Abstract
Various substituted 1-arylmethyl-2,3-dioxo-2,3-dihydroindole thiosemicarbazones 3a-h, 1-benzyl-2,3-dioxo-2,3-dihydroindole N4-aryl thiosemicarbazones 4a-i and 1-benzyl-2,3-dioxy-2,3-dihydroindole N4-cyclohexylthiocarbazone 5 were synthesized. All of these compounds were evaluated against human Molt 4/C8 and CEM T-lymphocytes as well as murine L1210 leukemia cells. Nearly 40% of these compounds possess low micromolar IC50 values and some are either more potent than, or equipotent with, melphalan. Various correlations between the structures of these compounds and cytotoxic potencies were obtained which included the use of QSAR and molecular modeling techniques. Representative compounds displayed anticonvulsant properties in rats and were well tolerated by these animals. The encouraging biodata noted affords adequate rationale for outlining guidelines for further development of these molecular scaffolds.
Introduction
An ongoing interest in these laboratories are thiosemicarbazones which display antineoplastic properties [Citation1,Citation2] and also have the capacity to prevent convulsions Citation1, Citation2, Citation3, Citation4. In particular, various compounds having the general formula 1 () display antineoplastic [Citation5] and anticonvulsant [Citation6] properties; both of these activities are retained in the cyclic analog 2 [Citation2]. A further reason for using 2 as the lead molecule is that while various acyclic thiosemicarbazones are lethal to mice at doses of 25-100 mg/kg [Citation1], administration of 300 mg/kg of 2 was tolerated by these animals [Citation2].
The objectives of the present investigation were to develop analogs of 2 principally as candidate cytotoxins and also for an assessment of their anticonvulsant properties. The thiosemicarbazone 2 displays cytotoxicity to human Molt 4/C8 and CEM T-lymphocytes as well as murine L1210 cells but the IC50 values are low, viz 205, 83 and 76 μM, respectively [Citation2]. These potencies may reflect the formation of only weak interactions between the ligand and a binding site. Hence the systematic enlargement of the structure of 2 may allow the additional groups to bind to a receptor thereby reinforcing the interactions of the scaffold 2 with biomacromolecules.
A review of the literature revealed that the insertion of a nitrogen atom into the acyclic ring of 2 and conversion of one of the methylene group into a carbonyl function gave rise to a series of 2,3-dioxo-2,3-dihydroindoles which display cytotoxic properties [Citation7]. These additional polar atoms may enhance hydrogen bonding at a receptor. In addition, bearing in mind the importance of hydrophobic forces in eliciting a biological response, an arylalkyl group was attached to the heterocyclic nitrogen atom. From this basic design, various substituents were mounted on this scaffold leading to series 3-5. The decision to prepare this cluster of compounds was reinforced by the report that the related semicarbazones of 2,3-dioxy-2,3-dihydroindoles demonstrate anticonvulsant properties [Citation8].
In summary, the decision was made to prepare series 3-5 principally for cytotoxic evaluation with the aim of discovering compounds with significantly increased potencies compared to 2. If this objective was achieved, efforts would be made to provide guidelines for subsequent amplification of the project. In addition, the assessment of whether representative compounds have anticonvulsant properties was planned.
Materials and methods
Melting points are uncorrected and recorded in Celsius degrees. Rf values were obtained using silica gel thin layer chromatography plates and a solvent system of chloroform:methanol (1:9). Ultraviolet spectra of 3a, 4a,b,e,g-i and 5 in methanol were obtained on a Jasco V-630 instrument. The infrared spectra of all compounds were determined by a diffuse reflectance technique using potassium bromide powder on a Jasco 460+ FTIR machine. 1H NMR spectra (300, 400 or 500 MHz) of all compounds except for 4e were generated in dimethylsulfoxide –d6 on Bruker Ultraspec spectrophotometers. Electron impact mass spectra were obtained on 3a, 4a,b,e,g-i and 5 using a Perkin Elmer instrument.
Syntheses of 3a-h
The synthesis of the intermediate 2,3-dioxy-2,3-dihydroindoles was accomplished using a literature methodology [Citation9] and a previously reported procedure was used to convert these compounds to the corresponding 1-arylmethyl-2,3-dioxy-2,3-dihydroindoles [Citation10].
A mixture of the 1-arylmethyl-2,3-dioxy-2,3-dihydroindole (0.005 mol), thiosemicarbazide (0.005 mol), acetic acid (0.5-1.0 mL) and ethanol (100 mL) was heated under reflux until the reaction was completed (∼ 4 h). Approximately half of the ethanol was removed in vacuo and the solution was left overnight at room temperature. The solid which precipitated was collected, washed with cold ethanol and recrystallized from ethanol:chloroform (9:1) to give the following compounds (melting points and percentage yields in parentheses), namely 3a (260-263, 85), 3b (233-238, 69), 3c (256-260, 74), 3d (238-239, 72), 3e (251-253, 70), 3f (240-242, 75), 3g (241-243, 68), and 3h (258-260, 65). The physicochemical data generated for a representative compound 3e are as follows: Rf: 0.61, FTIR (KBr): 3411 (NH2), 3237 (NH), 3146, 3045-3015 (CH), 2984-2929 (CH), 1698 (CO), 1596 (CN) cm− 1. 1H NMR: δ: 12.21 (1H, s, NH), 9.12 (s, 1H, NH), 8.82 (s, 1H, Ar), 7.90 (m, 1H, Ar), 7.51-7.48 (m, 1H, Ar), 7.22 (2H, d, Ar, J = 8.0 Hz), 7.10 (d, 2H, Ar, J = 8.0 Hz), 6.90 (d, 2H, Ar, J = 8.0 Hz), 4.87 (s, 2H, CH2), 2.21 (s, 3H, CH3); EIMS: 403.55 (100).
Synthesis of 4a-c and 5
The N4-arylthiosemicarbazides and N-cyclohexylthiosemicarbazide required in the preparation of 4a-i and 5, respectively, were prepared by a literature methodology [Citation11,Citation12]. The appropriate 1-benzyl-2,3-dioxy-2,3-dihydroindole reacted with a thiosemicarbazide using the methodology for preparing 3a-h. The melting points and percentage yields of the compounds are as follows; 4a (160-162, 83), 4b (208-211, 80), 4c (215-219, 70), 4d (144-148, 73), 4e (204-207, 72), 4f (145-147, 68), 4g (185-188, 65), 4h (200-202, 74), 4i (228-230, 70) and 5 (184-195, 90). Some physical characteristics of two representative compounds are as follows; 4a: Rf 0.69; UV (λ max) 254, 368 nm; FTIR (KBr): 3321, 3225 (NH), 2915 (CH), 1686 (CO), 1541 (CN) cm− 1; 1H NMR: δ: 13.01 (s, 1H, NH), 9.52 (s, 1H, NH), 7.75 (d, 2H, Ar, J = 8.0 Hz), 7.65 (d, 1H, Ar, J = 7.0 Hz), 7.43-7.26 (t, m, 10H, Ar, J = 15.6 Hz), 7.13-7.09 (m, 1H, Ar), 6.83 (d, 1H, Ar, J = 8.0 Hz), 4.95 (s, 2H, N-CH2); EIMS: 387.52 (100). 5: Rf: 0.53; UV (λ max): 253, 257, 371 nm; FTIR (KBr): 3352 (NH), 3030 (CH), 2926-2848 (CH), 1691 (CO), 1571 (CN) cm− 1; 1H NMR: δ: 12.36 (s, 1H, NH), 8.83 (d, 1H, NH, J = 8.2 Hz), 7.72 (d, 1H, Ar, J = 7.3 Hz), 7.31-7.20 (m, 6H, Ar), 7.07 (t, 1H, Ar, J = 14.7 Hz), 6.97 (d, 1H, Ar, J = 7.8 Hz), 4.94 (s, 2H, N-CH2), 1.93-1.11 (m, 11H, cyclohexyl H); EIMS: 393.85 (100).
Molecular modeling
Models of 3a,4a,b,e,g-i and 5 were built using the Spartan programme [Citation13]. The conformers with the lowest averages were obtained with the CONFLEX programme and optimization took place by mechanics using augmented MM2 parameters.
Determination of log P values
The log P values of 3a-h, 4a-i, 5 and melphalan were generated using the JME molecular editor [Citation14].
Statistical analyses
The statistical evaluations were made using a commercial software package [Citation15].
Cytotoxicity assays
The methodology for undertaking the Molt 4/C8, CEM and L1210 assays has been published previously [Citation16]. In brief, varying concentrations of the compounds were incubated at 37°C for 72 h (Molt 4/C8 and CEM T-lymphocytes) or 48 h (L1210 cells).
Evaluation of 3a, 4a,b,e,g-i and 5 for anticonvulsant properties
Adult female Wistar rats (200-250 mg) were acclimatized to laboratory conditions for two or three days prior to experimentation and then fasted overnight. Each of the 2,3-dioxo-2,3-dihydroindole thiosemicarbazones and phenobarbitone were dissolved in saline or suspended in gum acacia solution (2% w/v) and administered orally to six animals. Maximal electroshock seizures were given to the rats by the application of a stimulus of 130 mA for 0.2 s from an electro-convulsometer (INCO, India). The time which elapsed between the administration of the electric current and the abolition of tonic seizures and clonic convulsions as well as recovery from stupor were recorded. The Protocol used for Animal Experimentation followed that of the Institutional Animal Ethics Committee of the KLES College of Pharmacy, Bangalore, Karnataka, India.
Results
The compounds in series 3-5 were prepared by the methodologies outlined in Scheme . 1H NMR spectroscopy indicated that the compounds exist as single isomers in solution. Molecular models of 3a and 4a revealed that the energies of the Z isomers are 394.2 and 556.0 KJ/mol, respectively, while the figures for the E isomers are 406.6 and 568.2 KJ/mol, respectively. Hence the assumption was made that the compounds adopted the Z conformation. Molecular models of 3a,4a,b,e,g-i and 5 were built and various structural parameters are presented in . The log P values of all of the compounds were computed and are portrayed in .
Scheme 1. The synthetic chemical route employed in obtaining the compounds in series3–5. The reagents used are as follows: i: CCl3CH(OH)2 / H2SO4 / Na2SO4; ii: R2-C6H4CH2Cl / K2CO3 /DMF; iii: H2NNHCSNH2; iv: R3C6H4NHCSNHNH2; v.: C6H11NHCSNHNH2. The nature of the R1, R2 and R3 substituents are presented in .
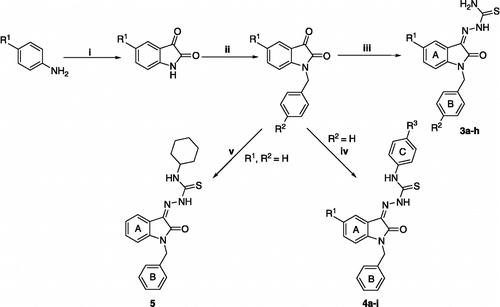
Table I. Evaluation of3a-h, 4a-i and 5 towards human Molt 4/C8 and CEM T-lymphocytes as well as murine L1210 leukemic cells and clogP values of these compounds.
All of the compounds were assayed against human Molt 4/C8 and CEM T-lymphocytes as well as murine L1210 cells. These data are summarized in . The anticonvulsant properties of 3a,4a,b,e,g-i and 5 were evaluated and the data are portrayed in .
Discussion
Comments will be made initially regarding the antineoplastic properties of 3a-h, 4a-i and 5 followed by some considerations of the potential of selected compounds as candidate anticonvulsants.
The choices of the R1 and R2 substituents in series 3 and 4 were made on the basis of attempting to discern the contributions of the various physicochemical properties of these groups to cytotoxic potencies. The substituents were principally chloro, bromo, methyl, fluoro and methoxy groups which are found in three of the four quadrants of a Craig plot [Citation17] thereby indicating a range of electronic and hydrophobic properties. In terms of the sizes of these groups, the molecular refractivity (MR) values vary from 0.92 (fluoro) to 8.88 (bromo) [Citation18].
The cytotoxic potential of the compounds in series 3-5 was evaluated against human Molt 4/C8 and CEM T-lymphocytes in order to ascertain their potencies towards human transformed cells. In addition, a number of clinically useful anticancer drugs display significant cytotoxic effects towards murine L1210 cells [Citation19]; this cell line therefore may detect promising lead compounds and was also employed in the bioassays. The results of these evaluations are presented in .
A review of the biodata in reveals that 37% of the compounds have IC50 values in the low micromolar range (1-10 μM). Hence various members of series 3 and 4 serve as lead molecules for further structural modifications. In particular the results in the Molt 4/C8 bioassay indicate that 3f and 4a,b,h have statistically significantly greater potencies than melphalan being 1.6, 1.9, 1.4 and 2.3 times, respectively, more potent than this established anticancer drug. Furthermore the following compounds are equipotent with melphalan, namely 4e,f (Molt 4/C8 screen), 3f and 4a,b,f,h (CEM test) and 3d,f,g (L1210 assay).
The biodata in reveal that in general Molt 4/C8 cells are the most sensitive to the compounds in series 3 and 4. The following observations were made pertaining to the influence of molecular modifications on cytotoxic potencies in this screen. The unsubstituted compound 3a has very low potency. However placement of halogens on ring A in series 3 led to 3b and 3c which caused a dramatic lowering of the IC50 values of 17-fold on average. No alterations in potencies were noted when a methyl group was introduced into ring B of 3b yielding 3d nor when either a methyl or fluoro substituent was placed in ring B of 3c giving rise to 3e and 3g, respectively. However retention of the methyl group in ring B and introducing a fluoro substituent in ring A led to the potent cytotoxin 3f. An interesting observation arose insofar as the structural isomer 3h is more than 250 times less potent than 3f which illustrates the remarkable influence of the aryl substituents on cytotoxic properties in series 3. Thus, in series 3 significant cytotoxic potencies are observed when R1 is a bromo, chloro or fluoro substituent.
A review of the IC50 values of series 4 in the Molt 4/C8 screen revealed the following correlations. First, the introduction of a phenyl ring onto 3a led to 4a with a 99-fold increase in potency. Various groups were placed onto ring C of 4a. While the 4-chloro analog 4h was equipotent with 4a, the introduction of methyl (4b), methoxy (4e), ethoxy (4g) and fluoro (4i) substituents led to compounds having 74%, 55%, 1% and 12% of the potency of 4a, respectively. In order to discern whether the relative biodata for 4a,b, e, g-i was controlled by the electronic, hydrophobic or steric properties of the atom or group in ring C, linear and semilogarithmic plots were constructed between the IC50 values and the Hammett sigma constants, Hansch pi figures and the molar refractivity (MR) values of the R3 group. A negative correlation was noted between the IC50 values and the σ constants (p = 0.046) revealing that potency increased as the electron-attracting properties of the aryl substituents rose. In addition, a trend towards a positive correlation with the MR constants was observed in the linear plot (p = 0.095) which suggests that potency increased as the size of the R3 substituent diminished. No other correlations were noted (p > 0.1).
Second, replacement of the R1 proton in ring A of 4b by fluorine or methyl gave rise to 4c and 4d, respectively, which have lower potencies than 4b while chloro substitution of 4e yielding 4f did not increase potency. Thus in contrast to series 3, halogenation at the R1 location did not yield compounds with lower IC50 figures than the unsubstituted analog (R1 = H). In summary, the location of a phenyl ring C on the terminal nitrogen of the thiosemicarbazono group gave a lead compound 4a with a low IC50 figure. With the exception of the 4-chloro analog 4h, substitution in ring C is deleterious in terms of cytotoxic potencies.
The dramatic increase in the potency of 4a compared to 3a is intriguing. Replacement of aryl ring C in 4a by a saturated six-membered alicyclic ring gave rise to 5. This compound is in excess of 294 times less potent than 4a. In addition, 5 is less potent than 3a. Hence the aryl ring C in series 4 may well form π-π stacking with a complementary aromatic structure at a binding site.
The general conclusions regarding the effect of molecular modifications on potencies in the Molt 4/C8 bioassay were similar for the CEM and L1210 screens. Thus in series 3, halogenation of ring A increased potencies compared to the unsubstituted analog 3a while the R2 substituents had marginal effects on the IC50 values. In the case of series 4, the conversion of 3a and 4a confirmed the importance of the aryl ring C with regard to increased potency while substitution in this ring generally did not lead to lower IC50 figures. However halogenation in ring A did increase potencies in general. Compound 5 has IC50 values in excess of 500 μM in all three screens. Linear and semilogarithmic plots were made between the IC50 values of 4a,b,e,g-i in the CEM assay and the σ, π and MR constants of the substituents R3 in ring C. Neither correlations nor trends were noted (p > 0.1). The procedure was repeated using the cytotoxicity data derived from the L1210 test. A semilogarithmic plot between the IC50 figures and the σ constants revealed a negative correlation (p = 0.046), i.e., increasing the electron-attracting properties of the R3 group led to increases in potencies. No other trends or correlations were found (p > 0.1).
The log P values of the compounds in series 3-5 were determined and are presented in . Linear, semilogarithmic and logarithmic plots were constructed between the IC50 Figures (when they were less than 500 μM) and the log P values in each of the three screens. Neither correlations nor trends were found (p > 0.1).
On the basis of the biodata generated, consideration was given pertaining to the expansion of this programme. While different avenues could be followed, the huge differential in the IC50 values of 3a, 4a and 5 in all three screens suggested that an estimate of the spatial requirements of the substituents attached to the terminal nitrogen of the thiosemicarbazono group (subsequently referred to as the N* atom) would be judicious. In this way compounds could be designed in the future to explore any correlation between the size of the group on the N* atom and cytotoxic potencies. In other words, a binding area close to the N* atom may well have strict constraints as to the size of the group on the N* atom which could be accommodated. Thus models of 3a,4a,b,e,g-i and 5 were built and the dimensions of these groups were obtained by measuring the length and breadth of the N* substituents as illustrated in ,B. In addition, the varying sizes of the N* groups may have caused differences in the relative locations of the N* substituents and the aryl ring A. Hence the spans d3 and d4 as illustrated in were determined as well as the angle ψ1 between the axes represented by d1 and d4 (). These data are presented in . From the point of view of clarity, the R3 substituents in series 4 are tabulated and, in addition, since the trends in cytotoxic potencies are similar in the three bioassays, the IC50 figures for only the Molt 4/C8 screen are presented in .
Figure 2. Determination of the spatial requirements of the atom or group attached to the terminal atom N* of the thiosemicarbazono moiety. The measurements are illustrated for4g. A. The distance d1. B. The distance d2. C. The spans d3 and d4. D. The angle ψ1.

Table II. Some data for3a, 4a,b,e,g-i and 5 obtained by molecular modeling.
The spatial requirement on the N* atom for each compound was estimated by multiplying d1 by d2 revealing an area A which is given in . The spans d4 show that the size of the N* group does not alter the relative location of the N* atom with respect to the aryl ring A. However the nature of the N* substituent causes changes in the angle ψ1. The compounds listed in may be classified into three groups G1, G2 and G3 which are potent cytotoxins G1 (IC50 values < 15 μM, 4a,b,e,h,i), weakly active compounds G2 (3a, 4g) and G3 namely the inactive thiosemicarbazone 5. In the case of G1, the figures for A, d3 and ψ1 are 27-37 sq Å, 11.4-13.6 Å and 152-160°, respectively. For group G2, the values fall outside of these ranges. Thus for 3a, the figures are 1.7 sq Å, 7.4 Å and 166°, respectively, while for 4 g the values are 43 sq Å, 14.8 Å and 163°, respectively. These differences in topological features may well influence cytotoxic potencies. The fact that the inactive cyclohexyl analog 5 has an A coordinate within the same ambit as G1 suggests that it is the aromaticity of ring C in series 4 which is responsible for the marked potency of 4a,b,e,h,i and not the size of the group on the N* atom. Thus in the future, further analogs in series 4 should be prepared for cytotoxic evaluation whose N* substituent should have varying d1 and d2 distances but retain A values in the 27-37 sq Å range. A null hypothesis could involve the placement of aromatic groups on the N* atom but whose A figures exceed 37 sq Å, e.g., by forming the corresponding naphthyl and anthryl analogs.
Finally an evaluation of the anticonvulsant potential of representative compounds was undertaken. The compounds chosen have no substituents in ring A (R1 = H in series 3 and 4) viz 3a, 4a, b,e,g-i and 5 which were examined in the maximal electroshock screen. This assay was selected since it has been claimed to be a predictor of compounds which control generalized tonic-clonic and partial seizures [Citation20]. Relatively high doses of 200 and 300 mg/kg were employed in order to observe whether any toxic symptoms (as well as anticonvulsant properties) would be observed. A reduction in the time of tonic convulsions was noted by the following compounds (the percentage decreases in the time of seizures is indicated in parentheses), namely 3a (37), 4a (26), 4b (31), 4e (34), 4g (32), 4i (27), 5 (23) and phenobarbitone (51). Thus protection in this screen was observed for all of the compounds except for 4h and in the case of 3a and 4b,e,g the reduction in the times of the seizures was the same as phenobarbitone. In the second anticonvulsant screen, the compounds were assessed for reducing the time of clonic seizures. The data in reveal that the following thiosemicarbazones reduce the time of convulsions (the percentage reductions are given in parentheses), namely 3a (59), 4a (49), 4b (55), 4e (89), 4g (72), 5 (40) and phenobarbitone (96).
Table III. Evaluation of3a, 4a, b, e, g-i and 5 for anticonvulsant properties.
The conclusion to be drawn from this survey of representative thiosemicarbazones is that, in general, these molecules have anticonvulsant properties. Lead compounds that were identified are 3a and 4a,b,e,g which controlled both tonic and clonic seizures. Furthermore the protection afforded by two of these compounds, namely 4e and 4g, towards clonic convulsions is noteworthy.
The rats became quiescent after administration of the compounds and the times taken for the animals to recover from this stupor are presented in . Only 4e and 4g prolonged the inertia of the rats compared to administration of the vehicle. In all cases, recovery was noted which is a favourable property of these compounds when considering their development as candidate antiepileptic drugs. In fact, the animals were observed for 72 hours after administration of the compounds and appear to have recovered normal functionality. In addition, this tolerance in rats is important in future molecular modifications of these series of compounds with a view to finding promising, novel anticancer agents. In other words, many of the compounds in series 3 and 4 have cytotoxic properties and coupled to low rodent toxicity, afford ample evidence for expansion of this cluster of compounds.
Conclusions
The structure of the prototype molecule 2 was modified leading to series 3 and 4 which, in general, were substantially more cytotoxic to Molt 4/C8, CEM and L1210 cells than 2. In fact, the IC50 values of many of these compounds are in the low micromolar range and in several cases exceed the potency of melphalan or were equipotent with this reference drug. Various correlations between the structures and cytotoxic potencies emerged thereby affording guidelines for expanding the project. The in vivo evaluation of representative compounds in rats revealed that protection against seizures was demonstrated and the animals tolerated doses of 200 or 300 mg/kg. A number of new lead compounds have been identified for development which may result in novel antineoplastic and/or anticonvulsant molecules with good tolerability in mammals.
Acknowledgements
The authors thank Professor B. G. Desai of the KLES College of Pharmacy, Bangalore, India for providing the necessary facilities. The Government of India, Ministry of Science and Technology, awarded a BOYSCAST Fellowship to Dr. S. S. Karki which enabled him to work at the University of Saskatchewan as a Visiting Scholar which is gratefully acknowledged. Appreciation is extended to the Belgian Fonds voor Wetenschappelijk Onderzoek (Vlaanderen) which funded the cytotoxicity assays and to Mrs. L. van Berckelaer for excellent technical assistance. Ms. B. McCullough is thanked for typing the manuscript.
Declaration of interest: The authors report no conflicts of interest. The authors alone are responsible for the content and writing of the paper.
References
- JR Dimmock, DC Smith, JM Brenner, SS Jonnalagadda, MS Sardessai, JD Wood, and GE Bigam. (1986). Antiepileptic and antileukemic thiosemicarbazones and semicarbazones of 4-aryl-3-buten-2-ones. Eur J Med Chem 21:187–192.
- JR Dimmock, SN Pandeya, JW Quail, U Pugazhenthi, TM Allen, GY Kao, J Balzarini, and E De Clercq. (1995). Evaluation of the semicarbazones, thiosemicarbazones and bis-carbohydrazones of some aryl alicyclic ketones for anticonvulsant and other biological activities. Eur J Med Chem 30:303–314.
- JR Dimmock, SS Jonnalagadda, S Hussein, S Tewari, JW Quail, RS Reid, LTJ Delbaere, and L Prasad. (1990). Evaluation of some thiosemicarbazones of arylidene ketones and analogues for anticonvulsant activities. Eur J Med Chem 25:581–588.
- JR Dimmock, RN Puthucode, JM Smith, M Hetherington, JW Quail, U Pugazhenthi, T Lechler, and JP Stables. (1996). (Aryloxy)aryl semicarbazones and related compounds: A novel class of anticonvulsant agents possessing high activity in the maximal electroshock screen. J Med Chem 39:3984–3997.
- VE Anatoly, SE Rashel, JL Margeris, AZ Aina, ZD Anda, and MK Iya. (1985). Condensation products of a-aminoisopropyl phenyl ketone, via the C = O carbonyl bond, with substituted amines, functionally substituted hydrazines or derivatives of natural amino acids, having anticancer activity. Chem Abstr 102:78567j. FR 2540868, August 17, 1984
- JR Dimmock, KK Sidhu, RS Thayer, P Mack, MJ Duffy, RS Reid, JW Quail, U Pugazhenthi, A Ong, JA Bikker, and DF Weaver. (1993). Anticonvulsant activities of some arylsemicarbazones displaying potent oral activity in the maximal electroshock screen in rats accompanied by high protection indices. J Med Chem 36:2243–2252.
- N Karali, N Terzioglu, and A Gürsoy. (2002). Syntheses and primary cytotoxicity evaluation of new 5-bromo-3-substituted-hydrazono-1H-2-indolinones. Arch Pharm Pharm Med Chem 8:374–380.
- SN Pandeya, AS Raja, and JP Stables. (2002). Synthesis of isatin semicarbazones as novel anticonvulsants – role of hydrogen bonding. J Pharm Pharmaceut Sci 5:266–271.
- CS Marvel, and GS Heirs In: AH Blatt, editor. Isatin. Organic syntheses collection. 2nd ed., Vol 1, New York: John Wiley and Sons, Inc; (1941). p 327–330.
- J Azizian, Fallah-Bagher-Shaidaei, and H Kefayati. (2003). A facile one-pot method for the preparation of N-alkyl isatins under microwave irradiation. Synth Commun 33:789–793.
- E Lieber, CN Pillai, and RD Hites. (1957). Reactions of nitrous acid with 4-substituted-thiosemicarbazides. Can J. Chem 35:832–842.
- AB Sen, and SK Gupta. (1962). Possible antiamoebic agents. Part XIX. Synthesis of 1,3,4-thiadiazolines and di-1,3,4-thiadiazolines. J Ind Chem Soc 39:628–634.
- Spartan '04 for Windows, Wavefunction, Inc., Irvine, CA 92612, USA.
- JME Molecular Editor, April 2006 version; http://www.molinspiration.com.
- Statistical Package for Social Sciences. 2005. SPSS for Windows, Release 14.0.0, SPSS Inc., Chicago.
- PB Baraldi, Nunez MDelC, MA Tabrizo, E De Clercq, J Balzarini, J Bermejo, F Estévez, and R Romagnoli. (2004). Design, syntheses and biological evaluation of hybrid molecules containing α-methylene-γ-butyrolactones and polypyrrole minor groove binders. J Med Chem 47:2877–2886.
- PN Craig. (1971). Interdependence between physical parameters and selection of substituent groups for correlation studies. J Med Chem 14:680–684.
- C Hansch, and AJ Leo. Substituent Constants for Correlation Analysis in Chemistry and Biology. New York: John Wiley and Sons; (1979). p 49.
- M Suffness, and J Douros. In: VT De Vita, and H Busch, editors. Methods in Cancer Research., Vol. XVI, Part A, New York: Academic Press; (1979). p 84. .
- EI Isaacson. In Wilson and Gisvold's Textbook of Organic Medicinal and Pharmaceutical Chemistry. 10th ed. Philadelphia: Lippincott-Raven Publishers; (1998). p 456.