Abstract
In this study, di(2,6-dimethylphenol) (Di-DMP), di(2,6-diisopropylphenol) (Di-DIP, dipropofol) and di(2,6-di-t-butylphenol) (Di-DTP) were synthesized by the reaction of monomeric phenol derivatives with catalytic CuCl(OH). TMEDA and Na2S2O4. Their antioxidant capacity and radical scavenging activity were examined using different in vitro methodologies such as 1,1-diphenyl-2-picryl-hydrazyl (DPPH·) free radical scavenging, 2,2′-azino-bis(3-ethylbenzthiazoline-6-sulfonic acid) (ABTS) radical scavenging activity, total antioxidant activity by ferric thiocyanate, total reducing power by potassium ferricyanide reduction method, superoxide anion radical scavenging, hydrogen peroxide scavenging and ferrous ions chelating activities.
Introduction
Although oxygen is a prerequisite to life, at concentrations beyond the physiological limits it may be hazardous to cells [Citation1]. Especially, oxidation of lipids, which is the main cause of quality deterioration in many food and pharmaceutical systems, may lead to off-flavours and formation of toxic compounds and oxidized lipids may lower the quality and nutritional or pharmaceutical value of foods. Furthermore, lipid oxidation is also associated with aging, membrane damage, heart disease and cancer [Citation2,Citation3]. Alternatively, the inhibition of membrane lipid peroxidation has been shown to have a protective effect in the initiation and promotion of certain cancers and in the side effects of several cytostatic agents [Citation4].
Free radicals are chemically active atoms or molecular fragments that have a charge due to an excess or deficient number of electrons. Free radicals containing oxygen are known as reactive oxygen species (ROS) and are the most biologically significant free radicals. Oxidative stress is initiated by ROS, such as superoxide anion radicals (O2·–), hydroxyl radicals (OH·) and non free-radical species such as H2O2 and singlet oxygen (1O2). These radicals are formed by a one electron reduction process of molecular oxygen (O2). These various forms of activated oxygen are exacerbating factors in cellular injury and aging process Citation5-7 and are linked to oxidation of proteins, DNA, and lipids [Citation1].
At normal physiological concentrations ROS are required for cellular activities. But, at higher concentrations, they can be toxic leading to oxidative stress. The excessive production of ROS from endogenous and exogenous sources disrupts many functions in living cells [Citation8]. The main cellular sources of ROS in the lung include not only neutrophils, eosinophils and alveolar macrophages [Citation9], but also alveolar epithelial cells, bronchial epithelial cells and endothelial cells [Citation10,Citation11]. The generation of ROS in the lungs is enhanced after exposure to numerous exogenous chemical and physical agents, which include mineral dusts, ozone, nitrogen oxides, ultraviolet and ionizing radiation [Citation12,Citation13] and tobacco smoke [Citation14]. ROS can easily initiate the peroxidation of membrane lipids, depletion of nicotinamide nucleotides, rises in intracellular calcium ions, cytoskeleton disruption and DNA damage [Citation1,Citation15]. However, they are removed by antioxidant defence mechanisms. There is a balance between the generation of ROS and inactivation of ROS by the antioxidant system in organisms. Under pathological conditions, ROS are overproduced and result in oxidative stress. ROS are formed when endogenous antioxidant defences are inadequate. The imbalance between ROS and antioxidant defence mechanisms leads to oxidative modification in cellular membrane or intracellular molecules Citation16-18.
Antioxidants may be defined as compounds that inhibit or delay the oxidation of other molecules by inhibiting the initiation or propagation of oxidizing chain reactions. Antioxidants, in general, are important not only for food preservation but also for the defence of the body against oxidative stress Citation19-20. They can also protect the human body from free radicals and ROS effects and retard the progress of many chronic diseases as well as lipid peroxidation Citation21-25 which is also associated with a number of diseases such as aging, membrane damage, heart disease and cancer [Citation2].
Although synthetic antioxidants such as butylated hydroxytoluene (BHT), butylated hydroxyanisole (BHA) and tert-butylhydroquinone (TBHQ) as well as propyl gallate (PG) have widely been used in retarding lipid peroxidation, their safety has recently been questioned due to toxicity e.g. liver damage and possible carcinogenicity [Citation18,Citation26,Citation27]. Thus, development of safer antioxidants from other materials that can replace these antioxidants has been of interest. We suggested that Di-DMP, Di-DIP and Di-DTP were safer than the above mentioned antioxidants. Especially propofol, the monomeric form of Di-DIP, which has a wide usage. DIP is a hypnotic intravenous agent with in vivo antioxidant and radical scavenging properties and commonly used as a sedative for critically ill patients [Citation28]. In another study, Di-DIP, dipropofol, showed a bactericidal effect against gram-positive bacteria, including Staphylococcus aureus, Enterococcus faecium and Escherichia coli. Ogata and co-workers showed that some of the dimeric phenol derivatives had a stronger in vivo antioxidant activity by thiobarbituric acid-reactive substances than related monomers [Citation29].
As far as our literature survey could ascertain, no information is available on Di-DMP, Di-DIP and Di-DTP for their in vitro total antioxidant activity, reducing power, DPPH· free radical scavenging, ABTS radical scavenging, superoxide anion radical scavenging, hydrogen peroxide scavenging and metal chelating activities. The objectives of this study were to investigate these properties of Di-DMP, Di-DIP and Di-DTP compared with the same concentration of commercial and standard antioxidant and radical scavengers such as BHA, BHT, α-tocopherol and trolox.
Materials and methods
Chemicals
DMP, DIP, DTP, ABTS, BHA, BHT, nitroblue tetrazolium (NBT), DPPH·, 3-(2-pyridyl)-5,6-bis (4-phenyl-sulfonic acid)-1,2,4-triazine (Ferrozine), linoleic acid, α-tocopherol, polyoxyethylenesorbitan monolaurate (Tween-20) and trichloroacetic acid (TCA) were obtained from Sigma-Aldrich GmbH, Sternheim, Germany. Ammonium thiocyanate was from Merck and all other chemicals used were of analytical grade and obtained from either Sigma-Aldrich or Merck.
Synthesis of dimeric phenols
In this study, Di-DMP, Di-DIP and Di-DTP were synthesized by methods described in the literature [Citation29,Citation30].
Synthesis of di(2,6-dimethylphenol) (Di-DMP)
DMP (5.0 g, 40.93 mmol) was dissolved in CH2Cl2 (100 mL) and mixed with CuCl(OH)·TMEDA (160 mg, 0.34 mmol) at room temperature at 24 h. The reaction product extracted with AcOEt and evaporated. The reactant was dissolved in ethanol, Na2S2O4 (10.0 g, 57.47 mmol) added and the mixture was heated for 2 h. The precipitate was collected and crystallized from hexane to give di(2,6-dimethylphenol) (3.62 g, 73%) as a white solid. 1H-NMR (400 MHz, CDCl3, δ, ppm): 7.16 (s, 4H, Haryl), 4.60 (s, 2H, OH), 2.30 (s, 12H, CH3). 13C-NMR (100 MHz, CDCl3, δ, ppm): 151.47, 133.55, 127.22, 123.36, 16.30. Anal. Calc. for C16H18O2: C, 79.31; H, 7.49. Found: C, 79.17; H, 7.67%.
Synthesis of di(2,6-diisopropylphenol) (Di-DIP, Dipropofol)
Propofol (4.0 g, 22.47 mmol) was dissolved in CH2Cl2 (40 mL) and stirred with CuCl(OH)·TMEDA (64 mg, 0.14 mmol) for 24 h at room temperature. The reaction product was extracted with AcOEt and the solvent was evaporated to afford a resultant residue which was dissolved in ethanol, and heated with Na2S2O4 (6.0 g, 34.48 mmol) for 20 min. The separated precipitate was collected and crystallized from hexane to give the dimeric propofol, dipropofol (2.94 g, 74%) as a white solid. 1H-NMR (400 MHz, CDCl3, δ, ppm): 7.33 (s, 4H, Haryl), 4.99 (m, 2H, OH), 3.35 (h, J = 6.8 Hz, 4H, CH), 1.46 (d, J = 6.8 Hz, 12H, CH3). 13C-NMR (100 MHz, CDCl3, δ, ppm): 149.46, 135.13, 134.19, 122.74, 27.77, 23.21. Anal. Calc. for C24H34O2: C, 81.31; H, 9.67. Found: C, 79.62; H, 9.70%.
Synthesis of di(2,6-di-t-butylphenol) (Di-DTP)
DTP (5.0 g, 24.27 mg) was dissolved in CH2Cl2 (100 mL) and stirred with CuCl(OH)·TMEDA (160 mg, 0.34 mmol) for 24 h at room temperature. The reaction product was extracted with AcOEt. After removal of solvent, the reactant was dissolved in ethanol, and heated with Na2S2O4 (6.0 g, 34.48 mmol) for 2 h. The precipitate was collected and crystallized from hexane to give di(2,6-di-t-butylphenol) (3.58 g, 72%) as a white solid. 1H-NMR (400 MHz, CDCl3, δ, ppm): 7.33 (s, 4H, Haryl), 5.22 (s, 2H, OH), 1.52 (s, 36H, CH3). 13C-NMR (100 MHz, CDCl3, δ, ppm): 153.06, 136.15, 134.18, 124.38, 34.70, 30.61. Anal. Calc. for C28H42O2: C, 81.90; H, 10.31. Found: C, 81.50; H, 10.27%.
Total antioxidant activity determination by ferric thiocyanate method
The antioxidant activity of Di-DMP, Di-DIP and Di-DTP and standards was determined according to the ferric thiocyanate method [Citation31] as described by Gülçin [Citation32]. For stock solutions, 10 mg of Di-DMP, Di-DIP and Di-DTP was dissolved in 10 mL ethanol. Then, the solution which contains the same concentration of stock Di-DMP, Di-DIP and Di-DTP solution or standard samples (from 15 μg/mL to 45 μg/mL) in sodium phosphate buffer (0.04 M, pH 7.0, 2.5 mL) was added to linoleic acid emulsion in sodium phosphate buffer (0.04 M, pH 7.0, 2.5 mL). 5 mL of the linoleic acid emulsion was prepared by mixing and homogenizing 15.5 μL of linoleic acid, 17.5 mg of Tween-20 as emulsifier, and 5 mL phosphate buffer (pH 7.0). Alternatively, 5 mL control was composed of 2.5 mL of linoleic acid emulsion and 2.5 mL, 0.04 M sodium phosphate buffer (pH 7.0). The mixed solution (5 mL) was incubated at 37°C in a polyethylene flask. The peroxide level was determined by reading the absorbance at 500 nm in a spectrophotometer (Shimadzu, UV-1208 UV-VIS Spectrophotometer, Japan, Cat No: 2006-67603-93, Serial No: A1012 3300010YS) after reaction with FeCl2 and thiocyanate at intervals during the incubation. During the linoleic acid oxidation, peroxides are formed and that leads to oxidation of Fe2+ to Fe3+. The latter ions form a complex with ammonium thiocyanate and this complex has a maximum absorbance at 500 nm. This step was repeated every 5 h until the control reached its maximum absorbance value. The percentage inhibition values were calculated at this point (30 h). High absorbance indicates high linoleic acid emulsion peroxidation. The solutions without Di-DMP, Di-DIP and Di-DTP were used as blank samples. Total antioxidant activity determination was performed in triplicate. The percentage inhibition of lipid peroxidation in the linoleic acid emulsion was calculated by the following equation:
in here AC is the absorbance of the control reaction which contains only linoleic acid emulsion and sodium phosphate buffer and AS is the absorbance in the presence of the test sample Di-DMP, Di-DIP and Di-DTP or standard compounds [Citation33].
Total reduction capability
The samples prepared for the ferric thiocyanate method above were used for this and the other assays. The reducing power of Di-DMP, Di-DIP and Di-DTP was determined by the method of Oyaizu [Citation34] with slight modification [Citation35]. Different concentrations of Di-DMP, Di-DIP and Di-DTP (15–45 μg/mL) in 1 mL of distilled water were mixed with phosphate buffer (2.5 mL, 0.2 M, pH 6.6) and potassium ferricyanide [K3Fe(CN)6] (2.5 mL, 1%). The mixture was incubated at 50°C for 20 min. Aliquots (2.5 mL) of trichloroacetic acid (10%) were added to the mixture. The supernatant (2.5 mL) was mixed with distilled water (2.5 mL) and FeCl3 (0.5 mL, 0.1%), and the absorbance was measured at 700 nm in a spectrophotometer. Increased absorbance of the reaction mixture indicates an increase in reduction capability.
Chelating activity on ferrous ions (Fe2+)
The chelating of ferrous ions by Di-DMP, Di-DIP and Di-DTP and standards was estimated by the method of Dinis et al. [Citation36]. The reaction was performed in an aqueous medium. Briefly, Di-DMP, Di-DIP and Di-DTP (15 μg/mL) in 0.4 mL was added to a solution of 2 mM FeCl2 (0.2 mL). The reaction was initiated by the addition of 5 mM ferrozine (0.4 mL) and the total volume was adjusted to 4 mL of ethanol. The mixture was then shaken vigorously, left at room temperature for ten min, and its absorbance measured spectrophotometrically at 562 nm. The percentage of inhibition of ferrozine-Fe2+ complex formation was calculated using the formula below:
where AC is the absorbance of control and AS is the absorbance in the presence of Di-DMP, Di-DIP and Di-DTP or standards. The control contains FeCl2 and ferrozine, the complex formation molecules [Citation37,Citation38].
Hydrogen peroxide scavenging activity
The hydrogen peroxide scavenging assay was carried out following the procedure of Ruch et al. [Citation39]. For this purpose, a solution of H2O2 (43 mM) was prepared in phosphate buffer (0.1 M, pH 7.4). Di-DMP, Di-DIP and Di-DTP at 15 μg/mL concentration in 3.4 mL phosphate buffer were added to 0.6 mL of H2O2 solution (0.6 mL, 43 mM). The absorbance of the reaction mixture was recorded at 230 nm. A blank solution contained the phosphate buffer without H2O2. The concentration of hydrogen peroxide in the assay medium was determined using a standard curve (r2:0.9895):
The percentage of H2O2 scavenging by Di-DMP, Di-DIP and Di-DTP and standard compounds was calculated using the following equation:
where AC is the absorbance of the control and AS is the absorbance in the presence of the test sample Di-DMP, Di-DIP, Di-DTP or standards [Citation40].
Radical scavenging activity
The total free radical scavenging capacity of the tested compounds was determined and compared to that of BHA, BHT, α-tocopherol and trolox using the DPPH, ABTS and superoxide anion radical scavenging methods.
DPPH free radical scavenging activity
The methodology of Blois [Citation41] previously described by Gülçin [Citation42] was used with slight modifications in order to assess the DPPH· free radical scavenging capacity of Di-DMP, Di-DIP and Di-DTP, wherein the bleaching rate of a stable free radical, DPPH· is monitored at a characteristic wavelength in the presence of the sample. In its radical form, DPPH· absorbs at 517 nm, but upon reduction by an antioxidant or a radical species its absorption decreases. Briefly, 0.1 mM solution of DPPH· was prepared in ethanol and 0.5 mL of this solution was added to 1.5 mL of Di-DMP, Di-DIP and Di-DTP solution in ethanol at different concentrations (15–45 μg/mL). These solutions were vortexed thoroughly and incubated in the dark. Half an hour later, the absorbance was measured at 517 nm against blank samples. Lower absorbance of the reaction mixture indicates higher DPPH· free radical scavenging activity. A standard curve was prepared using different concentrations of DPPH·. The DPPH· scavenging capacity was expressed as mM in the reaction medium and calculated from the calibration curve determined by linear regression (r2:0.9845):
The capability to scavenge the DPPH· radical was calculated using the following equation:
where AC is the absorbance of the control which contains 0.5 mL DPPH· solution and AS is the absorbance in the presence of Di-DMP, Di-DIP and Di-DTP [Citation43].
ABTS radical cation decolorization assay
The spectrophotometric analysis of ABTS√+ radical scavenging activity was determined according to the method of Re et al. [Citation44] described previously by Gülçin [Citation45]. This method is based on the ability of antioxidants to quench the long-lived ABTS radical cation, a blue/green chromophore with characteristic absorption at 734 nm, in comparison to that of BHA, BHT, α-tocopherol and trolox (a water-soluble α-tocopherol analogue). The ABTS√+ was produced by reacting 2 mM ABTS in H2O with 2.45 mM potassium persulfate (K2S2O8), in the dark at room temperature for 4 h. Before usage, the ABTS√+ solution was diluted to get an absorbance of 0.750 ± 0.025 at 734 nm with phosphate buffer (0.1 M, pH 7.4) before use. Then, 1 ml of ABTS√+ solution was added to 3 mL of Di-DMP, Di-DIP and Di-DTP solution in ethanol at different concentrations (15–45 μg/mL). After 30 min, the percentage inhibition at 734 nm was calculated for each concentration relative to a blank absorbance. Solvent blanks were run in each assay. The extent of decolorization was calculated as percentage reduction of absorbance. For preparation of a standard curve, different concentrations of ABTS√+ were used. The ABTS√+ concentration (mM) in the reaction medium was calculated from the following calibration curve, determined by linear regression (r2:0.9841):
The scavenging capability of the ABTS√+ radical was calculated using the following equation:
where in AC is the initial concentration of ABTS√+ and AS is absorbance of the remaining concentration of ABTS√+ in the presence of Di-DMP, Di-DIP and Di-DTP [Citation18,Citation32].
Superoxide anion radical scavenging activity
Superoxide radicals were generated by the method of Beauchamp and Fridovich [Citation45] described by Zhishen et al. [Citation46] with slight modification. Superoxide radicals were generated in riboflavin, methionine, illuminate and assayed by the reduction of nitro blue tetrazolium (NBT) to form blue formazan. All solutions were prepared in 0.05 M phosphate buffer (pH 7.8). The photo-induced reactions were performed using fluorescent lamps (20 W). The total volume of the reactant mixture was 5 mL and the concentrations of riboflavin, methionine and NBT were 1.33 × 10− 5, 4.46 × 10− 5 and 8.15 × 10− 8 M, respectively and it was illuminated at 25°C for 40 min. The photochemically reduced riboflavin generated O2√– which reduced NBT to form blue formazan. The unilluminated reaction mixture was used as a blank. The absorbance was measured at 560 nm. Di-DMP, Di-DIP and Di-DTP were added to the reaction mixture, in which O2√– was scavenged, thereby inhibiting NBT reduction. Decreased absorbance of the reaction mixture indicates increased superoxide anion scavenging activity. The inhibition percentage of superoxide anion generation was calculated by using the following formula:
where AC is the absorbance of the control and AS is the absorbance of Di-DMP, Di-DIP and Di-DTP or standards [Citation47].
Statistical analysis
The other analyses were performed in triplicate. The data were recorded as mean ± standard deviation and analysed by SPSS (version 11.5 for Windows 2000, SPSS Inc.). One-way analysis of variance was performed by ANOVA procedures. Significant differences between means were determined by Duncan's Multiple Range tests. P < 0.05 was regarded as significant and p < 0.01 as very significant.
Results and discussion
Di-DMP, Di-DIP and Di-DTP were synthesized by the procedure described by Ogata et al. [Citation29,Citation30]. Reaction of monomeric phenol derivatives 2,6-dimethylphenol, 2,6-diisopropylphenol and 2,6-di-t-butylphenol with catalytic CuCl(OH). TMEDA and then with Na2S2O4 gave the required dimeric phenol derivatives in Di-DMP, Di-DIP and Di-DTP in high yields ( and ). The obtained compounds were purified by crystallization to analytical grade (above 98%).
Figure 1 The structures of DMP, DIP and DTP and their synthesed dimeric compounds Di-DMP, Di-DIP and Di-DTP.
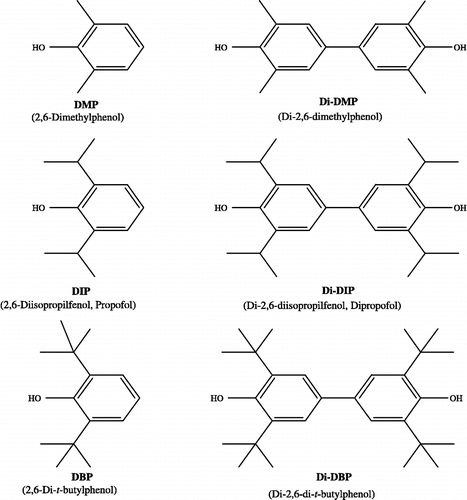
It has been reported in many studies, that natural antioxidants were closely related to their biofunctionalities, such as the reduction of chronic diseases like DNA damage, mutagenesis, carcinogenesis and inhibition of pathogenic bacterial growth which is often associated with the termination of free radical propagation in biological systems [Citation48,Citation49]. Antioxidant capacity is widely used as a parameter for medicinal bioactive components. In this study, the antioxidant activity of Di-DMP, Di-DIP and Di-DTP was compared to BHA, BHT α-tocopherol and its water-soluble analogue trolox. α-Tocopherol is a biological lipid antioxidant that prevents the formation of free radicals from lipid peroxidation [Citation50]. The antioxidant activity of Di-DMP, Di-DIP, Di-DTP, BHA, BHT, α-tocopherol and trolox were evaluated in a series of in vitro tests: 1, 1-diphenyl-2-picryl-hydrazyl free radical scavenging, ferric thiocyanate method, reducing power, scavenging of superoxide anion radical-generated non-enzymatic system, hydrogen peroxide scavenging and metal chelating activities.
Total antioxidant activity determination in a linoleic acid emulsion by the ferric thiocyanate method
Lipid peroxidation involves a series of free radical-mediated chain reactions and is also associated with several types of biological damage. The role of free radicals and ROS is becoming increasingly recognized in the pathogenesis of many human diseases, including cancer, aging, and atherosclerosis [Citation32,Citation51].
The thiocyanate method measures the amount of peroxide, which is the primary product of lipid oxidation, produced during the initial stages of oxidation. Total antioxidant activity of Di-DMP, Di-DIP, Di-DTP, BHA, BHT, α-tocopherol and trolox was determined by the ferric thiocyanate method in the linoleic acid system; Di-DMP, Di-DIP, Di-DTP and standard compounds exhibited effective antioxidant activity. The effects of the same concentration (45 μg/mL) of Di-DMP, Di-DIP and Di-DTP on lipid peroxidation of linoleic acid emulsion are shown in and were found to be 97.6, 95.2 and 98.8%, respectively, and their activities were greater than that of α-tocopherol (84.6%) and similar to trolox (95.6%) at this concentration.
Total reductive capability using the potassium ferricyanide reduction method
Antioxidants can be considered as reductants, and inactivation of oxidants by reductants are redox reactions where one reaction species is reduced at the expense of the oxidation of the other. The presence of reductants such as antioxidant substances in the antioxidant samples causes reduction of the Fe3+/ferricyanide complex to Fe2+ which can be monitored by measuring the formation of Perl's Prussian blue at 700 nm [Citation52,Citation53]. In this assay, the yellow colour of the test solution changes to various shades of green and blue depending on the reducing power of antioxidant samples. The reducing capacity of a compound may serve as a significant indicator of its potential antioxidant activity.
shows the reducing power of Di-DMP, Di-DIP, Di-DTP and standards (BHA, BHT, α-tocopherol and trolox) using the potassium ferricyanide reduction method. For the measurements of the reductive ability, the Fe3+-Fe2+ transformation was investigated in the presence of Di-DMP, Di-DIP and Di-DTP using the method of Oyaizu [Citation34].The reducing power of Di-DMP, Di-DIP, Di-DTP, BHA, BHT, α-tocopherol and trolox increased steadily with increasing concentration of sample. At different concentrations (15–45 μg/mL), Di-DMP, Di-DIP and Di-DTP showed an effective reducing power () and these differences were statistically significant (p < 0.05). The reducing power of Di-DMP, Di-DIP and Di-DTP and the standard compounds exhibited the following order: BHA ≈ BHT>Di-DIP>α-tocopherol ≈ Di-DMP>Di-DTP>trolox. The results on reducing power demonstrate the electron donor properties of Di-DMP, Di-DIP, Di-DTP thereby neutralizing free radicals by forming stable products. The outcome of the reducing reaction is to terminate the radical chain reactions that may otherwise be very damaging.
Ferrous ion (Fe2+) chelating capacity
Ferrous ion (Fe2+) chelation may lead to important antioxidative effects by retarding metal-catalysed oxidation. Ferrous ion chelating activities of Di-DMP, Di-DIP, Di-DTP, BHA, BHT, α-tocopherol and trolox are shown in . The effective ferrous ions chelator may also afford protection against oxidative damage by removing iron (Fe2+) that may otherwise participate in HO· generating Fenton type reactions. The chelating effect of ferrous ions by Di-DMP, Di-DIP, Di-DTP and standards was determined according to the method of Dinis [Citation36]. Among the transition metals, iron is known as the most important lipid oxidation pro-oxidant due to its high reactivity.
Table I. Comparison of hydrogen peroxide (H2O2) scavenging activity, ferrous ion (Fe2+) chelating activity and superoxide anion radicals (O2√–) scavenging activity of Di-DMP, Di-DIP, Di-DTP and the standard antioxidants BHA, BHT, α-tocopherol and trolox at concentration of 15 μg/mL.
Ferric (Fe3+) ions also produce radicals from peroxides although the rate is tenfold less than that of ferrous (Fe2+) ions [Citation55]. Ferrous ions (Fe2+) are the most powerful pro-oxidant among the various species of metal ions [Citation55,Citation56]. Minimizing ferrous ions (Fe2+) may afford protection against oxidative damage by inhibiting production of ROS and lipid peroxidation. Ferrozine can quantitatively form complexes with ferrous ions (Fe2+). In the presence of chelating agents, the complex formation is disrupted, resulting in a decrease in the red colour of the complex. Measurement of colour reduction therefore allows estimation of the metal chelating activity of the co-existing chelator; lower absorbance indicates higher metal chelating activity. In this assay, Di-DMP, Di-DIP and Di-DTP interfered with the formation of the ferrous and ferrozine complex, suggesting that they have chelating activity and are able to capture ferrous ion before ferrozine. It has been reported that compounds with structures containing a -OH functional group, a favourable structure–function configuration, can show metal chelation activity [Citation57,Citation58].
The difference between all the Di-DMP, Di-DIP and Di-DTP concentrations and the control was statistically significant (p < 0.01). In addition, Di-DMP, Di-DIP and Di-DTP exhibited 63.6 ± 4.7, 61.3 ± 4.2 and 58.2 ± 2.3% chelation of ferrous ion at 15 μg/mL concentration, respectively. On the other hand, the percentages of metal chelating capacity of the same concentration of BHA, BHT, α-tocopherol and trolox were found to be 69.9 ± 7.5, 60.0 ± 9.3, 31.3 ± 5.5and 45.2 ± 6.2%, respectively. The metal scavenging effect the compounds decreased in the order of BHA>Di-DMP>Di-DIP ≈ BHT ≈ Di-DTP>trolox>α-tocopherol.
Metal chelating capacity was significant since it reduced the concentration of the catalysing transition metal in lipid peroxidation. It was reported that chelating agents are effective antioxidants because they reduce the redox potential thereby stabilizing the oxidized form of the metal ion. The data in reveals that Di-DMP, Di-DIP and Di-DTP have a marked capacity for iron binding, suggesting that their main action as peroxidation protectors may be related to their iron binding capacity.
Hydrogen peroxide scavenging activity
Hydrogen peroxide can cross membranes and may slowly oxidize a number of compounds and it can be formed in vivo by many oxidizing enzymes such as superoxide dismutase. The ability of Di-DMP, Di-DIP and Di-DTP to scavenge hydrogen peroxide was determined according to the method of Ruch and co-workers [Citation39] () and compared with that of BHA, BHT, α -tocopherol and trolox as standards. At 15 μg/mL concentration, Di-DMP, Di-DIP and Di-DTP exhibited 38.2 ± 7.8, 30.3 ± 4.8 and 20.4 ± 3.9% scavenging activity whereas, BHA, BHT, α-tocopherol and trolox exhibited 36.4 ± 3.5, 34.3 ± 4.1, 39.3 ± 2.9 and 25.5 ± 3.3% activity at the same concentration, respectively. Hydrogen peroxide itself is not very reactive; however it can sometimes be toxic to the cell because it may give rise to the hydroxyl radical in the cell. Addition of hydrogen peroxide to cells in culture can lead to transition metal ion-dependent OH radicals mediated oxidative DNA damage. Levels of hydrogen peroxide at or below about 20–50 μg in the cell seem to have limited cytotoxicity to many cell types. Thus, removing hydrogen peroxide as well as superoxide anion is very important for protection of pharmaceutical and food systems.
Radical scavenging activity
Radical scavenging activity is very important due to the deleterious role of free radicals in foods and in biological systems. Excessive formation of free radicals accelerates the oxidation of lipids in foods and decreases food quality and consumer acceptance [Citation59]. Diverse methods are currently used to assess the antioxidant activity of plant phenolic compounds. Chemical assays are based on the ability to scavenge synthetic free radicals, using a variety of radical-generating systems and methods for detection of the oxidation end-point. ABTS or DPPH radical-scavenging methods are common spectrophotometric procedures for determining the antioxidant capacities of components. These chromogens (the violet DPPH radical and the blue oblique green ABTS radical cation) are easy to use, have a high sensitivity, and allow for rapid analysis of the antioxidant activity of a large number of samples. These assays have been applied to determine the antioxidant activity of food, wine and plant extracts and pure components Citation60-62.
DPPH has been widely used to evaluate the free radical scavenging effectiveness of various antioxidant substances [Citation63,Citation64]. In the DPPH assay, the antioxidants are able to reduce the stable radical DPPH to the yellow coloured diphenyl-picrylhydrazine. The method is based on the reduction of an alcoholic DPPH solution in the presence of a hydrogen-donating antioxidant due to the formation of the non-radical form DPPH-H by the reaction. DPPH is usually used as a reagent to evaluate free radical scavenging activity of antioxidants [Citation34]. DPPH is a stable free radical and accepts an electron or hydrogen radical to become a stable diamagnetic molecule [Citation65].
With this method it was possible to determine the radical scavenging power of an antioxidant by measuring the decrease in absorbance of DPPH· at 517 nm, resulting in a colour change from purple to yellow due to scavenging by an antioxidant through donation of hydrogen to form a stable DPPH· molecule [Citation66]. illustrates a significant decrease (p < 0.01) in the concentration of the DPPH radical due to the scavenging ability of Di-DMP, Di-DIP, Di-DTP and standards which decreased in the order of Di-DMP>Di-DIP>BHA>α-tocopherol>BHT>Di-DTP>trolox and were 75.5, 71.9, 67.8, 64.9, 62.5, 45.0 and 29.4%, at a concentration of 45 μg/mL, respectively. Free radical scavenging activity of these compounds also increased with increasing concentration.
Figure 5 DPPH· free radical scavenging activity of Di-DMP, Di-DIP and Di-DTP, α-tocopherol and trolox.
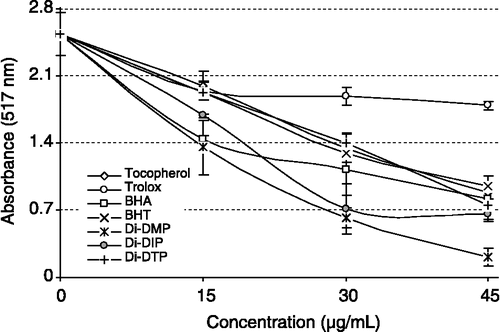
Generation of the ABTS radical cation forms the basis of one of the spectrophotometric methods that have been applied to the measurement of the total antioxidant activity of solutions of pure substances, aqueous mixtures and beverages [Citation54]. A more appropriate format for the assay is a decolorization technique in that the radical is generated directly in a stable form prior to reaction with putative antioxidants. The improved technique for the generation of ABTS√+ described here involves the direct production of the blue/green ABTS√+ chromophore through the reaction between ABTS and potassium persulfate.
All the tested compounds exhibited effective radical cation scavenging activity. As can seen from , Di-DMP, Di-DIP and Di-DTP had effective ABTS√+ radical scavenging activity in a concentration-dependent manner (15–45 μg/mL) which was significant (p < 0.01) and decreased in the order: Di-DIP ≈ BHA ≈ BHT>Di-DMP>α-tocopherol Di-DIP>trolox, which were 100, 100, 97.8, 96.9, 86.3, and 4.4%, at a concentration of 45 μg/mL, respectively.
Superoxide anions are a precursor to active free radicals that have the potential to react with biological macromolecules and thereby induce tissue damage [Citation55]. Also, they have been implicated in several pathophysiological processes due to their transformation into more reactive species such as hydroxyl radical that initiate lipid peroxidation. Superoxide has also been observed to directly initiate lipid peroxidation [Citation67]. It has also been reported that the antioxidant properties of some flavonoids are effective mainly via scavenging of superoxide anion radical [Citation68,Citation69]. Superoxide anion plays an important role in the formation of other ROS such as hydrogen peroxide, hydroxyl radical, and singlet oxygen, which induce oxidative damage in lipids, proteins, and DNA [Citation70]. Also, superoxide anion is an oxygen-centred radical with selective reactivity. These species are produced by a number of enzyme systems in auto-oxidation reactions and by non-enzymatic electron transfers that univalently reduce molecular oxygen. It can also reduce certain iron complexes such as cytochrome c.
Superoxide radical is normally initially formed, and its effects can be magnified because it produces other kinds of free radicals and oxidizing agents [Citation71]. Superoxide anions derived from dissolved oxygen by riboflavin/methionine/illumination system can reduce NBT. In this method, superoxide anion reduces the yellow dye (NBT2+) to produce the blue formazan which is measured spectrophotometrically at 560 nm. Antioxidants are able to inhibit the blue NBT formation [Citation72,Citation73]. The decrease of absorbance at 560 nm in the presence of antioxidants indicates the consumption of superoxide anion in the reaction mixture. shows the percentage inhibition of superoxide radical generation in the presence of 15 μg/mL concentration of Di-DMP, Di-DIP and Di-DTP which was found to be 67.4 ± 5.2, 33.7 ± 5.2 and 33.1 ± 6.3%, respectively. At the same concentration BHA, BHT, α-tocopherol and trolox exhibited 75.3 ± 6.5, 70.2 ± 7.1, 22.2 ± 3.3 and 16.0 ± 1.9% superoxide anion radical scavenging activity, respectively.
Conclusion
Di-DMP, Di-DIP and Di-DTP were found to be effective antioxidants in different in vitro assays including reducing power, DPPH radical, ABTS radical and superoxide anion radical scavenging, hydrogen peroxide scavenging and metal chelating activities when compared to the standard antioxidants α-BHA, BHT, tocopherol (a natural antioxidant) and trolox, which is a water-soluble analogue of tocopherol. The new compounds had better antioxidant and radical scavenging activity than the used standards. These dimeric antioxidants can be potentially used for minimizing or preventing lipid oxidation in food products, retarding the formation of toxic oxidation products, maintaining nutritional quality and prolonging the shelf life of foods and pharmaceuticals.
Abbreviations | ||
di(2,6-dimethylphenol: | = | Di-DMP |
di(2,6-diisopropylphenol): | = | Di-DIP, dipropofol |
di(2,6-di-t-butylphenol): | = | Di-DTP |
1,1-diphenyl-2-picryl-hydrazyl: | = | DPPH· |
2,2′-azino-bis/3-ethylbezthiazoline-b-sulfonic acid: | = | ABTS |
butylated hydroxytoluene: | = | BHT |
butylated hydroxyanisole: | = | BHA |
Acknowledgements
This study was partially supported by Atatürk University Research Foundation (Project no: 2001/35). The authors are grateful to the Research Fund of Atatürk University for financial support.
References
- Rahman I, Biswas SK, Kode A. Oxidant and antioxidant balance in the airways and airway diseases. Eur J Pharmacol 2006; 533: 222–239
- Ramarathnam N, Osawa T, Ochi H, Kawakishi S. The contribution of plant food antioxidants to human health. Trends Food Sci Technol 1995; 6: 75–82
- Chandrika M, Pathirana L, Shahidi F. Antioxidant properties of commercial soft and hard winter wheats (Triticum aestivum L.) and their milling fractions. J Sci Food Agric 2006; 86: 477–485
- Lin TJ, Liu GT, Liu Y, Xu GZ. Protection by salvianolic acid A against adriamycin toxicity on rat heart mitochondria. Free Radical Biol Med 1992; 12: 347–351
- Halliwell B, Gutteridge JM. Free radicals in biology and medicine. Clarendon Press, Oxford 1989; 23–30
- Gülçin İ, Büyükokuroğlu ME, Oktay M, Küfrevioğlu Öİ. On the in vitro antioxidant properties of melatonin. J Pineal Res 2002; 33: 167–171
- Gülçin İ, Oktay M, Küfrevioğlu Öİ, Aslan A. Determination of antioxidant activity of lichen Cetraria islandica (L)Ach. J Ethnopharmacol 2002; 79: 325–329
- Masuoka N, Isobe T, Kubo I. Antioxidants from Rabdosia japonica. Phytother Res 2006; 20: 206–213
- Kinnula VL, Crapo JD, Raivio KO. Biology of disease: Generation and disposal of reactive oxygen metabolites in the lung. Lab Invest 1995; 73: 3–19
- Holland JA, Pritchard KA, Pappolla MA, Wolin MS, Rogers NJ, Stemerman MB. Bradykinin induces superoxide anion release from human endothelial cells. J Cell Physiol 1990; 143: 21–25
- Kinnula VL, Chang L, Everitt JI, Crapo JD. Oxidants and antioxidants in alveolar epithelial type II cells: In situ, freshly isolated, and cultured cells. Am J Physiol 1992; 262: 69–77
- Janssen YMW, van Houten B, Borm PJA, Mossman BT. Cell and tissue responses to oxidative damage. Lab Invest 1993; 69: 261–274
- Oktay M, Gülçin İ, Küfrevioğlu Öİ. Determination of in vitro antioxidant activity of fennel (Foeniculum vulgare) seed extracts. Lebensm-Wiss Technol 2003; 36: 263–271
- Church DF, Prior WA. Free-radical chemistry of cigarette smoke and its toxicological implications. Environ Health Perspect 1985; 64: 111–126
- Halliwell B, Aruoma OI. DNA damage by oxygen-derived species its mechanisms and measurement in mammalian systems. FEBS Lett 1991; 281: 9–19
- Duh PD, Tu YY, Yen GC. Antioxidant activity of water extract of harng jyur (Chrysanthemum morifolium Ramat). Lebensm-Wiss Technol 1999; 32: 269–277
- Büyükokuroğlu ME, Gülçin İ, Oktay M, Küfrevioğlu Öİ. In vitro antioxidant properties of dantrolene sodium. Pharmacol Res 2001; 44: 491–495
- Gülçin İ. Antioxidant activity of caffeic acid (3,4-dihydroxycinnamic acid). Toxicology 2006; 217: 213–220
- Liyana-Pathirana CM, Shahidi F. Antioxidant properties of commercial soft and hard winter wheats (Triticum aestivum L.) and their milling fractions. J Sci Food Agric 2006; 86: 477–485
- Velioglu YS, Mazza G, Gao L, Oomah BD. Antioxidant activity and total phenolics in selected fruits, vegetables and grain products. J Agr Food Chem 1998; 46: 4113–4117
- Aruoma OI. Free radicals, oxidative stress, and antioxidants in human health and disease. J Am Oil Chem Soc 1998; 75: 199–212
- Gülçin İ, Oktay M, Kireçci E, Küfrevioğlu Öİ. Screening of antioxidant and antimicrobial activities of anise (Pimpinella anisum L.) seed extracts. Food Chem 2003; 83: 371–382
- Kinsella JE, Frankel E, German B, Kanner J. Possible mechanism for the protective role of the antioxidant in wine and plant foods. Food Technol 1993; 47: 85–89
- Lai LS, Chou ST, Chao WW. Studies on the antioxidative activities of Hsian-tsao (Mesona procumbens Hemsl) leaf gum. J Agr Food Chem 2001; 49: 963–968
- Pryor WA. The antioxidant nutrient and disease prevention – What do we know and what do we need to find out?. Am J Clin Nut 1991; 53: 391–393
- Sherwin ER. Food additives, Branen AL, Davidson PM, Salminen S, Marcel Dekker inc: New York; 1990. p 139–193
- Sun B, Fukuhara M. Effects of co-administration of butylated hydroxitoluene, butylated hydroxyanisole and flavonoids on the activation of mutagens and drugmetabolizing enzymes in mice. Toxicology 1997; 122: 61–72
- Gülçin İ, Alici HA, Cesur M. Determination of in vitro antioxidant and radical scavenging activities of propofol. Chem Pharm Bull 2005; 53: 281–285
- Ogata M, Shin-Ya K, Urano S, Endo T. Antioxidant activity of propofol and related monomeric and dimeric compounds. Chem Pharm Bull 2005; 53: 344–346
- Ogata M, Sato KT, Kunikane T, Oka K, Seki M, Urano S, Hiramatsu K, Endo T. Antibacterial activity of dipropofol and related compounds. Biol Pharm Bull 2005; 28: 1120–1122
- Mitsuda H, Yuasumoto K, Iwami K. Antioxidation action of indole compounds during the autoxidation of linoleic acid. Eiyo to Shokuryo 1996; 19: 210–214
- Gülçin İ. Antioxidant and antiradical activities of L-Carnitin. Life Sci 2006; 78: 803–811
- Gülçin İ, Şat İG, Beydemir Ş, Elmastaş M, Küfrevioğlu Öİ. Comparison of antioxidant activity of clove (Eugenia caryophylata Thunb) buds and lavender (Lavandula stoechas L.). Food Chem 2004; 87: 393–400
- Oyaizu M. Studies on product of browning reaction prepared from glucose amine. Jpn J Nut 1986; 44: 307–315
- Gülçin İ, Beydemir Ş, Şat İG, Küfrevioğlu Öİ. Evaluation of antioxidant activity of cornelian cherry (Cornus mas L.). Acta Aliment Hung 2005; 34: 193–202
- Dinis TCP, Madeira VMC, Almeida LM. Action of phenolic derivates (acetoaminophen, salycilate, and 5-aminosalycilate) as inhibitors of membrane lipid peroxidation and as peroxyl radical scavengers. Arch Biochem Biophys 1994; 315: 161–169
- Gülçin İ, Şat İG, Beydemir Ş, Küfrevioğlu Öİ. Evaluation of the in vitro antioxidant properties of extracts of broccoli (Brassica oleracea L.). Ital J Food Sci 2004; 16: 17–30
- Elmastaş M, Gülçin İ, Beydemir Ş, Küfrevioğlu Öİ, Aboul-Enein HY. A study on the in vitro antioxidant activity of juniper (Juniperus communis L.) seeds extracts. Anal Lett 2006; 39: 47–65
- Ruch RJ, Cheng SJ, Klaunig JE. Prevention of cytotoxicity and inhibition of intracellular communication by antioxidant catechins isolated from chinese green tea. Carcinogenesis 1989; 10: 1003–1008
- Elmastas M, Türkekul İ, Öztürk L, Gülçin İ, Işıldak Ö, Aboul-Enein HY. The antioxidant activity of two wild edible mushrooms (Morchella vulgaris and Morchella esculanta). Comb Chem High T Scr 2006; 6: 443–448
- Blois MS. Antioxidant determinations by the use of a stable free radical. Nature 1958; 26: 1199–1200
- Gülçin İ. The antioxidant and radical scavenging activities of black pepper (Piper nigrum) seeds. Int J Food Sci Nut 2005; 56: 491–499
- Gülçin İ, Beydemir Ş, Alici HA, Elmastaş M. In vitro antioxidant properties of morphine. Pharmacol Res 2004; 49: 59–66
- Re R, Pellegrini N, Proteggente A, Pannala A, Yang M, Rice-Evans C. Antioxidant activity applying an improved ABTS radical cation decolorization assay. Free Radical Bio Med 1999; 26: 1231–1237
- Beauchamp C, Fridovich I. Superoxide dismutase: Improved assays and an assay applicable to acrylamide gels. Anal Biochem 1971; 44: 276–287
- Zhishen J, Mengcheng T, Jianming W. The determination of flavonoid contents on mulberry and their scavenging effects on superoxide radical. Food Chem 1999; 64: 555–559
- Gülçin İ, Küfrevioğlu Öİ, Oktay M, Büyükokuroğlu ME. Antioxidant, antimicrobial, antiulcer and analgesic activities of nettle (Urtica dioica L.). J Ethnopharmacol 2004; 90: 205–215
- Covacci V, Torsello A, Palozza P, Sgambato A, Romano G, Boninsegna A, Cittadini A, Wolf FI. DNA oxidative damage during differentiation of HL-60 human promyelocytic leukemia cells. Chem Res Toxicol 2001; 14: 1492–1497
- Zhu QY, Hackman RM, Ensunsa JL, Holt RR, Keen CL. Antioxidative activities of oolong tea. J Agr Food Chem 2002; 50: 6929–6934
- Tavan E, Mazier S, Narbonne JF, Cassand P. Effects of vitamin A and E on methylazoxymethanol-induced mutagenesis in Salmonella typhimurim strains TA100. Mutat Res 1997; 377: 231–237
- Perry G, Raina AK, Nonomura A, Wataya T, Sayre LM, Smith MA. How important is oxidative damage? Lessons from Alzheimer's disease. Free Radical Bio Med 2000; 28: 831–834
- Chung YC, Chang CT, Chao WW, Lin CF, Chou STJ. Antioxidative activity and safety of the 50% ethanolic extract from red bean fermented by Bacillus subtilis IMR-NK1. Agr Food Chem 2002; 50: 2454–2458
- Gülçin İ, Elias R, Gepdiremen A, Boyer L. Antioxidant activity of lignans from fringe tree (Chionanthus virginicus L.). Eur Food Res Technol 2006; 223: 759–767
- Miller DD. Mineral. Food chemistry, OR Fennema. Marcel Deckker, New York 1996; 618–649
- Halliwell B, Gutteridge JMC. Oxygen toxicology, oxygen radicals, transition metals and disease. Biochem J 1984; 219: 1–4
- Gülçin İ, Berashvili D, Gepdiremen A. Antiradical and antioxidant activity of total anthocyanins from Perilla pankinensis decne. J Ethnopharmacol 2005; 101: 287–293
- Lindsay RC. Food additives. Food chemistry, OR Fennema. Marcel Dekker Inc, New York 1996; 778–780, Chapter 12
- Yuan YV, Bone DE, Carrington MF. Antioxidant activity of dulse (Palmaria palmata) extract evaluated in vitro. Food Chem 2005; 91: 485–494
- Min DB. Lipid oxidation of edible oil. Food lipids chemistry, nutrition, and biotechnology, CC Akoh, DB Min. Marcel Dekker, New York 1998; 283–296
- Awika JM, Rooney LW, Wu X, Prior RL, Cisneros-Zevallos L. Screening methods to measure antioxidant activity of Sorghum (Sorghum bicolor) and sorghum product. J Agr Food Chem 2003; 51: 6657–6662
- van den Berg R, Haenen GRMM, van den Berg H, van den Vijgh W, Bast A. The predictive value of the antioxidant capacity of structurally related flavonoids using trolox equivalent antioxidant capacity (TEAC) assay. Food Chem 2000; 70: 391–395
- Yu L, Halley S, Perret J, Harris M, Wilson J, Qian MJ. Free radical scavenging properties of wheat extracts. Agr Food Chem 2002; 50: 1619–1624
- Cotelle N, Bemier JL, Catteau JP, Pommery J, Wallet JC, Gaydou EM. Antioxidant properties of hydroxyl-flavones. Free Radical Bio Med 1996; 20: 35–43
- Özcelik B, Lee JH, Min DB. Effects of light, oxygen and pH on the 2,2-diphenyl-1-picrylhydrazyl (DPPH) method to evaluate antioxidants. J Food Sci 2003; 68: 487–490
- Soares JR, Dins TCP, Cunha AP, Ameida LM. Antioxidant activity of some extracts of Thymus zygis. Free Radical Res 1997; 26: 469–478
- Matthäus B. Antioxidant activity of extracts obtained from residues of different oilseeds. J Agr Food Chem 2002; 50: 3444–3452
- Wickens AP. Aging and the free radical theory. Resp Physiol 2001; 128: 379–391
- Yen GC, Duh PD. Scavenging effect of methanolic extract of peanut hulls on free radical and active oxygen species. J Agr Food Chem 1994; 42: 629–632
- Gülçin İ, Mshvildadze V, Gepdiremen A, Elias R. Screening of antioxidant and antiradical activity of monodesmosides and crude extract from Leontice smirnowii tuber. Phytomedicine 2006; 13: 343–351
- Pietta PG. Flavonoids as antioxidants. J Nat Prod 2000; 63: 1035–1042
- Liu F, Ooi VEC, Chang ST. Free radical scavenging activities of mushroom polysaccharide extracts. Life Sci 1997; 60: 763–771
- Cos P, Ying LY, Calomme M, Hu JH, Cimanga K, Van Poel B, Pieters L, Vlietinck AJ, Berghe DV. Structure activity relationships and classification of flavonoids as inhibitors of xanthine oxidase and superoxide scavengers. J Nat Prod 1998; 61: 71–76
- Parejo I, Viladomat F, Bastida J, Rosas-Romero A, Flerlage N, Burillo J, Codına C. Comparison between the radical scavenging activity and antioxidant activity of six distilled and nondistilled mediterranean herbs and aromatic plants. J Agr Food Chem 2002; 50: 6882–6890