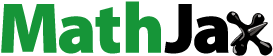
Abstract
Aiming at finding natural sources of antidiabetics agents, 15 extracts from Brazilian medicinal plants of the Atlantic Forest and Amazon region were tested against α-glucosidase enzyme. Plants were selected based on the taxonomic relationships with genera including several species with antidiabetic activity. In this screening, the extracts obtained from the flowers of Hyptis monticola and the leaves of Lantana trifolia and Lippia origanoides resulted endowed with promising anti-α-glucosidase activity. The extracts from H. monticola and from L. origanoides collected in two different areas, were characterised by ultra-high performance liquid chromatography coupled to mass spectrometry. Bioassay-guided fractionation led to the identification of several enzyme inhibiting compounds, among them the mechanism of action of naringenin and pinocembrin was investigated. The two L. origanoides extracts showed differences in bioactivity and in the phytochemical profiles. The fractionation of the extract from H. monticola led to a partial loss of the inhibitory effect.
1. Introduction
Inhibition of α-glucosidase, an enzyme involved in carbohydrates digestion, represents a strategy to treat type 2 diabetes mellitus. α-Glucosidase is the key enzyme catalysing the final step in the digestive process of carbohydrates, It is an exo-type carbohydrase distributed widely in microorganisms, plants, and animal tissuesCitation1 which catalyses the liberation of α-glucose from the non-reducing end of the substrateCitation2. Hence, α-glucosidase inhibitors can retard the liberation of α-glucose from dietary complex carbohydrates and delay glucose absorption, resulting in reduced postprandial plasma glucose levels and suppression of postprandial hyperglycemiaCitation3,Citation4. In recent years, many efforts have been made to identify effective α-glucosidase inhibitors from natural sources to develop a physiologic functional food or lead compounds for use against diabetes. Several α-glucosidase inhibitors that are phytoconstituents, such as flavonoids, alkaloids, terpenoids, anthocyanins, glycosides, phenolic compounds, among others, have been isolated from plantsCitation5. Brazil comprises several areas particularly rich in biodiversityCitation6, as in the case of the Amazon region and the Atlantic ForestCitation7,Citation8. Covering several climatic belts and a wide variety of altitudes and soil types, the Amazon rainforest hosts more than 50,000 plant speciesCitation9, while the Atlantic Forest hosts more than 20,000Citation10. Starting from a chemotaxonomic approach, 15 extracts from plant species of the Brazilian Atlantic Forest and Amazon region were tested against α-glucosidase enzyme. The selected plants belong to plant genera for which anti-diabetic activity is documentedCitation11–26. Specifically, medicinal plants from five genera, namely Lippia, Lantana, Hyptis, Solanum, and Struthanthus were tested in this study. Modern mass spectrometry (MS) is being successfully used for natural products discovery, in the characterisation of components in plant extracts mixtures, providing data on weight and molecular formula, enabling the identification of characteristic fragments of a moleculeCitation27. The development of new powerful tools to process MS data, such as MZmine 2 software, have allowed faster dereplication and structural elucidation of metabolites, which is leading to a boom in screening new biologically active compounds. In this way, the extracts inhibiting α-glucosidase enzyme were characterised by ultra-high performance liquid chromatography coupled to MS.
2. Experimental
2.1. General procedure
1H (400 MHz), 13C (100 MHz), and 2D NMR spectra were obtained using standard programs on a Bruker 400 MHz spectrometer (Billerica, MA) with TMS as internal reference, using DMSO-d6 as solvent, δ in ppm, and J in Hz.
Countercurrent chromatography separations were performed on a P.C. Inc. (Potomac, MD) apparatus equipped with a triple polytetrafluoroethylene multi-layer coil (15 mL + 80 mL + 280 mL, 1.6 mm i.d.) equilibrated by a counterweight. The rotation speed is adjustable from 0 to 1000 rpm. The 80-mL coil was used in all experiments. The solvents were pumped with a HPLC Solvent Delivery System Model M-45 Waters (Milford, MA) and the fractions were collected in a Super Fraction Collector SF-2120 Advantec MFS Inc. (Tokyo, Japan). The solvent systems tests were performed as follows: small amounts of a sample extract were dissolved in a test tube containing a two-phase solvent system. After shaking and allowing compounds’ partition between the two phases, equal aliquots of each phase were spotted individually on silica gel TLC plates to determine the distribution coefficients (KD) by visual inspection. Solvent systems used in all separations by CCC were prepared in a separatory funnel at room temperature. After the equilibrating, the two phases were separated and degassed by sonication for 5 min. In each run, a CCC column was first filled with the stationary phase, after setting the rotation, the mobile phase was pumped in. Samples were dissolved in equal volumes of phases and were injected after the hydrodynamic equilibrium inside the column was reached.
2.2. Plant material
Plant material and extraction procedures were previously reported for: Hyptis monticolaCitation28, Lippia rubellaCitation29, Lippia lacunosaCitation30, Lippia origanoidesCitation31, Lantana fucataCitation32, Lantana trifoliaCitation33, and Struthanthus concinnnusCitation34. Lippia aristata and Lippia brasiliensis were collected in April 2015 from cultivated specimens at the Plant Experimental Area of the Federal University of Juiz de Fora, Minas Gerais, Brazil. Dr. Fátima Regina Gonçalves Salimena identified the plant material. Voucher specimens were deposited in the Herbarium Leopoldo Krieger, Universidade Federal de Juiz de Fora, under the accession numbers CESJ 48.652 and 48.652. Lippia origanoides from Videiras Vale was collected at Petropolis (S 22°25.184′, W 43°17.044′, elevation 1364 m), Rio de Janeiro State, in October 2013 (authorisation number SISBIO 37042-5), and Solanum gilo fruits were purchased from Ceasa food market, Rio de Janeiro, Brazil. Plants were extracted exhaustively by percolation with ethanol (95%, eight times, 1:5 w/v). The resulting extracts were filtered and concentrated under reduced pressure at 40 °C, using a vacuum rotary evaporator. Part of the ethanol extracts were separately suspended with water and then submitted to liquid − liquid partition with organic solvents to afford hexane, dichloromethane, ethyl acetate, and n-butanol extracts.
2.3. α-Glucosidase inhibitory assay
α-Glucosidase from Saccharomyces cerevisiae (E.C. 3.2.1.20) was purchased from Sigma-Aldrich Co (St. Louis, MO) and α-glucosidase inhibition assay was performed according to Venditti et al.Citation35 with slight modifications. Samples were prepared in DMSO, thus the final concentration of DMSO in the assay was 20% v/v, and negative control was performed in the same conditions. Considering that one enzyme unit will liberate 1.0 µmol of d-glucose from p-nitrophenyl-α-d-glucopyranoside (p-NPG) per min at pH 6.8 and 37 °C, 1 mU of enzyme prepared in 0.1 M phosphate buffer pH 6.8 was incubated for 5 min with test samples in the same conditions. The synthetic substrate p-NPG, prepared in buffer, was added at final concentration of 2 mM, to start the reaction with a final volume of 200 µL. The formation of p-nitrophenol from the hydrolysis of the substrate was immediately monitored for 5 min at 405 nm under constant temperature of 37 °C using a microplate reader (Victor™ X3 PerkinElmer, Waltham, MA). The percentage of inhibition of enzyme activity was calculated by the following formula:
The IC50 values (concentration necessary for 50% inhibition of enzyme activity) were calculated by constructing a logarithmic curve with the sample concentrations on x-axes and the percentage inhibition on y-axes. As positive control acarbose was used showing an IC50 of 105 µM. A negative control was obtained by adding water instead of samples.
The Lineweaver–Burk (L–B) plots were constructed to calculate the kinetic parameters (KM expressed in mM and Vmax in µkat) of the enzymatic reaction without and with the bioactive compounds at the IC50 concentration. Different p-NPG concentrations were used in the range 2–0.25 mM. The rate of the enzymatic reaction expressed in µkat was calculated from ΔAbs min, considering p-nitrophenol Ɛ at 405 nm = 18.5 mM−1 cm−1 and light path length = 0.8 cm.
2.4. Statistical analysis
The kinetic parameters Vmax and KM, and IC50 values of the two extracts from L. origanoides (LOM and LOVV) were expressed as the mean ± standard deviation (SD) of three independent experiments with samples in duplicate. The statistical analyses were performed with GraphPad Prism version 5.00 (GraphPad Software, San Diego, CA), the values were compared by one-way analysis of variance (ANOVA) followed by Tukey’s post hoc test, considering significant differences at p values <.05.
2.5. LC–MS/MS conditions
The ultra-high performance liquid chromatography with ultraviolet detector coupled to tandem mass spectrometry (UHPLC-UV-MS/MS) analyses were performed using a UHPLC Dionex UltiMate 3000 system, coupled to a LCQ Fleet mass spectrometer (Thermo Fisher Scientific, Waltham, MA), consisting of an oven, solvent degasser, Ultra High-Pressure pump, an autosampler, a diode array detector and a column temperature manager. An Acquity UPLC BEH C18 column (2.1 × 150 mm, 1.7 µ, Waters, Milford, MA), was used at a flow rate of 0.25 mL min−1. The column temperature was 40 °C and the mobile phase was water containing 0.1% formic acid (A) and MeOH (B). The gradient elution program is as follows: 0–1 min, 10% (B); 1–20 min, 10–35% (B); 20–35 min, 35–45% (B); 35–40 min, 45% (B); 40–55 min, 45–80% (B); 55–56 min, 80–95% (B); 56–60 min, 95% (B). After each injection, cleaning (98% B, 6 min) and column re-equilibration (7 min) were performed. The MS, equipped with an electrospray source (ESI), was operated in positive ionisation mode. High purity nitrogen (N2) was used as sheath gas (35 arbitrary units) and auxiliary gas (10 arbitrary units). High purity helium (He) was used as collision gas. The MS parameters were as follows: source voltage 5 kV, capillary voltage 7 V, tube lens voltage 65 V, and capillary temperature 400 °C. MS spectra were acquired by full range acquisition covering 140–1500 m/z. For fragmentation study, a data dependent scan was performed, and the normalised collision energy of the collision-induced dissociation (CID) cell was set at 30 eV and the isolation width of precursor ions was set at m/z 2.0.
2.6. Data processing and dereplication
Data obtained from LC–MS analyses was converted to mzML format using the Proteowizard software. Then, data were processed in Mzmine 2.53 and exported in .mgf format to GNPS platform, where dereplication proceeded. The dereplication parameters were as follows: Precursor ion and fragment ion mass tolerances: 0.3 Da; minimum matched peaks: 6 and score threshold: 7. After processing, data were downloaded and evaluated against literature information to corroborate the suggestions made by the platform.
2.7. Fractionation of active plant extracts by high-speed countercurrent chromatography (HSCCC)
2.7.1. HSCCC separation LOVV (Lippia origanoides from Videiras Valley) by isocratic elution
Part of the LOVV extract (1 g), dissolved in 10 mL of the biphasic solvent system, was fractionated by HSCCC with the solvent system hexane–ethanol–water (4:3:1), organic as mobile phase, flow rate was 2 mL/min, 850 rpm. The stationary phase retention was 82.5% (Vm=12 mL, Sf=82.5%). Fractions of 4 mL/tube were collected, rotor rotation was stopped at tube 80 and the column content was extruded, totalising 160 tubes. Fractions were analysed by TLC (solvent systems I and II) and those with similar profile were pooled together to afford nine fractions as follows: F01 (tubes 1–23); F02 (tubes 24–45); F03 (tubes 46–65); F04 (tubes 66–101); F05 (tubes 102–105); F06 (tubes 106–107); F07 (tubes 108–109); F08 (tubes 110–111) and F09 (tubes 112–160). Pinocembrin, present in fraction F05 was isolated as previously describedCitation31. This isolated compound was used as standard for the identification and to carry out the enzymatic inhibition assays. Naringenin, present in fractions F08, was identified by comparison with the standard from Sigma-Aldrich (Merck, Darmstadt, Germany). The standard was used to perform the enzyme assays.
2.7.2. HSCCC separation Hyptis monticola ethyl acetate extract
Part of the Hyptis monticola extract (0,4 g) dissolved in 10 mL of the biphasic solvent system was fractionated by HSCCC with the solvent system hexane–ethyl acetate–butyl alcohol–water (2:5:1:5), organic as stationary phase, flow rate was 2 mL/min, 850 rpm. The stationary phase retention was 70% (Vm=56 mL, Sf=70%). Fractions of 4 mL/tube were collected; rotor rotation was stopped at tube 40. At tube 80, the stationary phase was pumped, and the column content was extruded until tube 120. Fractions were analysed by TLC (solvent system dichloromethane–ethyl acetate–formic acid–methanol, 5:5:1.5:0.5) and those with similar profile were pooled together to afford 23 fractions, as follows: F1 (tubes 1–8); F2 (tubes 9–11) identified by comparison with a standard as rutin; F3 (tube 12); F4 (tubes 13–17), identified by 1H NMR and 13C NMR as a mixture of hyperoside and isoquercetin (Tables S1 and S2); F5 (tubes 18–20); F6 (tubes 21–24); F7 (tube 25); F8 (tubes 26–28); F9 (tubes 29–32); F10 (tubes 33–39); F11 (tubes 40–42); F12 (tubes 43–47); F13 (tubes 48–51); F14 (tubes 52–54); F15 (tubes 55–57); F16 (tubes 58–62); F17 (tubes 63–64)) , annotated by UHPLC-ESI-MS/MS as a quercetin isomer; F18 (tubes 65–68) annotated by UHPLC-ESI-MS/MS as a quercetin isomer; F19 (tubes 69–71); F20 (tubes 72–82); F21 (tubes 83–99) identified by comparison with a standard as quercetin; F22 (tubes 100–101), annotated by UHPLC-ESI-MS/MS as a mixture of luteolin and aromadendrin and F23 (tubes 102–120).
3. Results and discussion
The 15 extracts were first screened at a fixed concentration of 100 µg/mL. To achieve the complete solubilisation of the extracts, DMSO and 0.1 M phosphate buffer pH 6.8 were used as solvents. The effects of DMSO were evaluated by following the kinetics of the enzymatic inhibition reaction in presence and in the absence of DMSO, testing p-NPG concentrations in the range 2–0.25 mM. The use of 20% DMSO in the final volume of the assay did not change the kinetics parameters of the enzyme; hence, all samples were tested maintaining this ratio. The extracts showing inhibition higher than 30% at concentration of 100 µg/mL were considered active (), thus their IC50 values were calculated. The selected samples were the ethyl acetate extract from the flowers of Hyptis monticola, the hexane extract from the leaves of Lantana trifolia and the ethanolic extracts from the leaves of Lippia origanoides collected in two different areas. Specifically, one sampling was carried out in Manaus, in the North of Brazil, in the Amazon region, at approximately 50 m above the sea level (extract LOM). A second harvest was made in Videiras Valley, Southeast of Brazil, in the Atlantic Forest biome, at altitude above 1300 m (extract LOVV). The IC50s of the active extracts resulted between 15 and 115 µg/mL, being the most active one that of H. monticola. The chemical profiles of the three most active extracts were obtained by ultra-high performance liquid chromatography coupled to tandem MS aligned in .MZmine (). Tentative metabolite identifications/assignments were made by interpreting MS fragment ions (fragmentation patterns) combined with chemotaxonomic data reported in the literature and databases such as GNPS (Global Natural Products Social) software ( and ).
Figure 1. Aligned chromatograms of (A) Hyptis monticola, (B) Lippia origanoides from Manaus, and (C) Lippia origanoides from Videiras Valley obtained by UHPLC-ESI(+)3D-IT-MS/MS.

Table 1. In vitro inhibition of α-glucosidase by Brazilian medicinal plant extracts.
Table 2. Enzymatic inhibition (%) and compounds annotated by UHPLC-ESI-MS/MS in L. origanoides from Manaus (LOM), L. origanoides from Videiras Valley (LOVV) extracts and in fraction F08, in the positive mode.
Table 3. Enzymatic inhibition (%) and compounds annotated by UHPLC-ESI-MS/MS in Hyptis monticola extract and fractions, in the positive mode.
Bioassay-guided fractionation was carried out on those extracts with sufficient mass to enable it, namely the extract from L. origanoides of Videiras Valley (LOVV) and that from H. monticola. The fractionations were performed by HSCCC, which is a support-free chromatographic technique, suitable for biological screening since there is no loss of compounds or bioactivity due to interactions between the solid phase and the target compounds. Thus, the integrity of the analytes is better maintainedCitation36.
From UHPLC-ESI-MS/MS analysis, the chemical composition of the two extracts from the leaves of L. origanoides resulted slightly different (). The extract from Manaus (LOM) showed more abundant presence of C-glycosyl flavones. Isoorientin and isovitexin, possessing the highest relative percentage, were not detected in the leaves extract from Videiras Valley (LOVV). Both compounds were successfully tested against α-glucosidase enzyme, resulting potent inhibitorsCitation37. Vicenin 2 was detected in both extracts. This C-glycosyl flavone was proved to be a multitarget agent for treatment of diabetes and relative complications. In addition to inhibiting the α-glucosidase enzyme, it resulted active against the rat lens aldose reductase and the protein tyrosine phosphatase, two enzymes involved in the modulation of insulin sensitivity and in the conversion of glucose into sorbitol, respectivelyCitation38. Moreover, its interaction against the enzyme was described by a docking study, where vicenin 2 showed a docking score lower than isoorientin and isovitexinCitation39. Some compounds detected in LOVV were not found in LOM. These metabolites were pinocembrin, sakuranetin, genkwanin, aromadendrin, and homoeriodictyol. Considering the relative percentages of the detected compounds, the LOVV extract resulted mostly composed by sakuranetin, vicenin 2, naringenin, and pinocembrin. Among these, vicenin 2, naringenin, and pinocembrin are promising α-glucosidase inhibitorsCitation40,Citation41, while sakuranetin has been found to possess only a moderate activity against the enzymeCitation42. These differences in the phytochemical profiles of the two extracts, which resulted in a different bioactivity, are certainly due to the different environment where the plants grew. Altitude, solar irradiation, supply of water and soil type strongly influence the production of flavonoids and the activation of certain biosynthetic pathways rather than othersCitation43,Citation44. L. origanoides (LOVV) extract was fractioned into nine fractions which were tested at the concentration of 100 µg/mL (Table S3), that is close to the IC50 of the extract (94.24 ± 2.1 µg/mL). Considering this, only the fractions with inhibition greater than 60% were considered for the calculation of IC50, namely F05 and F08. The most active fraction F08, showing an IC50 value of 15.9 μg/mL, was also dereplicated by UHPLC-ESI-MS/MS analysis (). This fraction resulted mostly composed by vicenin 2 and naringenin, while sakuranetin was detected in low quantity. Fraction F05 was predominantly composed by pinocembrin, which was previously isolated from the same plant material as described by Leitão et al.Citation31, thus, this isolated compound was used as standard for the identification.
Considering that the C-glycosyl flavones, as vicenin 2, are widely renowned for their antidiabetic propertiesCitation45, so much so that the activity of LOM extract could be attributed to them, we decided to better investigate the activity of flavonoid aglycones, to which a significant part of LOVV's activity can be referred.
Hence, the IC50 of the compounds were calculated, resulting in 70 ± 2.1 μM for naringenin and 156 ± 1.3 μM for pinocembrin. Taking into account that the IC50 of acarbose was of 105 μM, both flavonoids reached remarkably enzymatic inhibition, especially naringenin which demonstrated stronger anti-α-glucosidase activity than the positive control. In face of these results, the enzymatic inhibition mechanism of both flavonoids was investigated. Hence, the L–B plots were built by following the kinetics of α-glucosidase in the absence and in the presence of the compounds, fitting the inhibition data of three independent experiments with samples in duplicate (Table S5). The mechanism of inhibition was determined by comparing through statistical analysis the KM and Vmax of 1 mU of enzyme reacting with different amount of substrate under the experimental conditions of the assay, with those of 1 mU of enzyme reacting with the same amount of substrate, under the same conditions, adding the bioactive compounds at the IC50 concentration. As shown in , treating the enzyme with naringenin the Vmax value remained unchanged, while KM was altered, resulting higher. This occurs when a competitive inhibitory mechanism is involved. For pinocembrin, both kinetic parameters resulted altered, specifically KM was higher and Vmax lower than those of the enzyme reacting only with the substrate. These values indicated the involvement of a mixed-type inhibition mechanism (). The inhibitory activity of naringenin against α-glucosidase enzyme has already been reported, and our results (70 µM) agree with the published IC50 (75 µM) Citation41. Priscilla et al.Citation46 investigated the inhibitory mechanism of naringenin, indicating a competitive mode of inhibition against mammalian-α-glucosidase, such as in our experiment. Recently, Potipiranun et al.Citation40 reported the α-glucosidase inhibitory activity of pinocembrin, they also defined the mechanism of action, reporting a mixed type of inhibition for this compound, as in our results.
Figure 2. The Lineweaver–Burk plot of α-glucosidase and p-NPG without naringenin (negative control) and in presence of naringenin at IC50 concentration (NARINGENIN).

Figure 3. The Lineweaver–Burk plot of α-glucosidase and p-NPG without pinocembrin (negative control) and with pinocembrin at IC50 concentration (PINOCEMBRIN).

Considering the prominent presence of secondary metabolites with anti-α-glucosidase activity, and the importance of L. origanoides as a Brazilian medicinal plant and spice (oregano-like), its leaves extract proved to be particularly suitable for more in-depth investigations to develop nutraceutical formulations against the type 2 diabetes disease. However, this study points out that the same plant harvested in different environments may have a different expression of secondary metabolites, resulting in a different bioactivity.
The extract from H. monticola was fractioned into 22 fractions which were tested against α-glucosidase enzyme (Tables S3 and S4). None of the fractions showed a lower IC50 than the extract, meaning that a synergistic effect may be acting, or the extract may also contain hydrolysable tannins responsible for part of the activity, that is lost during the fractionation. Tannins may cause interference in the assay, leading to an overestimation of the enzymatic inhibition due to the non-specific enzyme complexationCitation47–49. However, the IC50s values of the fractions were still considerable. From the UPLC–MS analysis, several compounds with known α-glucosidase inhibitory activity were identified. Precisely, the flavonoids rutin, hyperoside, quercetin, isoquercetin, luteolin, and kaempferol are well-characterised inhibitors acting through different mechanisms which lead to a substantial reduction in the activity of the enzymeCitation33,Citation50–54. Rutin, hyperoside and isoquercetin were identified in fractions with inhibition values lower than 60%. Conversely, the most active fractions were the ones containing quercetin, quercetin isomers, luteolin, and aromadendrin.
4. Conclusions
In the screening of 15 extracts from Brazilian medicinal plants, four extracts emerged as promising inhibitors of the α-glucosidase enzyme. These extracts were obtained from the flowers of Hyptis monticola and the leaves of Lantana trifolia and Lippia origanoides harvested in two different areas. The three most active extracts, from H. monticola and L. origanoides, were analysed by UHPLC–MS/MS. Comparing the two L. origanoides leaves extracts, the sample from the Amazon region resulted enriched in C-glycosyl flavones. Among these, isoorientin and isovitexin, two potent inhibitors, were not found in the sample from the Atlantic Forest. Conversely, some metabolites of the extract LOVV were not detected in LOM. These include pinocembrin and sakuranetin, which were proved to possess good and moderate activity against the enzyme, respectively. The differences in the chemical profiles could explain the different bioactivity of the extracts. The bioassay-guided fractionation of LOVV extract led to the identification of the bioactive compounds, namely vicenin 2, naringenin, and pinocembrin. Aiming at assessing the activity of the flavonoid aglycones, more highly expressed in LOVV than in the LOM extract, their IC50 was calculated, and the mechanism of enzyme inhibition determined. Naringenin showed an IC50 of 70 ± 2.1 μM and the mechanism of enzyme inhibition resulted of competitive type. The IC50 of pinocembrin was of 156 ± 1.3 μM and the inhibition resulted of mixed type. These data agree with those found in literature. The fractionation of the extract from H. monticola caused a loss of activity, no fractions yielded IC50s values lower than the extract. This could be the consequence of a synergistic effect of metabolites or could be caused by the presence of a tannin component which are interfering in this type of assay. Overall, the analysed extracts were found to possess several compounds with anti-α-glucosidase activity, particularly the leaves extract from L. origanoides. Hence, they could be considered as natural sources of antidiabetic agents and they could be evaluated for the development of nutraceutical formulations for the treatment of type 2 diabetes.
Supplemental Material
Download PDF (228.8 KB)Acknowledgements
We are indebted to the Laboratório Multiusuário de Análises por RMN at the Federal University of Rio de Janeiro for the access to NMR facilities, and to the Central Analítica, Departamento de Produtos Naturais e Alimentos for the MS analyses.
Disclosure statement
All the authors declare that there is no conflict of interest concerning this work.
Additional information
Funding
References
- Kimura A, Lee JH, Lee IS, et al. Two potent competitive inhibitors discriminating alpha-glucosidase family I from family II. Carbohydr Res 2004;339:1035–40.
- Lebovitz HE. Alpha-glucosidase inhibitors. Endocrinol Metab Clin North Am 1997;26:539–51.
- Scheen AJ. Is there a role for α-glucosidase inhibitors in the prevention of type 2 diabetes mellitus? Drugs 2003;63:933–51.
- Baron AD. Postprandial hyperglycaemia and α-glucosidase inhibitors. Diabetes Res Clin Pract 1998;40:S51–S5.
- Kumar S, Narwal S, Kumar V, Prakash O. α-Glucosidase inhibitors from plants: a natural approach to treat diabetes. Pharmacogn Rev 2011;5:19–29.
- Myers N, Mittermeier RA, Mittermeier CG, et al. Biodiversity hotspots for conservation priorities. Nature 2000;403:853–8.
- Mittermeier RA, Robles Gil P, Hoffmann M, et al., editors. Hotspots revisited: earth's biologically richest and most endangered terrestrial ecoregions; revised edition. Mexico City: Conservation International; 2005:392.
- Tabarelli M, Pinto LP, Silva JM, et al. Challenges and opportunities for biodiversity conservation in the Brazilian Atlantic Forest. Conserv Biol 2005;19:695–700.
- Cardoso D, Särkinen T, Alexander S, et al. Amazon plant diversity revealed by a taxonomically verified species list. Proc Natl Acad Sci USA 2017;114:10695–700.
- Ribeiro MC, Metzger JP, Martensen AC, et al. The Brazilian Atlantic Forest: how much is left, and how is the remaining forest distributed? Implications for conservation. Biol Conserv 2009;142:1141–53.
- Arika WM, Abdirahman YA, Mawia MM, et al. Hypoglycemic effect of Lippia javanica in alloxan induced diabetic mice. J Diabetes Metab 2015;6:624–30.
- Pascual ME, Slowing K, Carretero E, et al. Lippia: traditional uses, chemistry and pharmacology: a review. J Ethnopharmacol 2001;76:201–14.
- Maroyi A. Lippia scaberrima Sond. (Verbenaceae): traditional uses, phytochemical and pharmacological properties. J Pharm Sci 2019;11:3685–90.
- El-Ouady F, Eddouks M. Antihyperglycemic and antihyperlipidemic effects of Lippia citriodora in rats. Endocr Metab Immune Disord Drug Targets 2021;21:711–9.
- Al-Snai AE. Pharmacological and therapeutic effects of Lippia nodiflora (Phyla nodiflora). IOSR J Pharm 2019;9:15–25.
- Ngugi MP, Kimuni NS, Ngeranwa NJ, et al. Antidiabetic and safety of Lantana rhodesiensis in alloxan induced diabetic rats. J Dev Drugs 2015;4:129.
- Venkatachalam T, Kumar VK, Selvi PK, et al. Antidiabetic activity of Lantana camara Linn fruits in normal and streptozotocin-induced diabetic rats. J Pharm Res 2011;4:1550–2.
- Kumar KV, Sharief SD, Rajkumar R, et al. Antidiabetic potential of Lantana aculeata root extract in alloxan-induced diabetic rats. Int J Phytomed 2010;2:299–303.
- Nayak S. Evaluation of antidiabetic and antioxidant activity of aerial parts of Hyptis suaveolens Poit. Afr J Pharm Pharmacol 2013;7:1–7.
- Ogar I, Egbung GE, Nna VU, et al. Anti-hyperglycemic potential of Hyptis verticillata Jacq in streptozotocin-induced diabetic rats. Biomed Pharmacother 2018;107:1268–76.
- Hernandez-Galicia E, Aguilar-Contreras A, Aguilar-Santamaria L, et al. Studies on hypoglycemic activity of Mexican medicinal plants. Proc West Pharmacol Soc 2002;45:118–24.
- Esquivel-Gutiérrez ER, Noriega-Cisneros R, Saavedra-Molina A, Salgado-Garciglia R. Plants used in Mexican folk medicine with antidiabetic and antihypertensive properties. Pharmacol Online 2013;2:15–23.
- Gandhi GR, Ignacimuthu S, Paulraj MG. Solanum torvum Swartz. fruit containing phenolic compounds shows antidiabetic and antioxidant effects in streptozotocin induced diabetic rats. Food Chem Toxicol 2011;49:2725–33.
- Sugunabai J, Jayaraj M, Karpagam T, Varalakshmi B. Antidiabetic efficiency of Moringa oleifera and Solanum nigrum. Int J Pharm Pharm Sci 2014;6:40–2.
- Doss A, Palaniswamy M, Angayarkanni J, Dhanabalan R. Antidiabetic activity of water extract of Solanum trilobatum (Linn.) in alloxan-induced diabetes in rats. Afr J Biotechnol 2009;8:5562–4.
- Kandimalla R, Kalita S, Choudhury B, Kotoky J. A review on anti-diabetic potential of genus Solanum (Solanaceae). J Drug Deliv Ther 2015;5:24–7.
- Bouslimani A, Sanchez LM, Garg N, Dorrestein PC. Mass spectrometry of natural products: current, emerging and future technologies. Nat Prod Rep 2014;31:718–29.
- Martínez-Fructuoso L, Pereda-Miranda R, Rosas-Ramírez D, et al. Structure elucidation, conformation, and configuration of cytotoxic 6-heptyl-5,6-dihydro-2H-pyran-2-ones from Hyptis species and their molecular docking to α-tubulin. J Nat Prod 2019;82:520–31.
- Martins GR, Fonseca TS, Martínez-Fructuoso L, et al. Antifungal phenylpropanoid glycosides from Lippia rubella. J Nat Prod 2019;82:566–72.
- Castellar A, Coelho TS, Silva PEA, et al. The activity of flavones and oleanolic acid from Lippia lacunosa against susceptible and resistant Mycobacterium tuberculosis strains. Rev Bras Farmacogn 2011;21:835–40.
- Leitão SG, Leitão GG, Vicco DKT, et al. Counter-current chromatography with off-line detection by ultra high performance liquid chromatography/high resolution mass spectrometry in the study of the phenolic profile of Lippia origanoides. J Chromatogr A 2017;1520:83–90.
- Julião LS, Piccinelli AL, Leitão SG, et al. Phenylethanoid glycosides from Lantana fucata Lindl. with in vitro antiinflammatory activity. J Nat Prod 2009;72:1424–8.
- Julião LS, Leitão SG, Lotti C, et al. Flavones and phenylpropanoids from a sedative extract of Lantana trifolia L. Phytochemistry 2010;71:294–300.
- Leitão F, Moreira DL, Almeida MZ, Leitão SG. Secondary metabolites from the mistletoes Struthanthus marginatus and S. concinnus (Loranthaceae). Biochem Syst Ecol 2013;48:215–8.
- Venditti A, Maggi F, Vittori S, et al. Antioxidant and α-glucosidase inhibitory activities of Achillea tenorii. Pharm Biol 2015;53:1505–10.
- Leitão GG, Eladji SS, Melo WAL, Leitao SG, Brown L. Separation of free and glycosylated flavonoids from Siparuna guianensis by gradient and isocratic CCC. J Liq Chromatogr R T 2005;28(12/13):2041–2051.
- Costa GM, Cárdenas PA, Gazola AC, Aragón DM, Castellanos L, Reginatto FH, Schenkel EP. Isolation of C-glycosylflavonoids with α-glucosidase inhibitory activity from Passiflora bogotensis Benth by gradient high-speed counter-current chromatography. J Chromatogr B 2015;990:104–110.
- Islam MN, Ishita IJ, Jung HA, Choi JS. Vicenin 2 isolated from Artemisia capillaris exhibited potent anti-glycation properties. Food Chem Toxicol 2014;69:55–62.
- Anjitha R, Subbaraya U, Narasimhan, G. Integrated In vitro-In silico screening strategy of Ethanolic extract of leaves of Passiflora edulis Sims var. flavicarpa against Alpha Glucosidase. Res J Pharm Tech 2020; 13(3):1309–1313.
- Potipiranun T, Adisakwattana S, Worawalai W, Ramadhan R, Phuwapraisirisan P. Identification of Pinocembrin as an Anti-Glycation Agent and α-Glucosidase Inhibitor from Fingerroot (Boesenbergia rotunda): The Tentative Structure–Activity Relationship towards MG-Trapping Activity. Molecules 2018;23(12):3365.
- Tadera K, Minami Y, Takamatsu K, Matsuoka T. Inhibition of α-glucosidase and α-amylase by flavonoids. J Nutr Sci Vitaminol 2006;52(2):149–153.
- Amador S, Nieto-Camacho A, Ramírez-Apan MT, Martínez M, Maldonado E. Cytotoxic, anti-inflammatory, and α-glucosidase inhibitory effects of flavonoids from Lippia graveolens (Mexican oregano). Med Chem Res 2020;29:1497–1506.
- Jaakola L, Hohtola A. Effect of latitude on flavonoid biosynthesis in plants. Plant Cell Environ 2010;33(8):1239–1247.
- Giupponi L, Leoni V, Pavlovic R, Giorgi A. Influence of altitude on phytochemical composition of hemp inflorescence: A metabolomic approach. Molecules, 2020;25(6):1381.
- Chua LS, Abdullah FI, Awang MA. Potential of natural bioactive C-glycosyl flavones for antidiabetic properties. In Stud Nat Prod Chem 2020;64:241–261.
- Priscilla DH, Roy D, Suresh A, Kumar V, Thirumurugan K. Naringenin inhibits α-glucosidase activity: A promising strategy for the regulation of postprandial hyperglycemia in high fat diet fed streptozotocin induced diabetic rats. Chem Biol Interact 2014;210:77–85.
- Setyaningsih EP, Saputri FC, Mun'im A. The Antidiabetic Effectivity of Indonesian Plants Extracts via DPP-IV Inhibitory Mechanism. J Young Pharm 2019;11(2).
- Adamczyk B, Simon J, Kitunen V, Adamczyk S, Smolander A. Tannins and their complex interaction with different organic nitrogen compounds and enzymes: old paradigms versus recent advances. ChemistryOpen 2017;6(5):610–614.
- Wong AIC, Huang D. Assessment of the degree of interference of polyphenolic compounds on glucose oxidation/peroxidase assay. J Agr Food Chem 2014;62(20):4571–4576.
- Dubey S, Ganeshpurkar A, Ganeshpurkar A, Bansal D, Dubey N. Glycolytic enzyme inhibitory and antiglycation potential of rutin. Future J Pharm Sci 2017;3(2):158–162.
- Islam MN, Jung HA, Sohn HS, Kim H M, Choi J S. Potent α-glucosidase and protein tyrosine phosphatase 1B inhibitors from Artemisia capillaris. Archiv Pharm Res 2013;36(5):542–552.
- Flores-Bocanegra L, Pérez-Vásquez A, Torres-Piedra M, Bye R, Linares E, Mata R. α-Glucosidase inhibitors from Vauquelinia corymbosa. Molecules 2015;20(8):15330–15342.
- Li YQ, Zhou FC, Gao F, Bian JS, Shan F. Comparative evaluation of quercetin, isoquercetin and rutin as inhibitors of α-glucosidase. J Agric Food Chem 2009;57(24):11463–11468.
- Cai Y, Wu L, Lin X, Hu X, Wang L. Phenolic profiles and screening of potential α-glucosidase inhibitors from Polygonum aviculare L. leaves using ultra-filtration combined with HPLC-ESI-qTOF-MS/MS and molecular docking analysis. Ind Crops Prod 2020;154:112673.
- Tang G, Liu X, Gong X, Lin X, Lai X, Wang D, Ji S. Studies on the chemical compositions of Hyptis suaveolens (L.) Poit. J Serb Chem 2019;84(3):245–252.
- Abedini A, Roumy V, Mahieux S, Biabiany M, Standaert-Vitse A, Rivière C, Hennebelle T. Rosmarinic acid and its methyl ester as antimicrobial components of the hydromethanolic extract of Hyptis atrorubens Poit. (Lamiaceae). Evid Based Complement Alternat Med 2013;2013.