Abstract
The activities of the novel aminopeptidase N inhibitor (APNI), β-Amino-α-Hydroxyl-Phenyl butanic acid-Valine (AHPA-Val), were compared with APNI (amastatin). AHPA-Val and amastatin produced competitive inhibition of the hydrolysis of Tyr-Gly in the guinea-pig striatal membrane preparation, with Ki equal to 14.06 μM and 12.48 μM respectively. Met-enkephalin-induced twitch inhibition of the guinea-pig ileum preparation was enhanced by AHPA-Val and amastatin with pA1/2 values (the negative logarithm concentration of APNI that decreased the IC50 of Met-enkephalin by half), of 7.08 and 7.79 respectively. These results suggest that AHPA-Val has good activity as an APNI and that these two assay systems are useful for evaluating the potency of novel APNIs.
Introduction
Met-enkephalin, an endogenous opioid peptide, is produced in the central nervous system as well as in the pituitary and adrenal glands and is known to act primarily as a neuromodulator in pain transmission [Citation10]. Xu et al. [Citation22] showed that Met-enkephalin was also an immunomodulator that could abolish virus-induced over-expression of caspase-3; over-expression of caspase-3 encourages human immunodeficiency virus infection. Met-enkephalin also possesses anti-tumor properties. Jaglowski et al. [Citation9] showed that Met-enkephalin enhanced the inhibition of tumor growth, suggesting that Met-enkephalin administration combined with an anti-cancer drug (i.e., paclitaxel) might provide a valuable alternative to the standard therapy in the treatment of squamous cell carcinoma of the head and neck.
However, like most other peptides, the therapeutic use of Met-enkephalin for various disease conditions has been restricted because of its rapid degradation in biological systems. Three enzymes, i.e., aminopeptidase N (APN, CD13, EC 3.4.11.2), dipeptidyl carboxypeptidase (3.4.15.1) and neutral endopeptidase (EC 3.4.24.11), have been shown to participate in the degradation of Met-enkephalin at different sites of peptide linkages. Previous studies showed that the mixed peptidase inhibitors strongly potentiated Met-enkephalin-induced effects. For example, Met-enkephalin-induced antiniciception was augmented by its intracerebroventricular- coadministration with three peptidase inhibitors amastatin (APNI), captopril (carboxypeptidase inhibitor), and phosphoramidon (neutral endopeptidase inhibitor) [Citation11,Citation18]. In facts, Glavas et al. [Citation4] demonstrated a significant suppression of tumor cell growth by a combination of Met-enkephalin and the peptidase inhibitors.
Originally, neutral endopeptidase was considered to be the major enzyme that inactivates Met-enkephalin through the hydrolysis of the Gly-Phe bond [Citation20]. However, APN, which hydrolyses the Tyr-Gly bond in Met-enkephalin, was shown to have the dominant role in Met-enkephalin hydrolysis in the peripheral tissue as well as the brain [Citation12], and cerebral microvascular aminopeptidase N was suggested to be the most important for the metabolism of low molecular weight opioid peptidases [Citation1]. Additionally, Jaba et al. [Citation8] also reported that the Tyr residue at the N-terminal sequence has the necessary minimal structure for enkephalin-like peptides to show strong analgesic activity.
Inhibition of APN can block the inactivation of Met-enkephalin and allow potentiation of the antinociceptive and anti-tumor property of Met-enkephalin [Citation3], which reinforces our early physiological approach aimed at developing new analgesics and antineoplastics. Different classes of natural and small molecular aminopeptidase inhibitors (APNIs) have been synthesized. APNI can covalently bind to the catalytic site of APN and the first natural inhibitor, bestatin, has been available to treat some diseases [Citation13].
Recently, we synthesized a new bestatin derivative, named β-Amino-α-Hydroxyl-Phenyl butanic acid-Valine (AHPA-Val), which contains a carbonyl group as a zinc coordinating ligand, a free N-terminal amino group, and an aliphatic chain, fulfilling the requirements for selective recognition of APN. In this study, we investigated the potency of AHPA-Val, compared with that of the representative APN inhibitor, amastatin. Additionally, we established two assay systems to evaluate newly synthesized APNIs.
Materials and methods
Animals
Male Hartley guinea-pigs weighing 200 to 300 g were obtained from Kiwa laboratory animals Co. Ltd. (Wakayama, Japan). The guinea-pigs were housed two per cage at a temperature of 23–24°C with a light-dark cycle 12 h: 12 h (light on 8:00 a.m. to 8:00 p.m.), and were given food and water ad libitum. The present study was conduced in accordance with the Guiding Principles for the Care and Use of Laboratory Animals, adopted by the Wakayama Medical University (Approved No. 205).
Chemicals
AHPA-Val (general formula is H3N+-CH(CH2Ph)-CH(OH)-CONH-CH[CH(CH3)2]-COOCH3 · Cl–), was provided by the Department of Medicinal Chemistry of Shandong University (Shandong, China). Other reagents were purchased from the following sources: Met-enkephalin, amastatin, phosphoramidon (Peptide Institute. Inc, Osaka, Japan); captopril (Research Biochemical Inc, Natick, USA); Tyr-Gly (Bachem. A G, Bubendorf, Switzerland); Tyr (Wako Pure Chemical Industries, Ltd, Osaka, Japan). All chemicals were dissolved in distilled water. The stock solution for all reagents used was prepared at concentrations of 0.1 to 10 mM in siliconizing plastic tubes. Met-enkephalin, amastatin, phosphoramidon, captopril and APHA-Val were stored at − 80°C. Tyr-Gly and Tyr were stored at 4°C. All reagents were diluted to the desired concentration just before used.
Preparation of membrane fractions
The striatum of guinea pig brain was dissected by the method of Glowinski and Lyerson [Citation5]. Membrane fractions of the striatum were prepared as previously described [Citation6]. Briefly, isolated striatum weas cut into small pieces in 50 mM Tris-HCl buffer, pH7.4, and then homogenized in 15 volumes of this buffer with a Teflon-glass homogenizer. The homogenate was diluted to a 3% suspension and centrifuged at 800 × g for 15 min. The supernatant was collected. The pellet was resuspended with 5 mL of buffer, and the suspension was centrifuged at 800 × g for 15 min. The supernatant was combined with initial supernatant. The combined supernatant was centrifuged at 49 000 × g for 10 min. The resulting pellet was washed three times by suspension in buffer and centrifugation. The washed pellet was suspended and adjusted with the buffer to a concentration of 2 mg/mL of protein, which yielded the sample used in the following experiment as the membrane fraction. The protein concentration was determined by the Coomassie (Bradford) Protein Assay Kit (PIERCE, Rockford, USA), with bovine serum albumin as the standard. All steps above were carried out at 4°C.
Measurement Of Tyr-gly And Tyr By Hplc-ecd
The sample of membrane fraction (0.5 mg of protein) in 50 mM Tris-HCl buffer was incubated with different amounts of Tyr-Gly (25 nmol, 5 nmol, 50 nmol and 100 nmol) in either the absence or the presence of APN inhibitors at 37°C in a total volume of 0.5 mL. The reaction was stopped by the application of 0.5 mL of 10% trichloroacetic acid, followed by centrifugation at 3000 rpm for 10 min. Samples (20 μL) of supernatant were then analyzed by HPLC-ECD.
The HPLC-ECD apparatus consists of the following: a LC-10AD pump (Shimadzu); a column (4.6 × 250 mm, Eicompak MA-ODS, Eicom, Kyoto, Japan); a Waters 712 WISP sample injector (Millipore) and a Coulochem II electrochemical analyzer (550 mV, ESA). The chromatographic mobile phase was 0.1 M phosphate buffer at pH 3.0 containing 2.5% acetonitrile for the Tyr-Gly and Tyr assays, at a flow rate of 0.8 mL/min. Under these conditions, the retention time of Tyr-Gly and Tyr are15.1 min and 9.6 min, respectively.
The content of each compound was determined by comparing the peak heights in the sample with the standard. Working standards were run at regular intervals to check the sensitivity of the detector.
Isolated preparation of guinea-pig ileum
The myenteric plexus-longitudinal muscle strip of guinea pig ileum was prepared and set up for electrical stimulation as described previously [Citation14]. Briefly, the myenteric plexus-longitudinal muscle was peeled from segments (2–3 cm long) of the guinea pig ileum, and was mounted between two electrodes, consisting of platinum rod (bottom) and a circular platinum wire (top) with 1 g as resting tension in 5-mL isolated tissue baths containing Krebs' solution of the following composition (g/L concentrations): NaCl, 6.93; KCl, 0.35; CaCl2·2H2O, 0.37; KH2PO4, 0.16; MgSO4·7H2O, 0.29; NaHCO3, 2.10; and Glucose, 2.0. In all experiments, tissues were maintained at 37°C and equilibrated with the mixed gas (95% O2–5% CO2).
Electrically evoked twitch response and calculations
Tissues were stimulated with rectanglar pulses (0.1 Hz) of supramaximal voltage and 1 millisecond duration, provided by an electronic stimulator (NihonKoden, SEN-7203, Japan). Met-enkephalin (10 μL of application volume) was added to the baths in a cumulative fashion to evaluate inhibition of the electrically evoked twitch response. The degree of the guinea-pig ileum twitch response was measured before and after drug application. The percentage of inhibition induced by Met-enkephalin was calculated from the following formula: % inhibition = [1 - (the contractile height after drug administration/the contractile height before drug administration)] × 100. The percent inhibition of the electrically evoked muscle twitch response by Met-enkephalin was plotted against the log concentration of the Met-enkephalin to estimate the IC50 (concentration of the Met-enkephalin to produce 50% inhibition of the ileum twitch). When the effect of APN inhibitor on the IC50 was studied, each concentration of APN inhibitor, in the presence of phosphoramidon (1 μM) and captopril (1 μM), was given 10 min before the application of Met-enkephalin.
For an analytical method of the efficacy of an antagonist for its receptor, pA2 analysis [Citation17] is useful. To evaluate the activity of AHPA-Val, we modified the pA2 analysis to a pA1/2 analysis in the present experiment, which is defined as the negative log concentration of the peptidase inhibitor that decreased the IC50 of Met-enkephalin in half.
Quantitative structure- activity relationship (QSAR) research
We applied FlexX [Citation15] in Sybyl software (Dell Precision Workstation 360N) to dock the compound AHPA-Val and identified their conformations as well as their orientations. The coordinate for bestatin was taken from the Protein Data Bank (PDB, PDB code: 1HS6).
Statistical analysis
The unpaired t-test was used to analyze the statistical significance. Data are represented the mean with S.E.M (the mean of standard error). P < 0.05 was considered statistically significant.
Results
Tyr-Gly hydrolysis during the incubation with striatal membrane fractions
To estimate the potency of AHPA-Val, we used the dipeptide Tyr-Gly as the substrate for APN. Tyr-Gly (50 nmol) was incubated in a membrane fraction (0.5 mg/mL protein) prepared from the striatum of guinea pig brain. The amounts of Tyr-Gly and its hydrolysis product, Tyr, after the incubation were estimated and a submaximal reaction was observed at 2 h (see ).
Figure 1 The hydrolysis of Tyr-Gly in striatum membrane preparations. (A) Time course of the contents of Tyr-Gly (○) and of its hydrolysis product Tyr (•) with 0.5 mg/mL protein. Each point represents the mean with S.E.M. of 5 independent experiments. (B) Protein concentration- dependence of the change in the contents of Tyr-Gly (▪) and Tyr (▪) in 2 h incubation period. Each column represents the mean with S.E.M. of 5 independent experiments. When the error bars are not shown, they are smaller than the symbol.
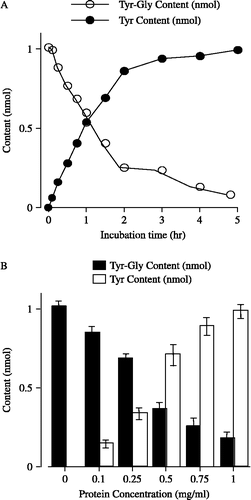
shows the effects of protein concentration on Tyr-Gly hydrolysis at a 2 h incubation time. Tyr-Gly (50 nmol) was incubated in the membrane fractions at various protein concentrations. The change in the amounts of Tyr-Gly and Tyr confirmed protein concentration dependence, with a moderate effect being observed at the concentration of 0.5 mg/mL protein.
For the subsequent experiments in this study, we determined the inhibitory effects of APNIs on the hydrolysis of Tyr-Gly at 0.5 mg/mL protein for a 2 hour incubation period, using the parameters described above.
Effects of APNIs on the hydrolysis of Tyr-Gly
The inhibitory potency of AHPA-Val or amastatin on Tyr-Gly hydrolysis was studied using membrane fraction preparations. Amastatin (0.01 μM–1000 μM) concentration-dependently increased the content of Tyr-Gly, and concomitantly decreased the amount of its hydrolysis product Tyr (see ), indicating that amastatin significantly inhibited the hydrolysis of Tyr-Gly by the inhibition of APN activity. Additionally, the contents of Tyr-Gly and Tyr in the preparations pretreated with AHPA-Val were qualitatively similar to, but quantitatively different from, those in the presence of amastatin (see ). Kinetic analyses were then carried out to demonstrate the inhibitory mechanism of the APNIs. We adopted commonly used standard linear transformation, analyzed by the Lineweaver-Burk plot,. These data indicated that amastatin and AHPA-Val increased apparent Km values compared with controls, whereas the apparent Vmax remained constant in the absence or presence of the inhibitors (see and B). The K i values were 12.48 μM and 14.06 μM for amastatin and AHPA-Val, respectively, indicating that the potency of AHPA-Val to inhibit the hydrolysis of Tyr-Gly in striatal membrane fractions was slightly lower than that of amastatin.
Figure 2 Effects of amastatin and AHPA-Val on the amounts of Tyr-Gly and its hydrolysis product Tyr in striatum membrane preparations, respectively. Each point represents the mean and vertical bar indicates the S.E.M. of 6 ∼ 8 preparations. For the “veh”, we incubated Tyr-Gly in Tris-HCl buffer without protein and APNIs. Vs. respective concentration of amastatin, * p < 0.05, *** p < 0.001 (unpaired t-test).
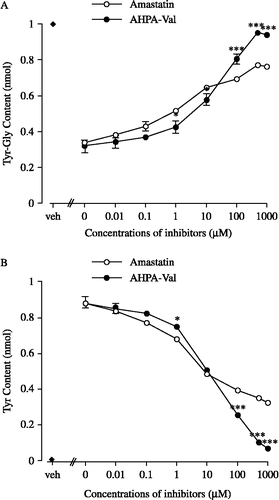
Figure 3 Kinetic analyses of amastatin (A) and AHPA-Val (B) on the hydrolysis of Tyr-Gly using Lineweaver-Burk plot of 1/V against 1/[S]. The inhibitory potencies of AHPA-Val and amastatin were measured using Tyr-Gly as substrate with the concentrations indicated (0.05 mM, 0.1 mM and 0.2 mM). Each point represents the mean of five separate experiments.
![Figure 3 Kinetic analyses of amastatin (A) and AHPA-Val (B) on the hydrolysis of Tyr-Gly using Lineweaver-Burk plot of 1/V against 1/[S]. The inhibitory potencies of AHPA-Val and amastatin were measured using Tyr-Gly as substrate with the concentrations indicated (0.05 mM, 0.1 mM and 0.2 mM). Each point represents the mean of five separate experiments.](/cms/asset/b1529047-e521-4a7b-ba57-3d4b2d88685a/ienz_a_251421_f0003_b.gif)
On the other hand, the submaximal inhibitory concentration of amastatin and AHPA-Val was approximately 500 μM (see ). In the presence of 500 μM AHPA-Val, 95.2% of Tyr-Gly remained intact, indicating that the hydrolysis of Tyr-Gly in striatum membrane fraction was almost completely prevented. In contrast to AHPA-Val, 76.1% of Tyr-Gly was detected in the sample in the presence of 500 μM amastatin. When the concentration of inhibitor was below 10 μM, Tyr-Gly in the presence of AHPA-Val was less than that in the presence of amastatin. However, as the dose up to 10 μM or more, more Tyr-Gly remained intact in the presence of AHPA-Val than in the presence of amastatin.
Potentiating effect of APNIs on the twitch inhibition of Met-enkephalin in guinea pig ileum preparation
In the presence of captopril (10− 6 M) and phosphoramidon (10− 6 M), the IC50 value of Met-enkephalin-induced twitch inhibition was repeatedly estimated in each concentration of APNIs (see ). The IC50 values of Met-enkephalins in the various concentrations of either amastatin or AHPA-Val were significantly lower than the IC50 value of the control (see ).
Figure 4 Effects of amastatin (A) and AHPA-Val (B) on Met-enkephalin-induced twitch inhibition in guinea pig ileum preparations. Peptidase inhibitors were applied accumulatively. Each point represents the mean percentage inhibition with S.E.M of 9 independent experiments.
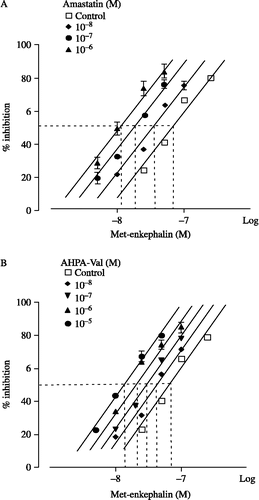
Table I. Effects of amastatin or AHPA-Val on the IC50 values of Met-enkephalin- induced twitch inhibition in guinea pig ileum preparations.
As determined by percent inhibition, amastatin significantly enhanced the inhibitory effect of Met-enkephalin on the electrically evoked-contractions of myenteric plexus longitudinal muscle preparation of guinea-pig at the concentration of 10− 8 M–10− 6 M. The concentration- response curve of Met-enkephalin shifted 5.64-fold to the left with the enhancing effect of amastatin 10− 6 M (see ). AHPA-Val also enhanced the inhibitory effect of Met-enkephalin at the concentration of 10− 8 M–10− 5 M and the concentration-response curve shifted 4.92-fold to the left with the enhancing effect of AHPA-Val 10− 5 M (see ).
As shown in , amastatin and AHPA-Val enhanced the inhibitory effect of Met-enkephalin with pA1/2 values of 7.79 and 7.08, respectively.
Figure 5 pA1/2 analyses of amastatin (▪) and AHPA-Val (•). pA1/2 value is defined as the negative log concentration of peptidase inhibitor that produced the effect to decrease the IC50 of Met-enkephalin in half. pA1/2 of amastatin and AHPA-Val is 7.79 and 7.08, respectively. Each point represents the mean of 9 independent experiments.

Discussion
Inhibition of APN has been of interest since the isolation of bestatin, a natural product found in culture filtrates of Streptomyces olivoreticuli, that potently inhibits aminopeptidase enzymes from a variety of sources. In the present study, we report that an analogue of bestatin, AHPA-Val, is able to suppress significantly the cleavage of the Tyr-Gly bond in Met-enkephalin.
APN, a member of the M1 family of zinc metallopeptidase [Citation16], is anchored to the cell membrane by a transmembrane helical region near the N-terminus; a small region of N-terminus (8–10 amino acids) protrudes to the cytoplasm. APN can catalyze the removal of the Tyr residue from Met-enkephalin and decrease the biological activity of Met-enkephalin. Structure-based drug design methods have played a critical role in the discovery of APNIs. Many computational methods were applied to research the correlations between the binding energy of inhibitors to APN and their inhibitory activity. The ligand-protein complex X-ray structures for bestatin (PDB code:1HS6) [Citation19] is shown in (a) The hydroxyl group and carbonyl group of bestatin bind to the zinc ion in the active pocket with a regularly spaced length of 2.09 Å and 2.51 Å, respectively. The superimposition of the structure with the corresponding crystal structure shown in gray validated the rationality and reliability of our molecular simulation, and revealed that the FlexX procedure was able to correctly reproduce the obtained complex [Citation2]. The next step was to generate the inhibitor-enzyme complex for AHPA-Val by the FlexX docking procedure. (b) is the result of docking with the FlexX procedure, showing the binding mode of AHPA-Val docked to 1HS6. Analyzing the stereoview and the feature of binding pocket, we reach the following conclusions: 1) The inhibitor occupies the active site of 1HS6. The amino group and carbonyl group bind to the zinc ion with a regularly spaced length of 2.64 Å and 1.91 Å, respectively, which is different from bestatin. 2) The aryl ring of AHPA-Val, is inserted deeply into the extended hydrophobic pocket, interacting with the aryl residues of 1HS6. 3) To optimize inhibitor-enzyme interactions, we modified the carboxyl group to the methyl ester. The methyl ester is inserted into the hydrophobic pocket formed by the side chains of Arg563, Lys565, Gly268 and Tyr267. This may improve the hydrophile-lipophile balance of AHPA-Val and enhance its potency. Therefore, a methyl ester moiety is suggested at the carboxyl terminal, aiding the interaction with the residues of 1HS6. In addition, modulation of the non-polar substituent is presumed to enhance the inhibitory potency, and the affinity of inhibitors with APN was strongly dependent on the nature of the side chain.
Figure 6 Stereoview of comparison between the binding modes of bestatin and AHPA-Val. The principal interactions between the inhibitor and active site are shown.
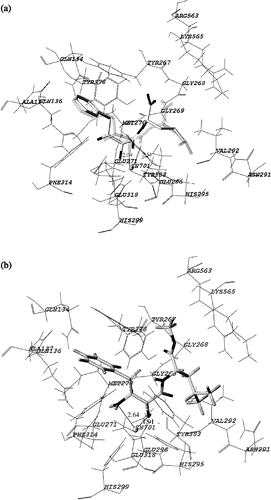
Previous experiments showed that endogenous opioid peptides, e.g., Met-enkephalin and dynophin, were mainly degraded to des-Tyr-peptide (e.g., des-Tyr-Met-enkephalin and des- Tyr-dynophin) in the absence of APNIs. However, Tyr-Gly bond in Met-enkephalin or dynophin remained in the presence of APNIs [Citation6,Citation7]. As our interest was to investigate the effect of APNIs on the hydrolysis of Tyr-Gly bond, we employed dipeptide Tyr-Gly instead of Met-enkephalin in the first experiment to evaluate the potency of AHPA-Val.
Our results showed that Tyr-Gly is almost completely hydrolyzed with the membrane fraction prepared from striatum of guinea pig brain in the absence of APNIs (see ). However, in the presence of amastatin or APHA-Val, most of Tyr-Gly remained intact. According to Lineweaver-Burk plots analysis, it is suggested that amastatin showed a competitive type of enzyme inhibition (see ), being in agreement with previous report [Citation21]. AHPA-Val also showed a competitive inhibition (see B). The Ki values against Tyr-Gly indicated that the potency of AHPA-Val was slightly lower than that of amastatin, being consistent with the result of the isolated ileum preparation. Moreover, it was indicated that the potency of amastatin was higher than that of AHPA-Val at doses ranging from 0–10 μM. However, with a high concentration (100 μM–1 mM) of APNIs, the effects of AHPA-Val were more potent than that of amastatin. The hydrolysis of Tyr-Gly was completely prevented by high concentrations of AHPA-Val, but a part of Tyr-Gly was still hydrolyzed in the presence of high concentrations of amastatin. These results suggest that AHPA-Val exhibited a significantly higher potency than amastatin when the concentrations of inhibitors increased up to 100 μM. Further studies are needed to elucidate this interesting result.
We demonstrated that the Met-enkephalin-induced twitch inhibition in guinea pig ileum was increased by APNIs, indicating significant suppression of Met-enkephalin degradation (see ). The pA2 is an analytical method to measure the potency of an antagonist at its receptor [Citation17]. In the present experiment we modified the pA2 analysis to a pA1/2 analysis for the evaluation of the potency of APNIs. The pA1/2 analysis was employed to estimate the inhibitory effect of amastatin and AHPA-Val on the degradation of Met-enkephalin (see ). From pA1/2 values of amastatin and AHPA-Val, we determined that the magnitude of the enhancing effect of 83.17 nM of AHPA-Val was equal to that of 16.32 nM of amastatin, indicating that the potency of AHPA-Val is 4.9 times lower than that of amastatin.
In this research, we established two independent systems to investigate the potency of the new APNI, AHPA-Val: one employing Tyr-Gly incubation with the membrane fraction prepared from striatum of guinea pig brain and the other using Met-enkephalin-induced twitch inhibition in guinea pig ileum. Additionally, pA1/2 analysis and Lineweaver-Burk pot analysis were performed to evaluate the potency of AHPA-Val, and coincident results were observed. These results indicate that both of two assay systems are useful to evaluate the potency of novel APNIs.
Conclusions
The inhibition of APN activity is proposed as a useful tool for enhancing the therapeutic actions, such as analgesia and antitumor, of endogenous opioid peptides. In this study, we employed two systems to investigate the activity of a novel compound, AHPA-Val, and we found that it significantly potentiated the effect of Met-enkephalin, indicating that AHPA-Val has good biological activity. Moreover, we established two useful systems for the in vitro evaluation of the APN inhibitor, which will be helpful for us to evaluate a highly potent APNI. Further studies will be needed to investigate the relationship between structure and activity of newly synthesized APNIs. In the future, we may design new compounds, and then evaluate the efficacy of APNIs using the two systems which that established in this study.
Acknowledgements
This study was supported by a Medical research grant from Wakayama Foundation for the Promotion of Medicine. The authors thank Dr. James H. Woods and Dr. Gail Winger (Department of Pharmacology, the University of Michigan) for their advice concerning this manuscript.
References
- Churchill L, Bausback HH, Gerritsen ME, Ward PE. Metabolism of opioid peptide by cerebral microvascular aminopeptidase. M Biochem Biophys Acta 1987; 923: 35–41
- Erickson JA, Jalie M, Robertson DH, Lewis RA, Vieh M. Lessons in molecular recognition: The effects of ligand and protein flexibility on molecular docking accuracy. J Med Chem 2004; 47: 45–55
- Fournie'-Zaluski MC, Chaillet P, Bouboutou R, Coulaud A, Cherot P, Waksman G, Costentin J, Roques BP. Analgesic effects of kelatorphan, a new highly potent inhibitor of multiple enkephalin degrading enzymes. Eur J Pharmacol 1984; 102: 525–528
- Glavas-Obrovac L, Jkas A, Marczi S, Horvat S. The influence of cell growth media on the stability and antitumour activity of methionine enkephalin. J Pept Sci 2005; 11: 506–511
- Glowinski J, Lyersen LL. Regional studies of catecholamines in the rat brain-1: The disposition of [3H]norepinephrine, [3H]dopamine and [3H]dopa in various regions of the brain. 1. J Neurochem 1966; 13: 655–669
- Hiranuma T, Iwao K, Kitamura K, Matsumiya T, Oka T. Almost complete protection from [Met5]-enkephalin-Arg6-Gly7-Leu8 (Met-enk-RGL) hydrolysis in membrane preparations by the combination of amastatin, captopril and phosphoramidon. J Pharmacol Exp Ther 1997; 281: 769–774
- Hiranuma T, Kitamura K, Taniguchi T, Kanai M, Arai Y, Iwao K, Oka T. Protection against dynorphin-(1–8) hydrolysis in membrane preparations by the combination of amastatin, captopril and phosphoramidon. J Pharmacol Exp Ther 1998; 286: 863–869
- Jaba IM, Vasincu D, Manolidis G, Haulica I, Mungiu OC. Experimental data regarding the implications of certain minimum structure enkephalin-like peptides in nociceptive processing. Rom J Physiol 2004; 41: 119–126
- Jagloski JR, Zagon IS, Stack BC, Jr, Verderame MF, Leure-duPree AE, Manning JD, Mc Laughlin P. Opioid growth factor enhances tumor growth inhibition and increases the survival of paclitaxel-treated mice with squamous cell carcinoma of the head and neck. Cancer Chemother Pharmacol 2005; 56: 97–104
- Jankovic BD, Maric D. Enkephalins modulate in vivo immune reactions through delta- and mu-opiod receptors. Ann N Y Acad Sci 1988; 540: 691–693
- Kishioka S, Miyamoto Y, Nishida S, Fukunaga Y, Yamamoto H. Effects of a mixture of peptidase inhibitors (amastatin, captopril and phosphoramidone) on Met-enkephalin-, β-endorphin-, dynorphin-(1–13)- and electroacupuncture-induced antinociception in rats. Jpn J Pharmacol 1994; 66: 337–345
- Marotti T, Balog T, Munic V, Sobocanec S, Abramic M. The link between met- enkephalin-induced down-regulation of APN activity and the release of superoxide anion. Neuropeptides 2000; 34: 121–128
- Scornik OA, Botbol V. Bestatin as an experimental tool in mammals. Curr Drug Metab 2001; 2: 67–85
- Oka T, Negishi K, Suda M, Sawa A, Fujino M, Wakimasu M. Evidence that dynophin- (1–13) acts as an agonist on opioid κ-receptors. Eur J Pharmacol 1982; 77: 137–141
- Rarey M, Kramer B, Lenqauer T, Klebe G. A fast flexible docking method using an incremental construction algorithm. J Mol Biol 1996; 261: 470–489
- Rawlings ND, Barret AJ. Evolutionary families of metallopeptidases. Meth Enzymol. 1995; 248: 183–228
- Tallarida RJ, Murray RB. Manual of pharmacologic calculations with computer programs2nd edn. Springer-Verlag, New York 1987; 53–56
- Taniguchi T, Fan XT, Kitamura K, Oka T. Effects of peptidase inhibitors on the enkephalin-induced anti-nociception in rats. Jpn J Pharmacol 1998; 78: 487–492
- Thunnissen MM, Nordlund P, Haeggstrom JZ. Crystal structure of human leukotriene A(4) hydrolase, a bifunctional enzyme in inflammation. Nat Struct Biol 2001; 8: 131–135
- Turer AJ, Matsas R, Kenny AJ. Are there neuropeptide-specific peptidases?. Biochem Pharmacol 1985; 34: 1347–1356
- Umezawa H. Low-molecular-weight enzyme inhibitors of microbial origin. Annu Rev Microbiol 1982; 36: 75
- Xu J, Xin S, Li H, Liu L, Xia W, Li P, Liu X, Li G. Involvement of caspase-3 pathway in anti-apoptotic action of methionine enkephalin on CEMx174 cells in prolonged infection with simian immunodeficiency virus in vitro. Cell Bio Int 2006; 30: 114–121