Abstract
In the present study, the influence of subchronic effects of two plant growth regulators (PGRs) [Abcisic acid (ABA) and Gibberellic acid (GA3)] on antioxidant defense systems [reduced glutathione (GSH), glutathione reductase (GR), superoxide dismutase (SOD), glutathione-S-transferase (GST) and catalase (CAT)] and lipid peroxidation level (malondialdehyde = MDA) in various tissues of the rat were investigated during treatment as a drinking water model. 75 ppm of ABA and GA3 in drinking water were continuously administered orally to rats (Sprague-Dawley albino) ad libitum for 50 days. The PGRs treatments caused different effects on the antioxidant defense systems and MDA content of dosed rats compared to controls. The lipid peroxidation end product MDA significantly increased in the lungs, heart and kidney of rats treated with GA3 without significant change in the spleen. ABA caused also a significant increase in MDA content in the spleen, lungs, heart and kidney. The GSH levels were significantly depleted in the spleen, lungs and stomach of rats treated with ABA without any change in the tissues of rats treated with GA3 except the kidney where it increased. Antioxidant enzyme activities such as SOD significantly increased in the lungs and stomach and decreased in the spleen and heart tissues of rats treated with GA3. Meanwhile, SOD significantly decreased in the spleen, heart and kidney and increased in the lungs of rats treated with ABA. While CAT activity significantly decreased in the lungs of rats treated with GA3, a significant increase occurred in the heart of rats treated with both PGRs. On the other hand, the ancillary enzyme GR activity in the tissues were either significantly depleted or not changed with PGRs treatment. The drug metabolizing enzyme GST activity significantly decreased in the lungs of rats treated with ABA but increased in the stomach of rats treated with both PGRs.
As a conclusion, the rats resisted oxidative stress via the antioxidant mechanism. But the antioxidant mechanism could not prevent the increases in lipid peroxidation in rat's tissues. This data, along with changes, suggests that PGRs produced substantial systemic organ toxicity in the spleen, lungs, stomach, heart and kidney during a 50-day period of subchronic exposure.
Introduction
Many chemicals are currently used in agriculture, and PGRs are among those widely employed. The amounts of these substances placed into the environment may soon exceed those of insecticides [Citation1]. Although PGRs are used for pest control and give rise to production of a wide variety of crops, little is known about the biochemical or physiological effects in mammals. Increased oxidative stress is obtained from a polluted area containing high concentrations of polyaromatic hydrocarbons, polychlorinated biphenyls, plant growth regulators (PGRs) and pesticides; however, little is known of how different PGRs classes contribute to the oxidative stress induced by these xenobiotics.
ABA plays important roles in many cellular processes including seed development, dormancy, germination, vegetative growth, and environmental stress responses. These diverse functions of ABA involve complex regulatory mechanisms that control its production, degradation, signal perception, and transduction. Because of the key role of ABA in plant stress responses, understanding these regulatory mechanisms will help devise rational strategies for breeding or genetically engineering crop plants with increased tolerance to adverse environmental conditions [Citation2].
GA3 plays, also, important roles in many cellular processes including promotion of stem elongation, overcomes dormancy in seed and buds, and is involved in parthenocarpic fruit development, flowering, mobilization of food reserves in grass seed germination, juvenility and sex expression [Citation3]
Antioxidant defenses, present in all aerobic organisms, include antioxidant enzymes and free-radical scavengers whose function is to remove reactive oxygen species (ROS), thus protecting the functions of organisms from oxidative stress [Citation4]. The sensitivity of the cell to oxidants is attenuated by antioxidant defense systems such as GSH, GST, CAT, SOD, GPx, GR and glucose-6-phosphate dehydrogenase (G6PD). The antioxidant defense system maintains a relatively low level of the reactive and harmful .OH. Oxidative stress occurs as a result of the effect of xenobiotics causing disturbances in the antioxidant enzyme systems [Citation5]. Among these, GPx, through reduction of both hydrogen peroxide and organic hydroperoxides, provides an efficient protection against oxidative damage and free radicals in the presence of reduced glutathione (GSH). Previously oxidized GSH is regenerated by GR. SOD catalyses dismutation of superoxide anion radicals, whereas CAT eliminates hydrogen peroxide. Antioxidant enzymes play a crucial role in maintaining cell homeostasis. Another group of enzymes, GST, act as a catalyst of a wide variety of conjugation reactions of glutathione with xenobiotic compounds containing an electrophilic center. Additionally, there are glutathione-independent enzymes that act as part of the cellular defense system against toxicity originated by active oxygen forms [Citation6]. Oxidative stress may produce DNA damage, enzymatic inactivation, and peroxidation of cell constituents, especially lipid peroxidation when antioxidant defenses are impaired or overcome [Citation7]. Oxidative stress is defined as a loss of the normal balance between reactive oxygen species (ROS) production and the antioxidant system [Citation5]. Indeed, in addition to an excess generation of ROS, xenobiotics have a decreased or increased antioxidant capacity, which causes oxidative damage to cells.
In the literature, it is reported that fecundity, longevity and egg viability have been changed in different insects by PGRs treatment Citation8-12. Frukawa et al.'s [Citation13] findings indicate that IAA (indole acetic acid) might induce neuronal apoptosis in the S phase and lead to microencephaly. Hsiao et al.'s [Citation14] results suggest that kinetin has effective free radical-scavenging activity in vitro and antithrombotic activity in vivo. Also, de Melo et al. [Citation15] found that incubation for 24 hours in the presence of IAA (1 mM) showed an increase in the activities of SOD, CAT and glutathione peroxidase. The addition of exogenous antioxidant enzymes (SOD and CAT) prevented the loss of cell membrane integrity induced by IAA. John et al. [Citation16] observed that IAA possessed teratogenic effects in mice and rats. Ozmen et al. [Citation17] observed that abscisic acid and gibberellic acid affected sexual differentiation and some physiological parameters of laboratory mice. Also, it is reported that PGRs cause an increase in the number of splenic plaque forming cells and circulating white blood cells, hematocrit values, and thymus weight in young deer mice [Citation18]. El-Mofty and Sakr [Citation19] found that GA3 induced liver neoplasm in Egyptian toads and suggested that the tumors could be diagnosed as hepatocellular carcinomas. GA3 also induces microabsceses and hydropic degeneration in the liver and mononuclear inflammatory infiltration in the kidneys of laboratory mice, but not tumors. On the other hand, some PGRs have been shown to affect the antioxidant defense systems and MDA content Citation20-21. The effects of IAA and Kn (kinetin) were also investigated on human serum enzymes in vitro. IAA was found to inhibit aspartate aminotransferase (AST) and activate amylase, creatine phosphokinase (CPK) and lactate dehydrogenase (LDH). Kn inhibited muscle creatine kinase (CK-MB), while it activated AST and alanine aminotransferase (ALT) [Citation22]. Also, we have found that while the levels of LDH and CPK were increased significantly by IBA (indole butyric acid) these levels and AST were increased significantly by IAA, and all three levels were increased significantly by kinetin [Citation23]. In addition, PGRs may induce oxidative stress, leading to generation of free radicals and cause lipid peroxidation as one of the molecular mechanisms involved in PGRs-induced toxicity [Citation24,Citation25]. According to The U.S. Environmental Protection Agency (EPA), toxic xenobiotic chemicals are irritating to the eyes, skin and mucous membrane and since they easily enter into the organism, e.g. orally or by inhalation, can injure liver, kidney, muscle and brain tissues.
Despite the reasons above, there is still great contradiction between results. Therefore, in order to achieve a more rational design of PGRs, it is necessary to clarify the mechanism of toxicity for PGRs and develop an understanding of structure-toxicity relationships. Here, with this aim, the PGRs treatment was done orally because the effect of chemicals represents a well characterized in vivo toxicity model system. The tissues were chosen due to their important role during detoxification in degradation and bioactivation of PGRs.
Materials and methods
Materials
PGRs, thiobarbituric acid (TBA), butylated hydroxytoluene (BHT), trichloroacetic acid (TCA), ethlenediaminetetraacetic acid (EDTA), reduced glutathione (GSH), metaphosphoric acid, 5,5′dithiobis-(2-nitrobenzoic acid) (DTNB), trihydroxymethyl aminomethane (Tris), 1-chloro-2,4-dinitrobenzene (CDNB), oxidized glutathione (GSSG), β-nicotinamide adenine dinucleotide phosphate (reduced) (NADPH), potassium chloride, hydrogen peroxide and sodium chloride of technical grade used in this study were supplied by Sigma Chemical Co. (St. Louis, MO, USA). Kits for antioxidant enzymes analysis were supplied by Randox Laboratories Ltd.
Animals
Rats (Sprague-Dawley albino) (4 months, 250–300 g) were provided by the animal house of the Medical School of Yüzüncü Yıl University, and were housed in 3 groups, each group containing 6 rats, at 20 ± 2°C in a daily light/dark cycle. All animals were fed a group wheat-soybean-meal-based diet and water ad libitum in stainless cages, and received humane care according to the criteria outlined in the ‘Guide for the Care and Use of Laboratory Animals’ prepared by the National Academy of Science and published by the National Institutes of Health [Citation26]. The ethical regulations have been followed in accordance with national and institutional guidelines for the protection of animal welfare during experiments.
Treatment of rats
This investigation was performed on male rats. The animals were housed for a minimum of five days to ’acclimatize’ before being dosed repeatedly with a substance over a period of 28–90 days. This was usually done orally (force-feeding with a syringe or tube) but may also have been administered dermally or inhaled. On the basis that it is accepted 7–28 days for subacute and 28–90 days for subchronic treatment the rats were exposed to 75 ppm ABA and GA3 in drinking water ad libitum for 50 days (subchronic). The PGRs (75 mg) were dissolved in 1 mL of 1 N NaOH which was then diluted with tap water to 1000 mL to obtain a 75 ppm dose. For the control rats, only 1 mL of 1 N NaOH was added to 1000 mL of tap water. Since all rats have the same physiological characters, daily water consumption of all groups of rats was approximately 30 ± 3 mL during the tests so that the amount of PGRs intake for each rat was about 2.2 ± 0.3 mg per day.
At the end of the treatment, the rats were anesthetized with diethyl ether, and after tissue samples were obtained, they were sacrificed. The tissues were dissected washed with physiological saline (0.9% NaCl), and kept at − 78°C until analysis. The tissues were homogenized for 5 min in 50 mM ice-cold KH2PO4 solution (1:5 w/v) using a glass-porcelain homogenizer (20 KHz frequency ultrasonic, Jencons Scientific Co.) for 5 min and then centrifuged at 7000 × g for 15 min. All processes were carried out at 4°C. Supernatants were used to determine antioxidant defense systems and MDA.
Biochemical analysis
The tissues MDA concentration was determined using the method described by Jain et al. [Citation27] based on TBA reactivity. Briefly, 0.2 mL supernatant obtained from tissues, 0.8 mL phosphate buffer (pH 7.4), 0.025 mL BHT and 0.5 mL 30% TCA were mixed. After 2 h incubation at − 20°C, the mixture was centrifuged (400 × g) for 15 min. After this, 1 mL supernatant was taken and mixed with 0.075 mL of 0.1 M EDTA and 0.25 mL of 1% TBA in tubes with Teflon-lined screw caps, and the mixture incubated at 90°C for 15 min and cooled to room temperature. The optical density was measured at 532 nm for the tissues' MDA concentration (Novaspec II Pharmacia-Biotech, Biochrom Ltd., UK).
The tissues' GSH concentration was measured using the method described by Beutler et al. [Citation28]. Briefly, 0.2 mL fresh supernatant was added to 1.8 mL distilled water. Three ml of the precipitating solution (1.67 g metaphosphoric acid, 0.2 g EDTA and 30 g NaCl in 100 mL distilled water) was mixed with the supernatant and the mixture allowed to stand for approximately 5 min and then filtered (Whatman N 42). Filtrate (2 mL) was taken, mixed with 8 mL of the phosphate solution (0.3 M disodium phosphate) and 1 mL DTNB was added. A blank was prepared with 8 mL of phosphate solution, 2 mL diluted precipitating solution (three parts to two parts distilled water), and 1 mL DTNB reagent. A standard solution of glutathione was prepared (40 mg /100 mL). The optical density was measured at 412 nm.
CAT (EC 1.11.1.6) activity was determined using the method described by Beutler [Citation29]. Briefly, 1 M Tris-HCl, 5 mM EDTA (pH = 8), 10 mM H2O2 and H2O were mixed and the rate of H2O2 consumption at 240 nm and 37oC was used for quantitative determination of CAT activity (20 μL of 95% ethanol was added in 1 mL of hemolysate to break down complex of catalase and H2O2). GST (EC 2.5.1.18) was assayed at 25oC spectrophotometrically by following the conjugation of glutathione with 1-chloro-2, 4-dinitrobenzene (CDNB) at 340 nm as described by Mannervik and Guthenberg [Citation30]. GR (EC 1.6.4.2) activity was assayed at 37°C and 340 nm by following the oxidation of NADPH by GSSG [Citation29]. SOD (EC 1.15.1.1) activity was measured at 505 nm and 37°C and calculated using percentage inhibition of formazon formation [Citation31].
Analysis of data
The data were expressed as mean ± standard deviation (SD). For statistical analysis the SPSS/PC+ package (SPSS/PC+, Chicago, IL, USA) was used. For all parameters, means and SD were calculated according to standard methods. The Mann-Whitney U-test was employed to determine differences between means of the treatments and the control rats. The significance level was accepted at p ≤ 0.05 for all tests.
Results and discussion
In the present study, ABA and GA3 were selected for study because information on their negative effects on higher animals are very limited for in vivo, oral exposures. Also, ABA and GA3 are found in a wide variety of biologically active compounds. The data collected in this study were from a single time-point of the experiment. We found that treatment with ABA and GA3 affected the MDA content and antioxidant defense systems in various tissues of rats.
So far, no in vivo study examining the effect of ABA and GA3 in drinking water has been made on the MDA content and antioxidant defense of these tissues. So, we could not compare our results with previous results. However, Tuluce and Celik [Citation24] have determined the effects of ABA and GA3 on different antioxidant defense systems and MDA content in different tissues of rats. Also, Celik and Tuluce [Citation20] have determined the effects of IAA and Kn on different antioxidant defense systems in these tissues of rats. On the other hand, de Melo et al. [Citation15] found that the incubation of rat neutrophils and lymphocytes for 24 h in the presence of IAA (1 mM) showed an increase in CAT activity. These studies are partly in agreement with our results although the experimentals conditions are different. Ciftci et al. [Citation32] also found that both in vivo and in vitro treatment with spermidine and kinetin did not affect the enzymatic activity of CAT and G6PD whereas putrescine decreased and abscisic acid increased the activity of the enzymes. Due to the different treatments, materials and the settings, the results are contradictory to our findings. In addition, because of the high variability in analysing immune potential and antioxidant enzymes-chemicals interaction in vivo and in vitro, and inconsistent factors like treatment time and manner, the setting of studies, purity of chemicals and species tissue differences etc., it is difficult to compare the present data to previous studies regarding the toxicological effect.
The results of the present study have also demonstrated that ABA and GA3 could have affected the antioxidant defense system and MDA content in vertebrates. This is seen from our observation that upon PGRs treatment in vivo, the activity of the enzymes and GSH levels in the spleen, lungs, stomach, heart and kidney differ from those of controls. PGRs caused significant alterations in the level of enzymes in almost all the tissues (). ABA caused a significant decrease in the activity of GR in spleen and heart, and significant decrease in SOD activity in spleen, heart and kidney and an increase in spleen. Furthermore, ABA caused a significant decrease in GST activity in the lungs but an increase in the stomach. On the other hand, antioxidant enzyme activities such as CAT significantly increased in the heart on with ABA. GA3 also significantly influenced the activity of enzymes; GR activity decreased in spleen and heart, and decreased spleen, heart and kidney SOD and lungs CAT activity whereas it increased stomach SOD and GST and heart CAT activity. The reasons for such effect of PGRs are not understood at the present. However, PGRs may lead to inhibition of enzyme synthesis in tissues as a result of mRNA breakdown or cellular transcription mechanisms. Also, oxidative stress can affect the activities of protective enzymatic antioxidants in organisms exposed to PGRs. The enzymatic antioxidants such as SOD, GR, GST and CAT have been shown to be sensitive indicators of increased oxidative stress in Mugil sp obtained from a polluted area containing high concentrations of polyaromatic hydrocarbons, polychlorinated biphenyls, and pesticides [Citation33]. The increased activities of SOD, CAT, GPx, GR, and GST are known to serve as protective responses to eliminate reactive free radicals [Citation34]. The changes in the levels of enzymes presented in this study might indicate that the exposure of rats to ABA and GA3 could affect no enzymatic antioxidant defense enzymes in vertebrates too. Results also showed that the GSH levels () were significantly depleted in the spleen, lungs and stomach of rats treated with ABA without any change in the tissues of rats treated with GA3 except the kidney in which it increased. Although the reasons for such effect of PGRs are not understood at the present, the increased ROS may result in an increase in GSH levels. It is conceivable that ABA and GA3 might be interacting primarily with the tissues, resulting in fluctuated enzyme activities through increased superoxide radicals as result of stress conditions in the rats. However, it is not a general rule that increases in pollutant concentrations induce antioxidant activity. Doyotte et al. [Citation35] pointed out that a decreased enzyme activity's response may accompany an initial exposure to pollutants, which can be followed by an induction of antioxidant systems. Thus, the existence of an inducible antioxidant system may reflect an adaptation of the organism. Another possibility is that PGRs may lead to the inhibition or stimulation of enzymes synthesis in tissues as result of mRNA breakdown or cellular transcription mechanisms. Nevertheless, the physiological roles of these antioxidant enzymes in the cell are poorly understood because of complex interactions and interrelationships among individual components. The findings of this study suggest that further experiments should be performed to elicit what is responsible for the decrease or increase in level of the antioxidant defense systems. In addition, the findings of this study suggest that the fluctuated levels in the constituents of the antioxidant defense systems and MDA content in the tissues of rats exposed to PGRs may be dependent on the differences between interstitial concentrations. Namely, the systems might have been exposed to different xenobiotic concentration due to blood volume differences in the tissues.
Figure 1 Effects of subchronic treatment of ABA and GA on GSH levels (mg/g tissue) in different tissues of rats. Values are means ± S.D.
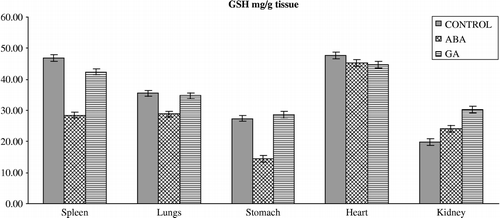
Figure 2 Effects of subchronic treatment of ABA and GA on MDA content (nmol/g tissue) in different tissues of rats. Values are means ± S.D.
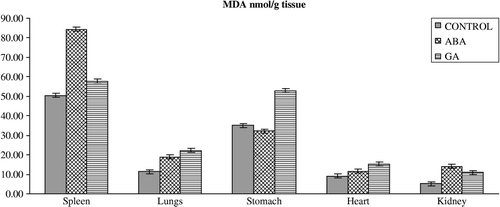
Figure 3 Effects of subchronic treatment of ABA and GA on GR activity (U/g tissue) in different tissues of rats. Values are means ± S.D.
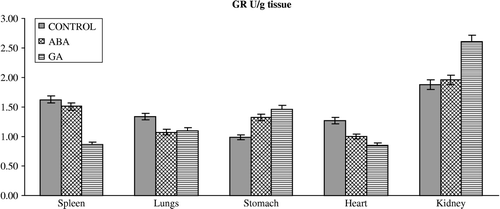
Figure 4 Effects of subchronic treatment of ABA and GA on GR activity (U/g tissue) in different tissues of rats. Values are means ± S.D.
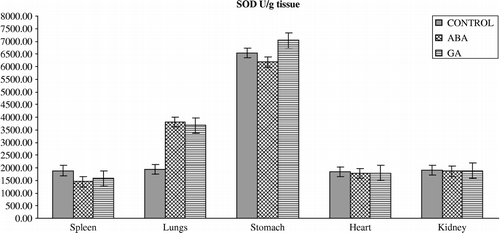
Figure 5 Effects of subchronic treatment of ABA and GA on GST activity (U/g tissue) in different tissues of rats. Values are means ± S.D.
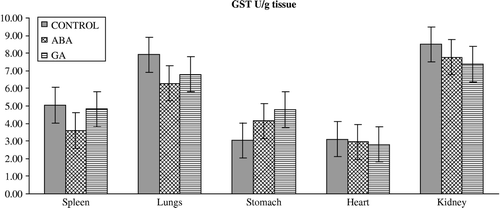
Figure 6 Effects of subchronic treatment of ABA and GA on CAT activity (U/g tissue) in different tissues of rats. Values are means ± S.D.
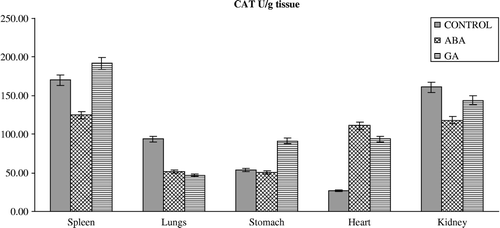
In addition to the fluctuated level of the antioxidant defense systems, oxidative stress can affect the MDA content in organisms exposed to PGRs (). The lipid peroxidation end product, MDA, significantly increased in the lungs, heart and kidney of rats treated with GA3 without significant change in the spleen. ABA also caused a significant increase in MDA content in the spleen, lungs, heart and kidney. The reasons for such effects of PGRs are also not understood at the present, but the increased MDA content may result from an increase of hydroxyl radicals (.OH). Since it is known that .OH can initiate lipid peroxidation in tissues [Citation6]. However, it is conceivable that ABA and GA3 might be interacting primarily with the lungs, heart and kidney, resulting in lipid peroxidation processes by way of an increase in superoxide radicals as a result of the stressed condition in the rats, leading to an increase in lipid peroxidation. MDA is a major oxidation product of peroxidized polyunsaturated fatty acids and an increased MDA content is an important indicator of lipid peroxidation [Citation36].
In conclusion, these results suggest that the constituents of the antioxidant defense systems and lipid peroxidation content of tissues may offer a means for monitoring the toxicity of compounds such as PGRs and be used in future investigations if more studies confirm our findings. Such a test will be of value in pollution studies, and also be of interest to understand the molecular basis of PGRs toxicity.
Abbreviations | ||
ADA: | = | adenosine deaminase |
ALT: | = | alanin aminotransferase |
AST: | = | aspartate aminotransferase |
BHT: | = | butylated hydroxytoluene |
CAT: | = | catalase |
CDNB: | = | 5,5′dithiobis-(2-nitrobenzoic acid) |
CPK: | = | creatine phosphokinase |
EDTA: | = | ethlenediaminetetraacetic acid |
GPx: | = | glutathione peroxidases |
GSH: | = | reduced glutathione |
GR: | = | glutathione reductase |
G6PD: | = | glucose-6-phosphate dehydrogenase |
IAA: | = | indole acetic acid |
IBA: | = | indole butyric acid |
Kn: | = | kinetin |
LDH: | = | lactate dehydrogenase |
NADPH: | = | β-Nicotinamide adenine dinucleotide phosphate (reduced) |
MDA: | = | Malondialdehyde |
MPO: | = | myeloperoxidase |
GSSG: | = | oxidized glutathione |
PGRs: | = | plant growth regulators |
ppm: | = | parts per million |
SOD: | = | superoxide dismutase |
References
- Mickel LG. “Plant growth regulators” controlling biological behavior with chemicals. Chem Eng News 1978; 56: 18
- Xiong L, Zhu JK. Regulation of abscisic acid biosynthesis. Plant Physiol 2003; 133: 29–36
- Salisbury FB, Ross CW. Plant physiology. Wadsworth, Belmont, CA 1992; 357–407, 531–548
- Regoli F, Principato G. Glutathione, glutathione dependent and antioxidant enzymes in mussel, mytilus galloprovincialis, exposed to metals under field and laboratory conditions: Implications for the use of biochemical biomarkers. Aquat Toxicol 1995; 31: 143–164
- Oruc-Ozcan E, Uner N. Marker enzyme assesment in the liver of Cyprinus carpio (L.) exposed to 2,4-D and azinphosmethyl. J Bıochem Molec Toxıcol 2002; 16: 183–188
- Halliwell B, Gutteridge JMC. Free radicals in biology and medicine2nd reprint. Clarendon Press, Oxford 1989; 543
- Himmelfarb J, Hakim RM. Oxidative stress in uremia. Curr Opin Nephrol Hypertens 2003; 12: 593–598
- Guarra AA. Effect of biological active substance in the diet on development and reproduction of Heliothis spp. J Econ Entomol 1970; 63: 1518–1521
- Visscher NS. Regulation of grasshopper fecundity, longevity and egg viability by plant growth hormones. Experimentia 1980; 36: 130–131
- Visscher NS. Special report dietary plant growth hormones affect insect growth and reproduction. Bull Plant Growth Reg Soc Am 1983; 11: 4–6
- De Man W, De Loof A, Briers T, Huybrechts R. Effect of abscisic acid on vitellogenesis in Sarcophaga bullata. Entomol Exp Appl 1991; 29: 259–267
- Alanso C. The effects of gibberellic acid upon developmental processes in Drosophila hydlei. Entomol Exp Appl 1971; 14: 73–82
- Furukawa S, Abe M, Usuda K, Ogawa I. Indole-3-acetic acid induces microencephaly in rat fetuses. Toxicol Pathol 2004; 32(6)659–667
- Hsiao G, Shen MY, Lin KH, Chou CY, Tzu NH, Lin CH, Chou DS, Chen TF, Sheu JR. Inhibitory activity of kinetin on free radical formation of activated platelets in vitro and on thrombus formation in vivo. Eur J Pharmacol 2004; 465(3)281–287
- de Melo MP, de Lima TM, Pithon-Curi TC, Curi R. The mechanism of indole acetic acid cytotoxicity. Toxicol Lett 2004; 14: 103–111
- John JA, Blogg CD, Murray FJ, Schwetz BA, Gehring PJ. Teratogenic effects of the plant hormone indole-3-acetic acid in mice and rats. Teratology 1979; 19(3)321–324
- Ozmen M, Topcuoglu SF, Bozcuk S, Bozcuk NA. Effects of abscisic acid and gibberellic acid on sexual differentiation and some physiological parameters of laboratory mice. Tr J Biol 1995; 19: 357–364
- Olson LJ, Hinsdill RD. Influence of feeding chlorocholine chloride and glyposine on selected immune parameters in deer mice peromiseus moniculatus. Toxicology 1984; 30: 103–114
- El-Mofty MM, Sakr SA. Induction of neoplasms in the Egyptian Toad by gibberellin A3. Oncology 1988; 45: 61–64
- Celik I, Tuluce Y. Effects of Indoleacetic acid and kinetin on lipid peroxidation and antioxidant defense in various tissues of rats. Pest Biochem Physiol 2006; 84: 49–54
- Celik I, Tuluce Y, Türker M. Antioxidant and immune potential marker enzymes assessment in the various tissues of rats exposed to indoleacetic acid and kinetin: A drinking water study. Pest Biochem Physiol, (in press)
- Celik I, Kara M. The effects of some plant growth regulators on activity of eight serum enzymes in-vitro. J Environ Sci Health 1997; 32: 1755–1761
- Celik I, Ozbek H, Tuluce Y. Effects of subchronic treatment of some plant growth regulators on serum enzyme levels in rats. Tr J Biol 2002; 26: 73–76
- Tuluce Y, Celik I. Influence of subacute and subchronic treatment of abcisic acid and gibberellic acid on serum marker enzymes and erythrocyte and tissue antioxidant defense systems and lipid peroxidation in rats. Pest Biochem and Physiol, (in press)
- Candeias LP, Folkes LK, Porssa M, Parrick J, Wardman P. Enhancement of lipid peroxidation by indole-3-acetic acide and derivates: Substituent effects. Free Rad Res 1995; 23: 403–418
- World Medical Association Declaration of Helsinki. 52nd WMA General Assembly, Edinburgh, Scotland, 2000.
- Jain SK, McVie R, Duett J, Herbst JJ. Erythrocyte membrane lipid peroxidation and glycolylated hemoglobin in diabetes. Diabetes 1989; 38: 1539–1543
- Beutler E, Dubon OB, Kelly M. Improved method for the determination of blood glutathione. J Lab Clin Med 1963; 61: 882–888
- Beutler E. Red Cell Metabolism: A Manual of Biochemical Methods, Third Edition. New York: Grune and Startton; 1984. p 105–106.
- Mannervik B, Guthenberg C. Glutathione S-transferase (Human placenta). Method Enzymol 1981; 77: 231–235
- McCord JM, Fridovich I. Superoxide dismutase: Anenzymatic function for erythrocuprein (hemocuprein). J Biol Chem 1969; 244: 6049–6053
- Ciftci M, Demir Y, Ozmen I, Atici O. In vivo and in vitro effects of some plant hormones on rat erythrocyte carbonic anhydrase and glucose-6-phosphate dehydrogenase activities. J Enz Inhib Med Chem 2003; 18: 71–76
- Rodriguez-Ariza A, Peinado J, Pueyo C, Lopez-Barea J. Bichemical indicators of oxidative stress in fish from polluted littoral areas. Can J Fish Aquat Sci 1993; 50: 2568–2573
- Cheung CC, Zheng GJ, Li AMY, Richardson BJ, Lam PKS. Relationship between tissue concentrations of polycyclic aromatic hydrocarbons and antioxidative responses of marine mussels. Perna viridis Aquat Toxicol 2001; 52: 189–203
- Doyotte A, Cossu C, Jacquin MC, Babut M, Vasseur P. Antioxidant enzymes, glutathione and lipid peroxidation as relevant biomarkers of experimental or field exposure in the gills and the digestive gland of the freshwater bivalve Unio tumidus. Aquat Toxicol 1997; 39: 93–110
- Freeman BA, Crapo JD. Hyperoxia increases oxygen radical production in rat lung and lung mitochondria. J Biol Chem 1981; 256: 10986–10992