 2001; 88: 294–299
- Sultana S, Khan N, Sharma S, Alam A. Modulation of biochemical parameters by Hemidesmus indicus in cumene hydroperoxide induced murine skin: Possible role in protection against free radical induced cutaneous oxidative stress and tumor promotion. J Ethno Pharmacol 2003; 85(1)33–41
- Kirthikar KR, Basu BD. Indian medicinal plants. Allahabad 1996b; 376–471
- Chauchan BL, Kulkarni RD. Effect of Liv 52, an herbal preparation, on absorption and metabolism of ethanol in humans. Eur J Clin Pharmacology 1991; 40: 189–191
- O'Brien TG, Simsiman RC, Boutwell RK. Induction of the polyamine-biosynthetic enzymes in mouse epidermis by tumor promoting agents. Cancer Res 1975; 35: 1662–1670
- Athar M, Raza H, Bickers D, Mukhtar H. Inhibition of benzoyl peroxide-mediated tumor promotion in 7,12-dimethylbenz(a)anthracene-initiated skin of sencar mice by antioxidants, nordihydroguaretic acid and diallyl sulphide. J Invest Dermatol 1990; 94: 162–165
- Lowry OH, Rosenbrough NJ, Farr AL, Randall RJ. Protein measurement with the Folin phenol reagent. J Biol Chem 1951; 193: 265–275
- Smart RC, Huang MT, Conney AH. Sn1, 2-diacylglycerols mimic the effects of TPA in vivo by inducing biochemical changes associated with tumor promotion in mouse epidermis. Carcinogenesis 1986; 7: 1865–1870
- Athar M, Iqbal M. Ferric nitrilotriacetate promotes N-diethylnitrosamine-induced renal tumorigenesis in the rats: implications for the involvement of oxidative stress. Carcinogenesis 1998; 19: 1133–1139
- Giles KW, Myers A. An improved diphenylamine method for the estimation of deoxyribonucleic acid. Nature 1965; 206: 63
- Jollow DJ, Mitchell JR, Zampaglione N, Gillette JR. Bromobenzene induced liver necrosis: protective role of glutathione and evidence for 3,4-bromobenzene oxide as the hepatotoxic intermediate. Pharmacology 1974; 11: 151–169
- Mohandas J, Marshall JJ, Duggin GG, Horvath JS, Tiller D. Differential distribution of glutathione and glutathione related enzymes in rabbit kidney: Possible interactions in analgesic neuropathy. Cancer Res 1984; 44: 5086–5091
- Habig WH, Pabst MJ, Jokoby WB. Glutathione-S-transferase: The first enzymatic step in mercapturic acid formation. J Biol Chem 1974; 249: 7130–7139
- Athar M, Khan WA, Mukhtar M. Effects of dietary tannic acid on epidermal, lung and forestomach polycyclic aromatic hydrocarbon metabolism and tumorigenicity in sencar mice. Cancer Res 1989; 49: 5783–5788
- Athar M, Sharma SD, Iqbal M, Sultana S, Pandeya KB, Tripathi IP. Coordination of copperpolyamine complex with imidazoles potentiates it superoxide dismutase mimicking activity and abolishes its interaction with albumin. Biochem Mol Biol Int 1996; 39(4)813–821
- Wright JR, Colby HD, Miles PR. Cytosolic factors which affect microsomal lipid peroxidation in lung and liver. Arch Biochem Biophys 1981; 206: 296–304
- Pick A, Keisari Y. Superoxide anion and H2O2 production by chemically elicited peritoneal macrophages-induction by multiple non phagocytic stimulus. Cell Immunol 1981; 59: 301–308
- Stevens MJ, Obrosova I, Cao X, Van Huysen C, Greene DA. Effects of DL-alpha- Lipoic acid on peripheral nerve conduction, blood flow, energy metabolism, and oxidative stress in experimental diabetic neuropathy. Diabetes 2000; 49: 1006–1015
- Kornberg A. Lactic dehydrogenase of muscle. Methods in Enzymology, SP Colowick, NO Kaplan. Academic Press, New York 1955; Vol I: 441–443
- Reitman S, Frankel S. A colorimetric method for the determination of serum oxaloacetic and glutamic pyruvic transaminases. Am J Clin Pathol 1957; 28: 56–63
- Orlowski M, Meister A. γ-glut tamyl cyclotransferase distribution, isozymic forms and specificity. J Biol Chem 1973; 248: 2836–2844
- Block G, Patterson B, Subar A. Fruits, vegetables, and cancer prevention: a review of the epidemiological evidence. Nutr Cancer 1992; 18: 1–29
- Weisburger JH. Molecular mechanisms anticarcinogenesis and antimutagenesis. Mutat Res 2001, 1: 23–35
- Talalay P, Talalay P. The Importance of Using Scientific Principles in the Development of Medicinal Agents from Plants. Acad Med 2001; 76: 238–247
- Ferreya EG, Castro JA, Diazgomez HI, Acoste ND, De castro CR, Fenos OM. Prevention and treatment of carbon tetrachloride hepatoptoxicity by cysteine:Studies about its mechanism. Toxicol Appl Pharmacol 1974; 27: 558–568
- Schmidt E, Schmidt FW, Mohr J, Otto P, Vido I, Wrogeman K, Herfarth C. Liver Morphology and enzyme release. Further studies in the isolated perfused rat liver. Pathogenesis and Mechanism of liver cell Necrosis, Keppler. Medical and Technical publishing Co Ltd, Lancaster 1975; 147
- Ansari RA, Tripathi SC, Patnaik GK, Dhawan BN. Antihepatotoxic properties of picroliv, an active fraction from rhizomes of Picrorhiza kurroa. J Ethnopharmacol 1991; 34: 61–68
- Pegg AE, Shantz LM, Coleman CS. Ornithine decarboxylase as a target for chemoprevention. J Cell Biochem 1995; 22: 132–138
- Carr CJ. Food and drug interactions. Xenobiotics in foods and feeds ACS Symposium 234, JW Finly, DE Schwass. American Chemical Society, Washington, DC 1985; 221–228
- Khan WA, Wang ZY, Athar M, Bickers DR, Mukhtar H. Inhibition of the skin tumorigenicity of (+/ − )-7-beta, 8-alpha-dihydroksy-3-alpha,10-alpha-epoxy-7,8,9,10-trahydrobenzo[a]pyrene by tannic acid, green tea polyphenols and quercetin in Sencar mice. Cancer Lett 1988; 42: 7–15
- Varanka Z, Rojik I, Varanka J, Nemcsok J, Abraham M. Biochemical and morphological changes in carp (Cyprinus carpio L.) liver following exposure to copper sulphate and tannic acid. Comp Biochem Phys C 2001; 128: 467–477
- Tanaka T, Kojima T, Kawamori T, Yoshimi N, Mori H. Chemoprevention of diethylnitrosamine-induced hepatocarcinogenesis by a simple phenolic acid protocatechuic acid in rats. Cancer Lett 1993; 53: 2775–2779
- Das M, Mukhtar H, Bik DP, Bickers DR. Inhibition of epidermal xenobiotic metabolism in SENCAR mice by naturally occurring plant phenols. Cancer Res 1987; 47: 760–766
- Teel RW, Dixit R, Stoner GD. The effect of ellagic acid on uptake, persistence, metabolism and DNA-binding of benzo(a)pyrene in cultured explains of strain A/J mouse lung. Carcinogenesis 1985; 6: 391–395
- Newmark H. Plant phenolics as inhibitors of mutational and precarcinogenic events. Can J Physiol Pharmacol 1987; 65: 61–466
- Singh K, Khanna AK, Chander R. Hepatoprotective activity of ellagic acid against carbon tetrachloride induced hepatotoxicity in rats. Ind J Exp Biol 1999; 37: 1025–1026