Abstract
The novel linear polymer of a macrocyclic polyamine copper (II) complex, which has many cyclen groups linked by epichlorohydrin, has been synthesized as a DNA cleavage agent. The structure of the polymer 3 was identified by 1HNMR and IR and its molecular weight was measured by GPC. The result of agarose gel electrophoresis assay showed that Cu-(II) complex 4 could act as a powerful catalyst for the cleavage of plasmid DNA under physiological conditions.
Introduction
The remarkable stability of the phosphodiester bond in DNA is one of the essential needs for the survival and maintenance of life, due to the repulsion between the negatively charged phosphodiester backbone and potential nucleophiles [Citation1]. The half-life for spontaneous hydrolysis of the phosphodiester has been estimated as about 1011 years at pH 7 and 30°C [Citation2]. Some natural enzymes, such as restrition endonucleases and topoisomerases, can catalyze phosphoryl cleavage efficiently under physiological conditions, but the enzymatic reaction is difficult to be performed in the laboratory [Citation3]. Thus, great efforts have been made to expand artificial enzyme that work on DNA with similar catalytic mode [Citation4]. Chemical nucleases could recognize a specific DNA sequence and enhance the hydrolysis of the phosphate diester bond, which would rapidly be an invaluable research tool of biology, bioorganic chemistry and molecular biology Citation5,Citation6,Citation7. Most of the known chemical nucleases for DNA hydrolysis are metal complexes Citation8,Citation9,Citation10,Citation11,Citation12.
Many complexes have been designed and studied as artificial enzyme models. In particular, 1, 4, 7, 10-tetraazacyclododecane (cyclen) has a special structure and can coordinate with most metal cations (transition metal ions and lanthanide ions) Citation13,Citation14,Citation15,Citation16. At present, many cyclen metal cations complexes have been used in DNA recognition and cleavage Citation15, Citation17,Citation18,Citation19,Citation20. Our group also reported several new metal complexes of cyclen derivatives, which are used as efficient chemical enzyme to enhance the cleavge ability of DNA Citation21,Citation22,Citation23,Citation24,Citation25,Citation26. However, almost all of those reported complexes of cyclen are mononuclear or dinuclear complexes. Yet to now, to best of our knowledge, a few complex of polymer of cyclen was reported. In most cases, synergistic effects exist between the metal centers when complex as chemical enzyme interact with DNA [Citation27]. Hence, it is anticipated that complexes of polymer of cyclen is an efficient artifical enzyme to cleavage DNA under physical conditions. On the other hand, copper (II) complex which can accelerate hydrolysis cleavage DNA is one of the most promising metals for artificial nucleases [Citation26]. Moreover, more than twelve enzymes depend on copper for their activity. Therefore, the synthesis and activity studies with DNA of copper complexes have attracted more attention from different perspectives Citation28,Citation29.
In this report, we described the synthesis and characteriazation of a novel linear polymer of cyclen copper (II) complex, which was linked by epichlorohydrin, and its applications on DNA cleavage was also studied. The result showed that it can catalyze the cleavage of supercoiled DNA (pUC 19, plasimid DNA) under physiological conditions to produce selectively nicked DNA (Form II).
Experimental
General
The 1H NMR spectra were measured on a Varian INOVA-400 spectrometer and the δ scales in ppm were referenced to residual solvent peaks or internal tetramethylsilane (TMS). IR spectra were recorded on a Shimadzu FTIR-4200 spectrometer as KBr pellets or thin films on KBr plates. Inductively Coupled Plasma Atomic Emission Spectrometers (ICP-AES) was conducted on IRIS Advantage. Gel permeation chromatogram (GPC) was using a waters instrument incorporating Shodex columns (OHPAK KB-803) and polyglycol standard was used for calibration. Electrophoresis apparatus was a biomeans stack II-electrophoresis system, PPSV-010. Bands were visualized by UV light and photographed using a gel documentation system by the estimation of the intensity of the DNA bands, recorded on an Olympus Grab-IT 2.0 annotating image computer system. Cyclen were prepared according to the literature [Citation31]. All other chemicals and reagents were obtained commercially and were used without further purification.
Synthesis of polymer 2
1, 7-bis (diethpxyphosphoryl)-1, 4, 7, 10-tetraazacyclodocane (130 mg, 0.293 mmoL) were dissolved in C2H5OH, then H2O and epichlorohydrins (27.1 mg, 0.293 mmoL) were added. Under the protection of N2, the reaction was stirring at 80°C for 24 h. After adding NaOH (26.4 mg, 0.659 mmoL), the system was stirred at 80°C for another 24 h. The solvent was removed and the alkaline solution was extracted with hot CHCl3. The organic layer was dried over anhydrous Na2SO4, and then the solvent was evaporated to 5 mL. After dropping petrol ether to the solution, polymer 2 was obtained. IR (CH2Cl2, cm− 1): 3329, 2968, 2928, 2843, 1458, 1373, 1249, 1216, 1159, 1090, 1045, 755. 1H NMR (400 MHz, CDCl3): δ (ppm) = 4.16–3.84 (m, 8H, OCH2CH3), 3.90–3.84 [m, 1H, CH(OH)], 3.65–3.45 [m, 4H, CH(OH)(CH2)2], 3.40–3.20 [m, 8H, N(CH2)2PO(OCH2CH3)2], 2.95–2.70 [m, 8H, N(CH2)2], 2.00 (bres, 1H, OH), 1.43–1.30 (m, 12H, OCH2CH3).
Synthesis of polymer 3
10 mL C2H5OH and 10 mL HCl (37%) were added to the complex 2 (330 mg) and the system was stirring at 50°C for 24 h under the protection of N2. After the solvent had been removed, the pH was adjusted to pH 12 with 5 N NaOH aqueous solution. The alkaline solution was extracted with hot CHCl3. The organic layer was dried over anhydrous Na2SO4, and then the solvent was evaporated to 5 mL. After dropping petrol ether to the solution, polymer 3 was obtained. The molecular weight of polymer 3 was measured by GPC (Mw = 1077). IR (CH2Cl2, cm− 1): 3395, 2924, 2825, 1342, 1161, 1091, 1032, 713. 1H NMR (400 MHz, DMSO): δ (ppm) = 5.7 (m, 1H, OH), 4.10–3.86 [m, 2H, NH(CH2)2], 3.65–3.45 [m, 4H, CH(OH)(CH2)], 3.40–3.20 [m, 8H, N(CH2)2], 2.95–2.70 [m, 8H, N(CH2)2]. Elemental analysis (HCl salt): C, 37.27; N, 15.20; H, 7.30.
Synthesis of complex 4
To a solution of polymer 3 (12 mg) and C2H5OH (2 mL), a solution of CuCl2·H2O (15 mg) and C2H5OH (1.0 mL) were added slowly. After the reaction mixture stirring overnight at room temperature, a pink blue solid (complex 4) was obtained after filtrating. IR (KBr, cm− 1): 3438, 2961, 2875, 1458, 1229, 1079, 1025, 805. ICP-AES [Cu2 + ]: 3.200 μg/mL. Found: 2.975 μg/mL.
Plasmid DNA cleavage
Plasmid DNA (pUC 19) cleavage activity of complex 4 was monitored by using agarose gel electrophoresis. In a typical experiment, supercoiled pUC 19 DNA (5 μL, 0.025 μg/μL) in Tris-HCl buffer (100 mM) was treated with different concentration of complex 4, followed by dilution with the Tris-HCl buffer to a total volume of 17.5 μL. The samples were then incubated at 37°C for 4 – 72 h, and loaded on a 1% agarose gel containing 1.0 mg/mL ethidium bromide. Electrophoresis was carried out at 40 V for 30 min in TAE buffer. Bands were visualized by UV light and photographed followed by the estimation of the intensity of the DNA bands using a Gel Documentation System.
Result and discussion
Preparation of polymer of cyclen Cu (II) complex
Scheme shows the synthetic route for the novel linear polymer of cyclen Cu (II) complex (complex 4) studied in this work. The 1, 7-bis (diethpxyphosphoryl)-1, 4, 7, 10-tetraazacyclodocane (1) [Citation30] has been found to be an effective protecting group for the synthesis of 1, 7-disubstituent cyclen. Hence, 1 was used as the intermediate, which was linked with epichlorohydrin to produce the polymer 2. The Dep protective groups were cleaved completely from polymer framework by dense hydrochloric acid, which was indicated by 1HNMR. Polymer 3 was allowed to react with CuCl2·H2O in EtOH overnight to give complex 4. ICP-AES indicated that almost all cyclen were coordinated with copper (II). However, the polymer 3 is a liquid and bibulous substance so that it is difficult to analyze its element content at room temperature. Therefore, we convert polymer 3 to its HCl salt to analyze its element content.
Cleavage of plasmid DNA
The activities of complex 4 with pUC 19 supercoiled DNA were studied. The cleavage of the supercoiled plasmid DNA (Form I) was processed to produce selectively open-circular (Form II). The amounts of strand scission were assessed by agarose gel electrophoresis. Typical gels showed that the highest activity was observed at pH 7.4 and the results for these experiments were shown in .
Figure 1. Effect of pH on the cleavage reaction of pUC 19 DNA (7 μg/mL) with complex 4 (1 mg/mL) in a Tris-HCl buffer (100 mM) at 37°C for 72 h. (A) Agarose gel electrophoresis diagram: lane 1, DNA control, pH 7.4; lane 2, pH 7.4; lane 3, DNA control, pH 7.0; lane 4, pH 7.0; lane 5, DNA control, pH 6.6; lane 6, pH 6.6; lane 7, DNA control, pH 6.2; lane 8, pH 6.2; lane 9, DNA control, pH 5.8; lane 10, pH 5.8. (B) quantitation of % plasmid relaxation relative to plasmid DNA per lane.
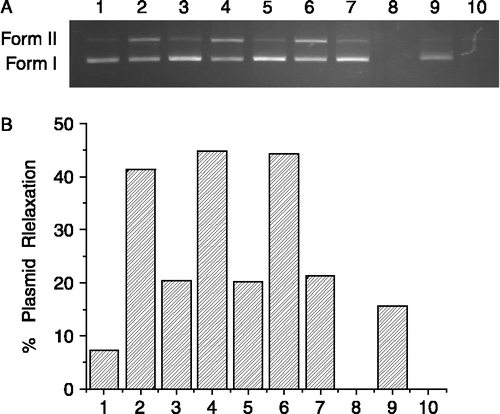
Figure 2. Effect of time on the cleavage reaction of pUC 19 DNA (7 μg/mL) with complex 4 (1 mg/mL) in a Tris-HCl buffer (100 mM, pH 7.4) at 37°C. (A) Agarose gel electrophoresis diagram: lane 1, DNA control, 0 h; lane 2, DNA control, 72 h; lane 3, 4 h; lane 4, 8 h; lane 5, 24 h; lane 6, 72 h. (B) quantitation of % plasmid relaxation relative to plasmid DNA per lane.
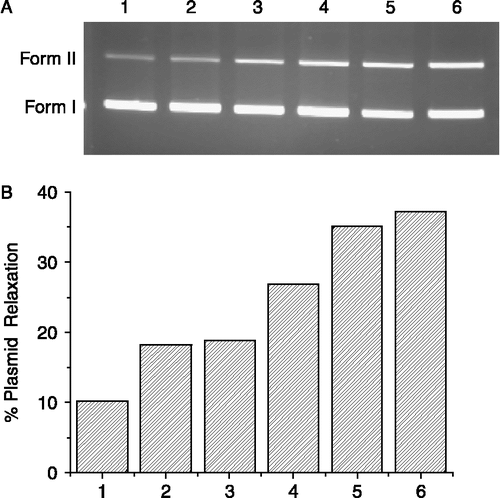
Figure 3. Effect of concentration of complex 4 on the cleavage reaction of pUC 19 DNA (7 μg/mL) with complex 4 in a Tris-HCl buffer (100 mM, pH 7.4) at 37°C. (A) Agarose gel electrophoresis diagram: lane 1, DNA control, 0 h; lane 2, DNA control, 72 h; lane 3, 0.2 mg/mL; lane 4, 0.4 mg/mL; lane 5, 0.6 mg/mL; lane 6, 0.8 mg/mL; lane 7, 1.0 mg/mL. (B) quantitation of % plasmid relaxation relative to plasmid DNA per lane.
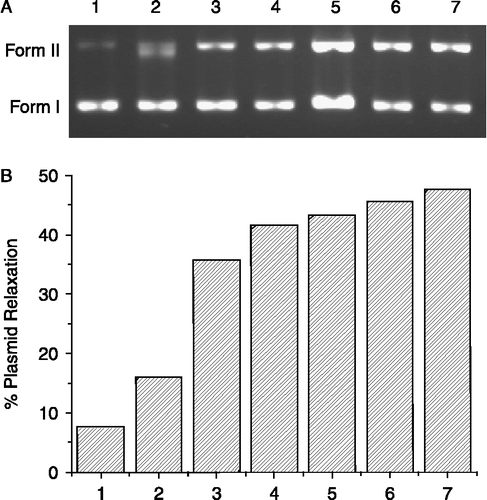
Firstly, we compared the DNA cleavage abilities of complex 4 under different pH buffer. As shown in , with the pH decrease from 7.4 to 6.6, complex 4 has almost the same catalytic effect for DNA cleavage, and no nicked DNA (From II) were found when the buffer pH = 6.2 and 5.8. On the other hand, the quantity of nicked DNA increased from 7.3 to 20% when the pH of buffer reduced from 7.4 to 6.6. From the above analysis, eliminating the nicked DNA by buffer, complex 4 has the best catalytic ability for DNA cleavage at pH = 7.4. So, complex 4 has the best catalytic effect under physical condition.
and showed the effect of cleavage time of complex 4. The decrease of supercoiled Form I was accompanied the appearance of opening circular Form II. It was found that the longer time reacted, the more product of DNA cleavage was detected. When the reaction time reached 72 h, 37.3% nicked DNA (Form II) was observed ().
The cleavage of DNA by different concentration of complex 4 was also studied (). The amount of nicked DNA (Form II) observed in agarose gel electrophoresis diagram increased with increase of concentration of complex 4 under the reaction system (). The concentration of complex 4 increased in the order of 0.2, 0.4, 0.6 and 0.8 mg/mL, resulting in 37.9, 47.1, 47.3 and 50% nicked DNA, respectively (). When the concentration of complex 4 reach to 1.0 mg/mL, there was more DNA cleavage (52.3% nicked DNA).
In conclusions, we first designed and synthesized a novel linear polymer of cyclen copper (II) complex. The agarose gel electrophoresis assay indicated that complex 4 as an artifical enzyme can quicken up the plasmid DNA cleavage to nicked DNA (Form II) under physical conditions.
Acknowledgements
We thank the financial support from the National Natural Science Foundation of China (Grant Nos. 20572075, 20471038 and 20732004), Program for New Century Excellent Talents in University, Specialized Research Fund for the Doctoral Program of Higher Education and Scientific Fund of Sichuan Province for Outstanding Young scientist.
Declaration of interest: The authors report no conflicts of interest. The authors alone are responsible for the content and writing of the paper.
References
- FH Westheimer. (1987). Why nature chose phosphates. Science 235:1173–1178.
- NH Williams, B Takasaki, and Wall M Chin. (1999). Structure and Nuclease Activity of Simple Dinuclear Metal Complexes: Quantitative Dissection of the Role of Metal Ions. J Acc Chem Res 32:485–493.
- EL Hegg, and JN Burstyn. (1998). Toward the development of metal-based synthetic nucleases and peptidases: A rationale and progress report in applying the principles of coordination chemistry. Coord Chem Rev 173:133–165.
- A Radzicka, and R Wolfenden. (1995). A proficient enzyme. Science 267:90–93.
- M Komiyama, N Takeda, and H Shigekawa. (1999). Hydrolysis of DNA and RNA by lanthanide ions: Mechanistic studies leading to new applications. Chem Commun 1443–1451.
- JR Morrow, LA Buttrey, VM Shelton, and KA Berback. (1992). Efficient catalytic cleavage of RNA by lanthanide(III) macrocyclic complexes: Toward synthetic nucleases for in vivo applications. J Am Chem Soc 114:1903–1905.
- CQ Pan, and RA Lazarus. (1997). Engineering Hyperactive Variants of Human Deoxyribonuclease I by Altering Its Functional Mechanism. Biochemistry 36:6624–6632.
- BK Takasaki, and J Chin. (1994). Cleavage of the Phosphate Diester Backbone of DNA with Cerium(III) and Molecular Oxygen. J Am Chem Soc 116:1121–1122.
- MP Fitzsimons, and JK Barton. (1997). Design of a Synthetic Nuclease: DNA Hydrolysis by a Zinc-Binding Peptide Tethered to a Rhodium Intercalator. J Am Chem Soc 119:3379–3380.
- A Roigk, R Hettich, and HJ Schneider. (1998). Unusual Catalyst Concentration Effects in the Hydrolysis of Phenyl Phosphate Esters and of DNA: A Systematic Investigation of the Lanthanide Series. Inorg Chem 37:751–756.
- A Sreedhara, JD Freed, and JA Cowan. (2000). Efficient Inorganic Deoxyribonucleases. Greater than 50-Million-Fold Rate Enhancement in Enzyme-Like DNA Cleavage. J Am Chem Soc 122:8814–8824.
- ME Branum, AK Tipton, S Zhu, and L QueJr. (2001). Double-Strand Hydrolysis of Plasmid DNA by Dicerium Complexes at 37°C. J Am Chem Soc 123:1898–1904.
- V Alexander. (1995). Design and Synthesis of Macrocyclic Ligands and Their Complexes of Lanthanides and Actinides. Chem Rev 95:273–342.
- P Caravan, JJ Ellison, TJ McMurry, and RB Lauffer. (1999). Gadolinium(III) Chelates as MRI Contrast Agents: Structure, Dynamics, and Applications. Chem Rev 99:2293–2352.
- S Aoki, and E Kimura. (2004). Zinc-Nucleic Acid Interaction. Chem Rev 104:769–788.
- I Lukeš, J Kotek, P Vojtišek, and P Hermann. (2001). Complexes of tetraazacycles bearing methylphosphinic/phosphonic acid pendant arms with copper(II), zinc(II) and lanthanides(III). A comparison with their acetic acid analogues. Coord Chem Rev 216–217:287–312.
- N Aki, and EU Akkaya. (2004). Bifunctional catalysis of ester hydrolysis: Novel hydrolytic enzyme models based on xanthene framework. J Mol Catal A: Chem 219:227–232.
- S Aoki, D Kagata, M Shiro, K Takeda, and E Kimura. (2004). Metal Chelation-Controlled Twisted Intramolecular Charge Transfer and Its Application to Fluorescent Sensing of Metal Ions and Anions. J Am Chem Soc 126:13377–13390.
- S Aoki, K Iwaida, N Hanamoto, M Shiro, and E Kimura. (2002). Guanidine Is a Zn2+-Binding Ligand at Neutral pH in Aqueous Solution. J Am Chem Soc 124:5256–5257.
- E Kimura, H Kitamura, K Ohtani, and T Koike. (2000). Elaboration of Selective and Efficient Recognition of Thymine Base in Dinucleotides (TpT, ApT, CpT, and GpT), Single-Stranded d(GTGACGCC), and Double-Stranded d(CGCTAGCG)2 by Zn2+-Acridinylcyclen (Acridinylcyclen = (9-Acridinyl)methyl-1,4,7,10-tetraazacyclododecane). J Am Chem Soc 122:4668–4677.
- QX Xiang, J Zhang, PY Liu, CQ Xia, ZY Zhou, RG Xie, and XQ Yu. (2005). Dinuclear macrocyclic polyamine zinc(II) complexes: Syntheses, characterization and their interaction with plasmid DNA. J Inorg Biochem 99:1661–1669.
- SY Chen, Y Huang, GL Zhang, H Cheng, CQ Xia, LJ Ma, H Yu, and XQ Yu. (2005). Synthesis of a cyclen-functionalized-amino acid and its incorporation into peptide sequence. Synthesis 6:888.
- LJ Ma, GL Zhang, SY Chen, B Wu, JS You, CQ Xia, and XQ Yu. (2005). The first synthesis of chiral PNA monomer-cyclen conjugates. J Peptide Sci 11:812.
- W Peng, PY Liu, N Jiang, HH Lin, GL Zhang, and XQ Yu. (2005). Dinuclear macrocyclic polyamine zinc (II) complexes: Synthesis, characterization and their interaction with plasmid DNA. Bioorg Chem 33:374.
- QL Li, J Huang, Q Wang, N Jiang, CQ Xia, HH Lin, J Wu, and XQ Yu. (2006). Monometallic complexes of 1, 4, 7, 10-tetraazacyclododecane containing an imidazolium side: Synthesis, characterization, and their interaction with plasmid DNA. Bioorg Med Chem 14:4151.
- XY Wang, J Zhang, K Li, N Jiang, SY Chen, HH Lin, Y Huang, LJ Ma, and XQ Yu. (2006). Synthesis and DNA cleavage activities of mononuclear macrocyclic polyamine zinc(II), copper(II), cobalt (II) complexes which linked with uracil. Bioorg Med Chem 14:6745–6751.
- N Saki, and EU Akkaya. (2004). Bifunctional catalysis of ester hydrolysis: Novel hydrolytic enzyme models based on xanthene framework. J Mol Catal A: Chem 219:227–232.
- YM Zhao, JH Zhu, WJ He, Z Yang, YG Zhu, YZ Li, JF Zhang, and ZJ Guo. (2006). Oxidative DNA Cleavage Promoted by Multinuclear Copper Complexes: Activity Dependence on the Complex Structure. J Chem Eur 12:6621–6629.
- LZ Li, C Zhao, T Xu, HW Ji, YH Yu, GQ Guo, and H Chao. (2005). Synthesis, crystal structure and nuclease activity of a Schiff base copper(II) complex. J Inorg Biochem 99:1076–1082.
- D Arnaud, J Vincent, QX Peng, and FD Jean. (1994). Regioselective synthesis of 1,7-diprotected 1,4,7,10-tetraazacyclododecane and preparation of a dialcohol dicarboxylic macrocyclic ligand. Tetrahedron Lett 35:3707–3710.
- PS Athey, and GE Kiefer. (2002). A New, Facile Synthesis of 1,4,7,10-Tetraazacyclododecane: Cyclen. J Org Chem 67:4081–4085.