Abstract
A series of monothiocarbamates (MTCs) was investigated for the inhibition of the β-class carbonic anhydrase (CAs, EC 4.2.1.1) from the fungal parasite Malassezia globosa, MgCA. These MTCs incorporate various scaffolds, among which aliphatic amine with 1–4 carbons atom in their molecule, morpholine, piperazine, as well as phenethylamine and benzylamine derivatives. All the reported MTCs displayed a better efficacy in inhibiting MgCA compared to the clinically used sulphonamide drug acetazolamide (KI of 74 μM), with KIs spanning between 1.85 and 18.9 μM. The homology model of the enzyme previously reported by us was used to rationalize the results by docking some of these MTCs within the fungal CA active site. This study might be useful to enrich the knowledge of the MgCA inhibition profile, eliciting novel ideas pertaining the design of modulators with potential efficacy in combatting dandruff or other fungal infections.
Introduction
Carbonic anhydrases (CAs, EC 4.2.1.1) are metalloenzymes present in all life kingdoms which catalyse the hydration of carbon dioxide to bicarbonate, with seven genetically distinct families described to date in various organisms, the α-, β-, γ-, δ-, ζ-, η-and θ-CAsCitation1–13. The inhibition of many such enzymes, present in mammals (in which there are 16 different isoforms)Citation14–24 or in various pathogens (fungiCitation10,Citation25–32, bacteriaCitation2,Citation4,Citation33–37, or protozoa)Citation6,Citation8,Citation10,Citation38,Citation39 may be exploited pharmacologicallyCitation1,Citation4,Citation10,Citation16,Citation40. Sulphonamides are the main class of CA inhibitors (CAIs)Citation1,Citation14,Citation41–46, but they show many side effects, and for this reason many efforts were made in the last decade in order to develop alternative classes of inhibitorsCitation14,Citation15,Citation47. In particular, the inorganic anionsCitation5,Citation13,Citation28, phenolsCitation48, polyaminesCitation49, and dithiocarbamates/xanthates/monothiocarbamates (MTCs)Citation50–55 represent interesting cases, which have been investigated in detail by kinetic and crystallographic studies. Such studies allowed a detailed understanding of the inhibition mechanisms with these classes of compounds and led to interesting drug design campaigns as well as the discovery of CAIs with a good selectivity ratio for inhibiting enzyme classes or isoforms of pharmaceutical interestCitation1,Citation4,Citation14,Citation17.
Recently, we cloned and characterized a β-CA in the pathogenic fungus provoking dandruff Malassezia globosa, MgCACitation26–28. This enzyme was shown to be an effective catalyst for the physiologic reaction, CO2 hydration to bicarbonate and protons, whereas its inhibition with sulphonamides led to growth defects of the fungus in vivo. Such results showed for the first time relevant antidandruff effects by targeting MgCA, which were equivalent to those of the standard azole drug ketoconazoleCitation26. Furthermore, we have subsequently developed a different cloning and purification strategy for this enzymeCitation27, and showed that apart sulphonamides, anions, dithiocarbamates and amino acids also constitute interesting modulators of its activityCitation27,Citation28. Finding non-sulphonamide, effective CAIs targeting MgCA seems to be a challenge not very easy to address, and this is the reason why we decided to investigate also the MTCs for this scope, as this class of CAIs was only recently reported for its interactions with human (h), α-class enzymesCitation54. Indeed, M. globosa, one of the main fungi belonging to this genus which infects humansCitation56–61, is difficult to eradicate and has developed significant resistance to most azole antifungalsCitation62.
Materials and methods
Chemistry
Compounds 1–17 used in the experiments were reported earlierCitation54.
CA assay
An applied photophysics stopped-flow instrument has been used for assaying the CA catalysed CO2 hydration activityCitation63. Bromothymol blue (at a concentration of 0.2 mM) has been used as indicator, working at the absorbance maximum of 557 nm, with 10–20 mM TRIS (pH 8.3) as buffer, and 20 mM NaBF4 for maintaining constant the ionic strength, following the initial rates of the CA-catalysed CO2 hydration reaction for a period of 10–100 s. The CO2 concentrations ranged from 1.7 to 17 mM for the determination of the kinetic parameters and inhibition constants. For each inhibitor at least six traces of the initial 5–10% of the reaction have been used for determining the initial velocity. The uncatalysed rates were determined in the same manner and subtracted from the total observed rates. Stock solutions of inhibitor (10 mM) were prepared in distilled-deionized water and dilutions up to 0.01 µM were done thereafter with the assay buffer. Inhibitor and enzyme solutions were preincubated together for 15 min at room temperature prior to assay, in order to allow for the formation of the E–I complex. The inhibition constants were obtained by non-linear least-squares methods using the Cheng–Prusoff equation whereas the kinetic parameters for the uninhibited enzymes from Lineweaver–Burk plots, as reported earlierCitation64–71, and represent the mean from at least three different determinations. MgCA was a recombinant protein, obtained and purified by a diverse procedure as the one reported earlierCitation27.
Molecular modelling studies
The dimeric form of the homology built model of MgCACitation55 was prepared for docking using the Schrodinger preparation wizard protocol that consists in preliminary pretreatment by adjusting the bond orders, metal ions and cofactors, evaluating the ionization states, adding hydrogen atoms, refining loop region and energy minimizationCitation72.
3D ligand structures were prepared by MaestroCitation73, evaluated for their ionization states at pH 7.4 ± 1.0 with EpikCitation74. The atomic electrostatic charges of the ligands were computed with JaguarCitation75 fitting them to an electrostatic potential calculated at the B3LYP/6–31 G*+ level of theory. ESP atomic charges were used in docking simulations.
Grids for docking analysis were centered in the centroid of the catalytic cavity residues. Docking studies were carried out with the program GlideCitation76. Grids for docking were centered in the centroid of the complexed ligand. The standard precision (SP) mode of the GlideScore function was applied to evaluate the predicted binding poses. The pictures were generated with MaestroCitation73.
Results and discussion
Chemistry
The rationale of this work was to investigate whether MTCs, ion analogue to the DTCs previously investigatedCitation55, show effective inhibitory action against MgCA, a β-class CA. It should be stressed that the MTCs were only recently reported as a new class of CAIs by this groupCitation54. Similarly to DTCs and to trithiocarbonate, they possess a zinc-binding group (ZBG) which may coordinate effectively to the catalytically crucial metal ion from the MgCA active site, which is a Zn(II) ionCitation26–28. The ZBG is of the CS2– type for trithiocarbonate and DTCsCitation50–55, and of the COS– type for MTCsCitation54. X-ray crystallography was successful so far only for adducts of the human (h) isoform hCA II with some DTCsCitation51, which clearly demonstrated that one sulphur of the ZBG is coordinated monodentately to the Zn(II) ion whereas the scaffold of the inhibitor participates in many favourable interactions with the enzyme active site. For MTCs, computational studies showed a similar behaviorCitation55, with the COS– ZBG coordinating (through the sulphur atom) to the metal ion from the enzyme active site. Recently, we also developed a homology model for MgCA alone and in complex with DTCsCitation55, which showed that also for the β-class enzyme, DTCs bind in a similar manner as for α-CAs, with the sulphur atom of the ZBG directly bound to the Zn(II) ion. Here, we extend the previous studies to MTCs in order to understand whether the COS– ZBG may also lead to effective MgCA inhibitors. The series of compounds 1–17 previously reportedCitation54, has been used for the enzyme inhibition measurements and includes MTCs 1–15, the trithiocarbonate 16 and the xanthate 17 (). These compounds incorporate aliphatic, aromatic and heterocyclic scaffolds, in order to explore the structure-activity relationship (SAR) of a varied chemical space for inhibition of MgCA with this class of compounds.
Table 1. hCA I, II and MgCA inhibition data with MTCs 1−15, trithiocarbonate 16 and xanthate 17 by a stopped-flow CO2 hydrase assayCitation63.
CA inhibition
Inhibition data with compounds 1–17 against the hCA isoforms (off targets) hCA I and II, as well as MgCA, with the sulphonamide inhibitor acetazolamide (AAZ) as standardCitation63, are shown in .
The following SAR for the inhibition of MgCA with compounds 1–17 can be compiled from the data of :
(i) Independently from the nature of the substituents attached to the MTC nitrogen, all reported derivatives showed better MgCA inhibitory properties than the clinically used sulphonamide drug AAZ (KI of 74 μM), with KIs ranging between 1.85 and 18.9 μM.
(ii) A rather flat SAR can be extrapolated from the data reported in . Indeed, the nature of the R and R1 groups demonstrated to possess a limited influence on the inhibitory effectiveness of compounds 1–17, which allegedly mainly lean on the ZBG interactions with the Zn ion and the residues nearby. On the other hand, it is necessary to stress the importance of lipophilic moieties (R and R1) present in the scaffold of the inhibitors, which may favour the overall interactions within the catalytic cleft, allowing by far a better inhibitory efficacy compared to simple inorganic anions (such as trithiocarbonate, CS32– which was the real lead for designing DTCs and MTCs as CAIs)Citation54.
(iii) The di-n-propyl derivative 4 demonstrated the best efficacy in inhibiting the enzyme among the reported MTCs (KI of 1.85 μM), sign that two short, unbranched aliphatic chains could better fit within the lipophilic pockets of the active site. The two secondary MTCs 1 and 2 were observed to generally possess slightly worse inhibitory potency (KI of 14.1 and 18.9 μM) compared to 4 and the remaining tertiary MTCs, showing comparable KIs (ranging between 4.22 and 9.16 μM). The only exception was represented by derivative 14, which incorporate a N-(3-Cl-phenyl)-piperazine moiety, this compound being in fact the less active tertiary MTC investigated here. In detail, the presence of a Cl atom in the meta towards the heterocyclic ring could elicit a steric hindrance within the MgCA binding pocket that reduced the KI to 15.9 μM. Finally, whereas the benzyl trithiocarbonate 16 possessed a KI comparable to those of most MTCs (KI of 8.12 μM), the phenethyl xanthate 17 showed a roughly two-fold reduced efficacy (KI of 16.2 μM).
(iv) The effectiveness of MTCs in inhibiting the MgCA was shown to be scarcely reduced, but anyhow comparable, to that of the DTCs investigated earlier by usCitation55. Diversely, the inhibition profile of the MTCs against MgCA was very poor in comparison to that of the human isoforms, which were more sensitive to this class of inhibitors. Notwithstanding no MgCA-selective inhibitors were found, the MTCs have been validated as a new class of MgCA inhibitors, and it is worth that they should be considered, alongside with the DTCs, as attractive for further investigations to discover more potent and selective fungal CAIs.
Molecular modelling studies
Docking simulations were performed to elucidate the binding mode of MTCs within the MgCA active site. Four inhibitors from (compounds 2, 8, 9 and 10) endowed with acceptable inhibitory properties and a varied structure were selected as representatives of the synthesized MTCs. These derivatives were submitted to quantum mechanics optimization (B3LYP/6–31 G*+) in order to compute the charge distribution and optimal geometry, prior to dock the molecules into the recently developed homology-built model of MgCACitation55. According to previously reported evidenceCitation54,Citation77, points of high electron density surface are located close to the sulphur atom of the MTC (as in the DTCs previously investigated). The active site of the enzyme comprises residues from the two monomers (chain A and B) that form the quaternary structure, and the catalytic zinc ion is coordinated by the side chains of C47, H103 and C106. The lowest energy docking solutions suggest that the fourth Zn coordination position can be occupied either by sulphur or oxygen atoms of the MTC inhibitor. However, based on the findings obtained by QM calculation () and on the previous spectroscopic and crystallographic studiesCitation77, which agreed in indicating that the negative charge distribution is mainly localized on sulphur, poses were selected in which the sulphur atom binds in tetrahedral coordination geometry to the catalytic zinc ion from the enzyme active site. The oxygen atom of the MTC moiety was, on the other hand, found in H-bond distance from residues S48 (chain B) and Q38 (chain A), depending on the selected pose ().
Figure 1. (a) ESP atomic charges of 2 derived from a B3LYP/6–31 G*+. Red colour represents negative values of the electrostatic potential (b) Schematic representation of the binding mode of MTCs into the MgCA active site.
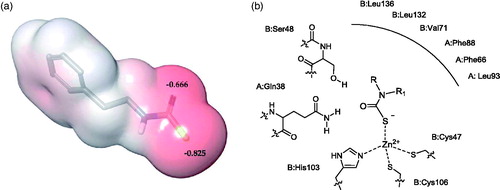
The scaffold fragments of the four derivatives accommodate into a hydrophobic pocket defined by residues from both monomers. π–π interactions occur between the phenyl moieties of derivatives 2a and 9a and the side chain of F88(A). The benzyl and phenethyl tails of these derivatives were further stabilized by the π-alkyl interactions established with the aliphatic side chain of V71(B) and L132(B) (). These same three residues and L136(B) were involved in hydrophobic interactions with the ethyl group of the ester function of 8 (). CH···π interactions were also observed for the N-methyl group of the zinc-binding group moiety of 8 and 9 and the side chains of F66 and L93 from monomer A. Alkyl- and π-alkyl interactions were also observed for the morpholine ring of 10 and the side chains of V71(B) and F88(A), respectively ().
Figure 2. Docked orientations of compounds 2 and 9 (a); 8 (b) and 10 (c) within MgCA active site. Monomer A and B are coloured blue and green, respectively.
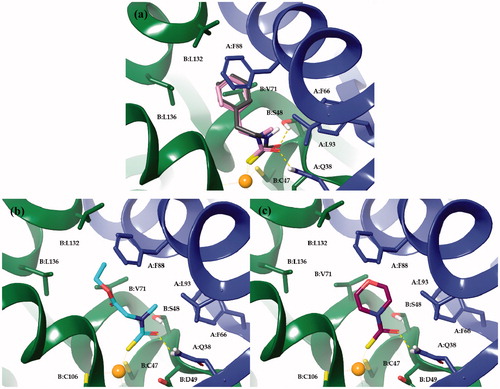
Compared to the predicted binding mode of DTCs, which form H-bond with both S48 (chain B) and Q38 (chain A) residues, the oxygen atom of the MTC was able to bind only to the side chain OG atom of S48 or NE2 atom of Q38. Hence, it is reasonable to hypothesize that the shorter length of the CO bond (1.25 Å) compared to that of the CS (1.75 Å) oneCitation77 may contribute to the generally worse inhibitory profile of MTCs compared to DTCs.
Conclusions
Kinetic and computational approaches were applied to investigate a series of MTCs as novel inhibitors of the β-class carbonic anhydrase from the fungal parasite M. globosa, a validated anti-dandruff drug targetCitation55,Citation78. All the reported MTCs displayed better MgCA inhibition profile than to the clinically used sulphonamide drug acetazolamide (KI of 74 μM), with KIs spanning between 1.85 and 18.9 μM. Docking procedures were applied to the homology model of the enzyme we previously reported to shed light on the binding mode the MTCs exhibited within the fungal CA active site. This study might be of help to better decipher the MgCA inhibition profile, raising the discovery of novel modulators with potential efficacy in combatting dandruff or other fungal infections.
Disclosure statement
No potential conflict of interest was reported by the authors.
References
- Supuran CT. Carbonic anhydrases: novel therapeutic applications for inhibitors and activators. Nature Rev Drug Discov 2008;7:168–81.
- Capasso C, Supuran CT. An overview of the alpha-, beta- and gamma-carbonic anhydrases from bacteria: can bacterial carbonic anhydrases shed new light on evolution of bacteria? J Enzyme Inhib Med Chem 2015;30:325–32.
- Zimmerman SA, Ferry JG, Supuran CT. Inhibition of the archaeal beta-class (Cab) and gamma-class (Cam) carbonic anhydrases. Curr Top Med Chem 2007;7:901–8.
- Supuran CT. Bacterial carbonic anhydrases as drug targets: towards novel antibiotics? Front Pharmacol 2011;2:34.
- Del Prete S, Vullo D, Scozzafava A, et al. Cloning, characterization and anion inhibition study of the δ-class carbonic anhydrase (TweCA) from the marine diatom Thalassiosira weissflogii. Bioorg Med. Chem 2014;22:531–7.
- Del Prete S, Vullo D, Fisher GM, et al. Discovery of a new family of carbonic anhydrases in the malaria pathogen Plasmodium falciparum – the η-carbonic anhydrases. Bioorg Med Chem Lett 2014;24:4389–96.
- Ferry JF. The gamma class of carbonic anhydrases. Biochim Biophys Acta 2010;1804:374–81.
- Smith KS, Jakubzick C, Whittam TS, et al. Carbonic anhydrase is an ancient enzyme widespread in prokaryotes. Proc Natl Acad Sci USA 1999;96:15184–9.
- Kikutani S, Nakajima K, Nagasato C, et al. Thylakoid luminal θ-carbonic anhydrase critical for growth and photosynthesis in the marine diatom Phaeodactylum tricornutum. Proc Natl Acad Sci USA 2016;113:9828–33.
- Capasso C, Supuran CT. Anti-infective carbonic anhydrase inhibitors: a patent and literature review. Expert Opin Ther Pat 2013;23:693–704.
- Alterio V, Langella E, Viparelli F, et al. Structural and inhibition insights into carbonic anhydrase CDCA1 from the marine diatom Thalassiosira weissflogii. Biochimie 2012;94:1232–41.
- Vullo D, Del Prete S, Osman SM, et al. Sulfonamide inhibition studies of the δ-carbonic anhydrase from the diatom Thalassiosira weissflogii. Bioorg Med Chem Lett 2014;24:275–9.
- Viparelli F, Monti SM, De Simone G, et al. Inhibition of the R1 fragment of the cadmium-containing zeta-class carbonic anhydrase from the diatom Thalassiosira weissflogii with anions. Bioorg Med Chem Lett 2010;20:4745–8.
- Alterio V, Di Fiore A, D’Ambrosio K, et al. Multiple binding modes of inhibitors to carbonic anhydrases: how to design specific drugs targeting 15 different isoforms? Chem Rev 2012;112:4421–68.
- Supuran CT. How many carbonic anhydrase inhibition mechanisms exist? J Enzyme Inhib Med Chem 2016;31:345–60.
- Neri D, Supuran CT. Interfering with pH regulation in tumours as a therapeutic strategy. Nat Rev Drug Discov 2011;10:767–77.
- Supuran CT. Structure-based drug discovery of carbonic anhydrase inhibitors. J Enzyme Inhib Med Chem 2012;27:759–72.
- De Simone G, Supuran CT. Carbonic anhydrase IX: biochemical and crystallographic characterization of a novel antitumor target. Biochim Biophys Acta 2010;1804:404–9.
- Supuran CT. Carbonic anhydrase inhibitors: an editorial. Expert Opin Ther Pat 2013;23:677–9.
- Supuran CT. Carbonic anhydrase inhibition/activation: trip of a scientist around the world in the search of novel chemotypes and drug targets. Curr Pharm Des 2010;16:3233–45.
- Supuran CT. Structure and function of carbonic anhydrases. Biochem J 2016;473:2023–32.
- Fabrizi F, Mincione F, Somma T, et al. A new approach to antiglaucoma drugs: carbonic anhydrase inhibitors with or without NO donating moieties. Mechanism of action and preliminary pharmacology. J Enzyme Inhib Med Chem 2012;27:138–47.
- Ekinci D, Karagoz L, Ekinci D, et al. Carbonic anhydrase inhibitors: in vitro inhibition of α isoforms (hCA I, hCA II, bCA III, hCA IV) by flavonoids. J Enzyme Inhib Med Chem 2013;28:283–8.
- Borras J, Scozzafava A, Menabuoni L, et al. Carbonic anhydrase inhibitors. Synthesis of water-soluble, topically effective intraocular pressure lowering aromatic/heterocyclic sulfonamides containing 8-quinoline-sulfonyl moieties: is the tail more important than the ring? Bioorg Med Chem 1999;7:2397–406.
- Supuran CT, Scozzafava A, Mastrolorenzo A. Bacterial proteases: current therapeutic use and future prospects for the development of new antibiotics. Expert Opin Ther Pat 2001;11:221–59.
- Hewitson KS, Vullo D, Scozzafava A, et al. Molecular cloning, characterization, and inhibition studies of a β-carbonic anhydrase from Malassezia globosa, a potential antidandruff target. J Med Chem 2012;55:3513–20.
- Del Prete S, De Luca V, Vullo D, et al. A new procedure for the cloning, expression and purification of the β-carbonic anhydrase from the pathogenic yeast Malassezia globosa, an anti-dandruff drug target. J Enzyme Inhib Med Chem 2016;31:1156–61.
- Del Prete S, Vullo D, Osman SM, et al. Anion inhibition studies of the dandruff-producing fungus Malassezia globosa β-carbonic anhydrase MgCA. Bioorg Med Chem Lett 2015;25:5194–8. 15
- Singh S, Supuran CT. In silico modeling of β-carbonic anhydrase inhibitors from the fungus Malassezia globosa as antidandruff agents. J Enzyme Inhib Med Chem 2016;31:417–24.
- Vullo D, Del Prete S, Capasso C, et al. Carbonic anhydrase activators: activation of the β-carbonic anhydrase from Malassezia globosa with amines and amino acids. Bioorg Med Chem Lett 2016;26:1381–5.
- Innocenti A, Hall RA, Scozzafava A, et al. Carbonic anhydrase activators: activation of the beta-carbonic anhydrases from the pathogenic fungi Candida albicans and Cryptococcus neoformans with amines and amino acids. Bioorg Med Chem 2010;18:1034–7.
- Nocentini A, Vullo D, Bartolucci G, et al. N-nitrosulfonamides: a new chemotype for carbonic anhydrase inhibition. Bioorg Med Chem 2016;24:3612–17.
- Del Prete S, Isik S, Vullo D, et al. DNA cloning, characterization, and inhibition studies of an α-carbonic anhydrase from the pathogenic bacterium Vibrio cholerae. J Med Chem 2012;55:10742–8.
- Vullo D, Nishimori I, Scozzafava A, et al. Inhibition studies of a beta-carbonic anhydrase from Brucella suis with a series of water soluble glycosyl sulfanilamides. Bioorg Med Chem Lett 2010;20:2178–82.
- Maresca A, Vullo D, Scozzafava A, et al. Inhibition of the alpha- and beta-carbonic anhydrases from the gastric pathogen Helycobacter pylori with anions. J Enzyme Inhib Med Chem 2013;28:388–91.
- Maresca A, Scozzafava A, Vullo D, Supuran CT. Dihalogenated sulfanilamides and benzolamides are effective inhibitors of the three β-class carbonic anhydrases from Mycobacterium tuberculosis. J Enzyme Inhib Med Chem 2013;28:384–7.
- Maresca A, Carta F, Vullo D, Supuran CT. Dithiocarbamates strongly inhibit the β-class carbonic anhydrases from Mycobacterium tuberculosis. J Enzyme Inhib Med Chem 2013;28:407–11.
- Vullo D, Del Prete S, Fisher GM, et al. Sulfonamide inhibition studies of the η-class carbonic anhydrase from the malaria pathogen Plasmodium falciparum. Bioorg Med Chem 2015;23:526–31.
- Vermelho AB, Capaci GR, Rodrigues IA, et al. Carbonic anhydrases from Trypanosoma and Leishmania as anti-protozoan drug targets. Bioorg Med Chem 2017;25:1543–55.
- Supuran CT. Carbonic anhydrase inhibitors in the treatment and prophylaxis of obesity. Expert Opin Ther Pat 2003;13:1545–50.
- Supuran CT. Carbonic anhydrase inhibitors. Bioorg Med Chem Lett 2010;20:3467–74.
- Carta F, Scozzafava A, Supuran CT. Sulfonamides: a patent review (2008–2012). Expert Opin Ther Pat 2012;22:747–58.
- Wilkinson BL, Bornaghi LF, Houston TA, et al. Carbonic anhydrase inhibitors: inhibition of isozymes I, II, and IX with triazole-linked O-glycosides of benzene sulfonamides. J Med Chem 2007;50:1651–7.
- Scozzafava A, Menabuoni L, Mincione F, et al. Carbonic anhydrase inhibitors: synthesis of sulfonamides incorporating dtpa tails and of their zinc complexes with powerful topical antiglaucoma properties. Bioorg Med Chem Lett 2001;11:575–82.
- Alp C, Özsoy Ş, Alp NA, et al. Sulfapyridine-like benzenesulfonamide derivatives as inhibitors of carbonic anhydrase isoenzymes I, II and VI. J Enzyme Inhib Med Chem 2012;27:818–24.
- Scozzafava A, Briganti F, Mincione G, et al. Carbonic anhydrase inhibitors: synthesis of water-soluble, aminoacyl/dipeptidyl sulfonamides possessing long-lasting intraocular pressure-lowering properties via the topical route. J Med Chem 1999;42:3690–700.
- Bonneau A, Maresca A, Winum JY, et al. Metronidazole-coumarin conjugates and 3-cyano-7-hydroxy-coumarin act as isoform-selective carbonic anhydrase inhibitors. J Enzyme Inhib Med Chem 2013;28:397–401.
- Entezari Heravi Y, Bua S, Nocentini A, et al. Inhibition of Malassezia globosa carbonic anhydrase with phenols. Bioorg Med Chem 2017;25:2577–82.
- Briganti F, Pierattelli R, Scozzafava A, Supuran CT. Carbonic anhydrase inhibitors. Part 37. Novel classes of isozyme I and II inhibitors and their mechanism of action. Kinetic and spectroscopic investigations on native and cobalt-substituted enzymes. Eur J Med Chem 1996;31:1001–10.
- Carta F, Aggarwal M, Maresca A, et al. Dithiocarbamates strongly inhibit carbonic anhydrases and show antiglaucoma action in vivo. J Med Chem 2012;55:1721–30.
- Carta F, Aggarwal M, Maresca A, et al. Dithiocarbamates: a new class of carbonic anhydrase inhibitors. Crystallographic and kinetic investigations. Chem Commun (Camb) 2012;48:1868–70.
- Bozdag M, Carta F, Vullo D, et al. Synthesis of a new series of dithiocarbamates with effective human carbonic anhydrase inhibitory activity and antiglaucoma action. Bioorg Med Chem 2015;23:2368–76.
- Bozdag M, Carta F, Vullo D, et al. Dithiocarbamates with potent inhibitory activity against the Saccharomyces cerevisiae β-carbonic anhydrase. J Enzyme Inhib Med Chem 2016;31:132–6.
- Vullo D, Durante M, Di Leva FS, et al. Monothiocarbamates strongly inhibit carbonic anhydrases in vitro and possess intraocular pressure lowering activity in an animal model of glaucoma. J Med Chem 2016;59:5857–67.
- Vullo D, Del Prete S, Nocentini A, et al. Dithiocarbamates effectively inhibit the β-carbonic anhydrase from the dandruff-producing fungus Malassezia globosa. Bioorg Med Chem 2017;25:1260–5.
- Clavaud C, Jourdain R, Bar-Hen A, et al. Dandruff is associated with disequilibrium in the proportion of the major bacterial and fungal populations colonizing the scalp. PLoS One 2013;8:e58203.
- Dawson TL Jr, Malassezia globosa and restricta: breakthrough understanding of the etiology and treatment of dandruff and seborrheic dermatitis through whole-genome analysis. J Investig Dermatol Symp Proc 2007;12:15–19.
- Guillot J, Hadina S, Guého E. The genus Malassezia: old facts and new concepts. Parassitologia 2008;50:77–9.
- Galuppi R, Tampieri MP. Epidemiology and variability of Malassezia spp. Parassitologia 2008;50:73–6.
- Angiolella L, Carradori S, Maccallini C, et al. Targeting Malassezia species for novel synthetic and natural antidandruff agents. Curr Med Chem 2017. doi: 10.2174/0929867324666170404110631
- Wang L, Clavaud C, Bar-Hen A, et al. Characterization of the major bacterial-fungal populations colonizing dandruff scalps in Shanghai, China, shows microbial disequilibrium. Exp Dermatol 2015;24:398–400.
- Cabanes JF. Malassezia yeasts: how many species infect humans and animals? Plos Pathogen 2014;10:e1003892.
- Khalifah RG. The carbon dioxide hydration activity of carbonic anhydrase. I. Stop-flow kinetic studies on the native human isoenzymes B and C. J Biol Chem 1971;246:2561–73.
- Sahin A, Isık S, Arslan O, et al. A new affinity gel for the purification of α-carbonic anhdrases. J Enzyme Inhib Med Chem 2015;30:224–8.
- De Luca V, Del Prete S, Supuran CT, et al. Protonography, a new technique for the analysis of carbonic anhydrase activity. J Enzyme Inhib Med Chem 2015;30:277–82.
- Del Prete S, Vullo D, De Luca V, et al. Biochemical characterization of recombinant β-carbonic anhydrase (PgiCAb) identified in the genome of the oral pathogenic bacterium Porphyromonas gingivalis. J Enzyme Inhib Med Chem 2015;30:366–70.
- Eldehna WM, Al-Ansary GH, Bua S, et al. Novel indolin-2-one-based sulfonamides as carbonic anhydrase inhibitors: synthesis, in vitro biological evaluation against carbonic anhydrases isoforms I, II, IV and VII and molecular docking studies. Eur J Med Chem 2017;127:521–30.
- Migliardini F, De Luca V, Carginale V, et al. Biomimetic CO2 capture using a highly thermostable bacterial α-carbonic anhydrase immobilized on a polyurethane foam. J Enzyme Inhib Med Chem 2014;29:146–50.
- Nocentini A, Ferraroni M, Carta F, et al. Benzenesulfonamides incorporating flexible triazole moieties are highly effective carbonic anhydrase inhibitors: synthesis and kinetic, crystallographic, computational, and intraocular pressure lowering investigations. J Med Chem 2016;59:10692–704.
- Abdel-Aziz AA, El-Azab AS, Ekinci D, et al. Investigation of arenesulfonyl-2-imidazolidinones as potent carbonic anhydrase inhibitors. J Enzyme Inhib Med Chem 2015;30:81–4.
- Akdemir A, De Monte C, Carradori S, Supuran CT. Computational investigation of the selectivity of salen and tetrahydrosalen compounds towards the tumor-associated hCA XII isozyme. J Enzyme Inhib Med Chem 2015;30:114–18.
- Schrödinger Release 2016-1: Schrödinger Suite 2016-1 Protein Preparation Wizard; Epik, Schrödinger, LLC, New York, NY, 2016; Impact, Schrödinger, LLC, New York, NY, 2016; Prime, Schrödinger, LLC, New York, NY, 2016.
- Schrödinger Release 2016-1: Maestro, Schrödinger, LLC, New York, NY, 2016.
- Schrödinger Release 2016-1: Epik, Schrödinger, LLC, New York, NY, 2016.
- Schrödinger Release 2016-1: Jaguar, Schrödinger, LLC, New York, NY, 2016
- Schrödinger Release 2016-1: Glide, Schrödinger, LLC, New York, NY, 2016.
- Greene DL, McCormick BJ, Pierpont CG. Amine adducts of thiocarbamate complexes. crystal and molecular structure of bis(cyc1opentamethylene thiocarbamato)bis(piperidine)zinc(II). Inorg. Chem 1973;12:2148–52.
- Chohan ZH, Arif M, Akhtar MA, et al. Metal-based antibacterial and antifungal agents: synthesis, characterization, and in vitro biological evaluation of Co(II), Cu(II), Ni(II), and Zn(II) complexes with amino acid-derived compounds. Bioinorg Chem Appl 2006;83131.