Abstract
To discover new agrochemicals with prominent pesticidal properties, a series of novel β-naphthol derivatives containing benzothiazolylamino and various heteroaryl groups (8a-q) were efficiently synthesised via Betti reaction. The bioassay results showed that most of the synthesised compounds exhibited favourable insecticidal potentials, particularly towards oriental armyworm (50–100% at 200 mg·L−1) and diamondback moth (50–95% at 10 mg·L−1). Compounds 8 b, 8f, 8 g, 8j, 8k, 8n, and 8o possessed LC50 values of 0.0988–5.8864 mg·L−1 against diamondback moth. Compounds 8i, 8 l, and 8 m also displayed lethality rates of 30–90% against spider mite at the concentration of 100 mg·L−1. Overall, some compounds could be considered as new insecticidal/acaricidal leading structures for further investigation. The calcium imaging experiments revealed that 8 h, 8i, and viii could activate the release of calcium ions in insect (M. separata) central neurons at a higher concentration (50 mg·L−1). The SAR analysis provided valuable information for further structural modifications.
Graphical abstract
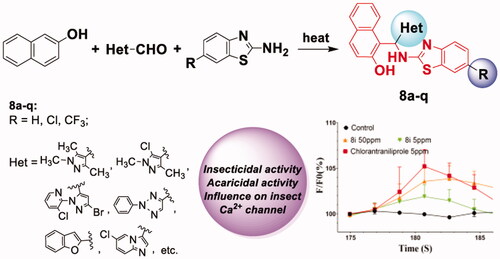
1. Introduction
Agrochemicals exert a vital role in guaranteeing the crop production of the world. According to the FAO of the United Nations, even with the application of agrochemicals, about 30% of the world potential crop production is still lost every year due to the influences of all kinds of crop pests, diseases and weedsCitation1. But what is more striking is that these losses of crop production will be possibly doubled if there are no existing agrochemicals used. However, during the use of agrochemicals, some negative effects, such as eco-environmental pollution and resistance, have always been accompanied. Due to the dominance of such issues, the discovery and development of more novel agrochemicals with prominent pesticidal properties are urgently neededCitation2–4.
The great potential of phenolic compounds in medicinal and pesticidal chemistry has been highly recognised. Many compounds containing phenol motifs have been found to possess a variety of pharmacological properties, such as antioxidantCitation5,Citation6, anti-inflammatoryCitation6, anticancerCitation7, antiproliferativeCitation8, antitumorCitation8, cytotoxicCitation9 and antibacterialCitation9 activities. Moreover, many phenols are important intermediates for producing commercial agrochemicals. Some phenolic derivatives also exhibit promising pesticidal activities. For examples, DNOC (, i) can be used as a herbicidal and a fungicidal agentCitation10,Citation11; eugenol (, ii) is a natural acaricide and fungicideCitation12,Citation13; dichlorophen and carvacrol (, iii and iv) have effective fungicidal activitiesCitation14,Citation15; thymol (, v) was recently reported to have promising insecticidal activity against Caenorhabditis elegans at different stages of growth and developmentCitation16. Comparatively, there are seldom reports about a naphthol moiety being a part of the potent insecticidal agents, except those several representative natural products, such as methoxyhemigossypol and gossypol (, vi and vii), both of which have insecticidal and antifungal activitiesCitation17–19.
Figure 1. Chemical structures of some natural and synthesised phenolic derivatives possessing pesticidal activities.

Recently, some β-naphthol derivatives containing benzothiazolylamino and substituted phenyl groups (e. g. compound viii in ) drew our attention, because of their favourable antifeedant and insecticidal activities against Spodoptera litura and Achaea janata (L.)Citation20. Compounds viii and ix () have also been found to have inhibitory activity against angiotensin-converting-enzyme as well as calcium channel blocking activity on mammal rats, as reported by Kalavagunta et al.Citation21. Whereas, both - the exploration of pesticidal potentials of more new β-naphthol derivatives and the influence of the compounds on the activity of insect calcium channels - remain uncovered. It has been known that the ryanodine receptor (RyR), which can modulate the release of calcium ions in the insect neuronal cells, is one of the most important targets for the insecticide design during the recent 15 years. Several diamide compounds with potent RyR activating effect, e.g., flubendiamide and chlorantroniliprole, have been discovered and successfully developed as commercial insecticides owing to their excellent pesticidal propertiesCitation22,Citation23. In addition, it has also been known that heterocyclic compounds comprise a large proportion of pharmaceuticals and agrochemicals due to their versatile biological activitiesCitation24,Citation25. A common strategy in molecular design is that heterocyclic moieties are introduced as substitutions of phenyl rings to improve the biological properties of the parent compounds. Based on this information, a series of novel benzothiazolylamino-containing β-naphthol derivatives were designed and successfully synthesised by introduction of various heteroaryl groups. Their insecticidal and acaricidal activities towards a variety of agricultural pests were evaluated. In addition, the influence of this type of compounds on the insect calcium ion channels (RyR) was also investigated.
2. Experimental
2.1. Chemistry
The melting points were determined on an X-4 binocular microscope apparatus (uncorrected). 1H NMR spectra were determined at 400 MHz and 13 C NMR spectra at 101 MHz using a Bruker AV 400 instrument with CDCl3 or DMSO-d6 as solvents, and tetramethylsilane as the internal standard. HRMS were determined on a Varian 7.0 T FTICR-MS instrument. The purity analysis of the synthesised compounds were conducted on an Agilent 1260-II high performance liquid chromatography (HPLC) apparatus. Column chromatography was conducted using 200–300 mesh silica gel. Reagents were of analytically pure grade. Solvents were dried and distilled using the standard procedure before use. Benzo[d]thiazol-2-amine, 6-chlorobenzo[d]thiazol-2-amine and 6-(trifluoromethyl)benzo[d]thiazol-2-amine were purchased from J&K Scientific Ltd. Naphthalen-2-ol and partial heteroaryl aldehydes were bought from Tianjin Heowns Business Licence and Bide Pharmatech Ltd. As the positive control for biological tests, chlorantraniliprole was prepared following the known literature procedureCitation26,Citation27. Another two bioassay controls that have the similar structural characteristic as β-naphthol − 1-((benzo[d]thiazol-2-ylamino)(4-methoxyphenyl)methyl)naphthalen-2-ol (viii) and 1-((benzo[d]thiazol-2-ylamino)(4-chlorophenyl)methyl)naphthalen-2-ol (ix), were prepared via the reported literature protocolCitation20.
2.1.1. Synthesis of the intermediate heteroaryl aldehydes 2a-d
2.1.1.1. Synthesis of 5-chloro-1,3-disubstituted-1H-pyrazole-4-carbaldehydes 2a-b
According to the reported procedure, 2,5-disubstitued-2,4-dihydro-3H-pyrazol-3-ones 1a-b were prepared via a cyclisation reaction of ethyl acetoacetate or ethyl trifluoroacetoacetate in the presence of methylhydrazine or phenylhydrazine in boiling ethanolCitation28,Citation29. 1a: white solid, yield 69%, mp 121–122 °C (Lit.Citation29 mp 122 °C). 1H NMR (400 MHz, CDCl3) δ: 3.27 (s, 3H), 3.18 (s, 2H), 2.10 (s, 3H); 1 b: white solid, yield 75%, mp 191–193 °C (Lit.Citation30 mp 193.0–193.5 °C). 1H NMR (400 MHz, CDCl3) δ: 7.80 (d, J = 8.0 Hz, 2H), 7.68 (d, J = 8.0 Hz, 2H), 7.42–7.49 (m, 5H), 7.37–7.39 (m, 1H), 5.90 (s, 1H), 3.71 (s, 2H). The product was obtained as a mixture of dihydropyrazolone and pyrazolol.
Using an improved method from the previous reportCitation31,Citation32, various pyrazole aldehyde 2a-b were synthesised smoothly. Phosphorus oxychloride (64 ml) was added dropwise to 23 ml of DMF at 0–10 °C under stirring. Thirty minutes later at this temperature, pyrazolone 1 (0.1 mol) was added in portions. The reaction system was then heated to 80–100 °C and stirred for 3–4 h. After cooling down to ambient temperature, the system was poured into ice-water (380 ml) and further stirred for 15 min, then extracted with ethyl acetate (3 × 20 ml). The organic phase was washed with saturated aq. solution of NaHCO3 (2 × 20 ml) and brine (2 × 20 ml) successively, and dried over anhydrous Na2SO4. After removal of solvent, the residue was recrystallized from petroleum ether and ethyl acetate or subjected for the silica gel column chromatography using ethyl acetate and petroleum ether as solvents (v/v 1:4) to afford pyrazole aldehydes 2a-b. 2a: white solid, Rf 0.76 (petroleum ether/ethyl acetate 3:1), mp 77–78 °C, yield 82% (Lit.Citation31 mp 78–79 °C), 1H NMR (400 MHz, CDCl3) δ: 9.86 (s, 1H), 3.83 (s, 3H), 2.46 (s, 3H); 2 b: yellow sticky oil (it can slowly solidify upon prolonged storage), mp 32–34 °C, Rf 0.73 (petroleum ether/ethyl acetate 3:1), yield 91% (Lit.Citation33 mp 34–36 °C), 1H NMR (400 MHz, CDCl3) δ: 10.06 (s, 1H), 5.56–5.57 (m, 5H).
2.1.1.2. Synthesis of 3-bromo-1-(3-chloropyridin-2-yl)-1H-pyrazole-5-carbaldehyde 2c
A 100 ml flask charged with a solution of pyrazole carboxylic ester 3 (0.66 g, 2.0 mmol) in THF (30 ml) was cooled to −5 °C by an ice-salt bath. Lithium aluminium hydride (0.076 g, 2.0 mmol) was added to the above solution in small portions maintaining the temperature of −5–5 °C. After that, the reaction system was stirred at room temperature overnight followed by addition of excess of Na2SO4·10H2O (10.0 g) to quench the reaction. The mixture was filtered and the filtrate was concentrated under reduced pressure. The residue was further purified via silica gel column chromatography using ethyl acetate and petroleum ether as solvents (v/v 1:4) to give intermediate alcohol 4 as a white solid with 72% yield and mp of 108–109 °C (lit.Citation34 mp 109–111 °C). Rf 0.30 (petroleum ether/ethyl acetate 3:1), 1H NMR (400 MHz, DMSO-d6) δ: 8.56 (d, J = 5.9 Hz, 1H), 8.26 (d, J = 8.1 Hz, 1H), 7.65 (dd, J = 8.1, 5.9 Hz, 1H), 6.58 (s, 1H), 5.36 (t, J = 5.8 Hz, 1H), 4.45 (d, J = 5.8 Hz, 2H).
The pyrazole aldehyde 2c was prepared using an improved method based on the previous reportCitation34. To a solution of intermediate alcohol 4 (2.7 g, 9.4 mmol) in DCM (50 ml), a well-mixed powder of pyridinium chlorochromate (6.0 g, 28.1 mmol) and silica gel (200–300 mesh, 9.0 g) was added at room temperature. The reaction mixture was stirred overnight and the solvent was removed under reduced pressure. The residue was purified by column chromatography using ethyl acetate and petroleum ether as solvents (v/v 1:6) to afford pyrazole aldehyde 2c as light yellow solid with 95% yield and mp of 103–104 °C (lit.Citation34 mp 104–105 °C). Rf 0.76 (petroleum ether/ethyl acetate 3:1), 1H NMR (400 MHz, DMSO-d6) δ: 9.80 (s, 1H), 8.58 (dd, J = 4.7, 1.4 Hz, 1H), 8.31 (dd, J = 8.1, 1.4 Hz, 1H), 7.73 (dd, J = 8.1, 4.7 Hz, 1H), 7.54 (s, 1H).
2.1.1.3. Synthesis of 2-phenyl-2H-1,2,3-triazole-4-carbaldehyde 2d
A mixture of the intermediate 1-(2-phenyl-2H-1,2,3-triazol-4-yl)butane-1,2,3,4-tetraol (5) (8.0 g, 0.03 mol), sodium periodate (19.26 g, 0.09 mol) and water (250 ml) was stirred at room temperature for 24 h, then filtered. The solid was washed with water thoroughly, dried in air and recrystallized from petroleum ether to afford phenyltriazole carbaldehyde intermediate 2d as light yellow crystals with 69% yield and mp of 66–67 °C (Lit.Citation35 mp 68–69 °C). 1H NMR (400 MHz, CDCl3) δ: 10.22 (s, 1H), 8.27 (s, 1H), 8.14 (d, J = 8.0 Hz, 2H), 7.54 (t, J = 8.0 Hz, 2H), 7.45 (t, J = 8.0 Hz, 1H).
2.1.2. Synthesis of the title compounds 8a-q
A 50 ml round-bottom flask equipped with a condenser and containing a well-mixed powder of naphthalen-2-ol (6, 1 mmol), 6-substitutedbenzo[d]thiazol-2-amine (7, 1 mmol) and heteroaryl aldehyde (2, 1.1 mmol) was put into a 115–125 °C oil-bath under stirring. The mixture was maintained at this temperature for 1–2 h. Methanol (15 ml) was added to the mixture when the temperature of oil-bath was decreased to 80 °C. The mixture was further stirred for 10 min. After cooling down to room temperature, the mixture was filtered and the residue was washed successively or submitted for silica gel column chromatography using ethyl acetate and petroleum ether (v/v 1:7) as solvents to afford compound 8(a-q).
2.1.2.1. 1-((Benzo[d]thiazol-2-ylamino)(1H-imidazol-2-yl)methyl)naphthalen-2-ol (8a)
White solid, yield 62%, Rf 0.64 (dichloromethane/methanol 100:1), mp 277–280 °C. 1H NMR (400 MHz, DMSO-d6) δ: 9.97 (s, 1H), 9.02 (d, J = 8.4 Hz, 1H), 8.63 (s, 1H), 8.04 (d, J = 8.5 Hz, 1H), 7.84–7.94 (m, 2H), 7.74–7.82 (m, 2H), 7.66 (d, J = 8.7 Hz, 1H), 7.35–7.53 (m, 3H), 7.29 (t, J = 7.2 Hz, 1H), 7.08–7.16 (m, 1H), 6.85 (d, J = 8.8 Hz, 1H), 6.74 (s, 1H); 13 C NMR (101 MHz, DMSO-d6) δ 164.9, 159.4, 155.8, 155.0, 148.0, 132.2, 131.1, 130.0, 129.2, 128.6, 126.9, 126.5, 124.5, 123.8, 123.5, 122.5, 119.6, 118.6, 117.0, 115.1, 48.6. HRMS (ESI): calcd for C21H17N4OS [M + H]+ 373.1123, found, 373.1116, the error 1.88 ppm.
2.1.2.2. 1-((Benzo[d]thiazol-2-ylamino)(1H-pyrazol-5-yl)methyl)naphthalen-2-ol (8 b)
White solid, yield 67%, Rf 0.63 (dichloromethane/methanol 100:1), mp 190–193 °C. 1H NMR (400 MHz, DMSO-d6) δ: 10.07 (s, 1H), 9.00 (d, J = 8.6 Hz, 1H), 8.02 (d, J = 8.5 Hz, 1H), 7.91 (d, J = 8.9 Hz, 1H), 7.82–7.87 (m, 2H), 7.79 (d, J = 7.1 Hz, 2H), 7.67 (d, J = 8.8 Hz, 1H), 7.55 (d, J = 7.8 Hz, 1H), 7.45 (d, J = 8.9 Hz, 1H), 7.40 (d, J = 7.3 Hz, 1H), 7.30 (d, J = 7.5 Hz, 1H), 7.09–7.15 (m, 1H), 6.89 (d, J = 8.8 Hz, 1H), 6.71 (s, 1H); 13 C NMR (101 MHz, DMSO-d6) δ 166.4, 160.7, 155.5, 148.2, 132.6, 131.4, 130.7, 129.9, 129.3, 129.1, 128.0, 127.5, 127.1, 125.2, 124.0, 123.1, 119.8, 119.1, 117.3, 114.9, 31.5. HRMS (ESI): calcd for C21H17N4OS [M + H]+ 373.1123, found, 373.1112, the error 2.95 ppm.
2.1.2.3. 1-((Benzo[d]thiazol-2-ylamino)(1,3,5-trimethyl-1H-pyrazol-4-yl)methyl)naphthalen-2-ol (8c)
White solid, yield 57%, Rf 0.67 (dichloromethane/methanol 100:1), mp 179–181 °C. 1H NMR (400 MHz, DMSO-d6) δ: 9.97 (br, 1H), 8.60 (d, J = 6.4 Hz, 1H), 8.02 (d, J = 8.6 Hz, 1H), 7.74–7.79 (m, 3H), 7.68 (d, J = 8.2 Hz, 1H), 7.63 (d, J = 7.7 Hz, 1H), 7.40 (d, J = 7.1 Hz, 1H), 7.25–7.28 (m, 1H), 7.21 (d, J = 8.8 Hz, 1H), 7.12 (s, 1H), 6.98–7.11 (m, 1H), 3.56 (s, 3H), 1.93 (s, 3H), 1.87 (s, 3H); 13 C NMR (101 MHz, DMSO-d6) δ: 165.9, 155.7, 153.5, 152.9, 144.2, 136.3, 135.1, 132.9, 131.0, 129.7, 128.1, 126.5, 125.8, 123.1, 122.8, 121.3, 118.9, 118.7, 115.8, 109.1, 48.3, 36.0, 13.1, 9.9. HRMS (ESI) calcd for C24H23N4OS [M + H]+ 415.1593, found 415.1584, the error 2.17 ppm.
2.1.2.4. 1-((Benzo[d]thiazol-2-ylamino)(1-methyl-3-(trifluoromethyl)-1H-pyrazol-4-yl)methyl)naphthalen-2-ol (8d)
White solid, yield 68%, Rf 0.65 (dichloromethane/methanol 100:1), mp 184–186 °C. 1H NMR (400 MHz, CDCl3) δ: 12.44 (br, 1H), 8.03 (d, J = 8.4 Hz, 1H), 7.79 (d, J = 8.0 Hz, 1H), 7.75 (d, J = 8.8 Hz, 1H), 7.49–7.60 (m, 3H), 7.47 (s, 1H), 7.27–7.37 (m, 3H), 7.11 (t, J = 7.6 Hz, 1H), 6.63 (s, 1H), 3.72 (s, 3H); 13 C NMR (101 MHz, CDCl3) δ: 169.3, 153.7, 150.0, 138.9 (q, J = 36.36 Hz, Pyrazole), 132.8, 131.9, 130.6, 129.5, 129.0, 128.7, 127.4, 126.3, 123.3, 122.2, 121.8 (q, J = 269.67 Hz, CF3), 121.5, 119.7, 119.0, 117.7, 117.3, 110.0, 50.0, 39.6. HRMS (ESI) calcd for C23H16F3N4OS [M-H]- 453.0997, found 453.1001, the error 0.88 ppm.
2.1.2.5. 1-((Benzo[d]thiazol-2-ylamino)(5-chloro-1,3-dimethyl-1H-pyrazol-4-yl)methyl)naphthalen-2-ol (8e)
White solid, yield 83%, Rf 0.09 (petroleum ether/ethyl acetate 2:1), mp 275–278 °C. 1H NMR (400 MHz, DMSO-d6) δ: 10.71 (s, 1H), 9.48 (s, 1H), 7.87–8.14 (m, 1H), 7.86 (d, J = 7.6 Hz, 1H), 7.65 (s, 1H), 7.49–7.52 (m, 2H), 7.39–7.44 (m, 2H), 7.34–7.37 (m, 1H), 7.25 (s, 1H), 7.01–7.04 (m, 1H), 6.77 (s, 1H), 3.74 (s, 3H), 1.82 (s, 3H); 13 C NMR (101 MHz, DMSO-d6) δ: 169.0, 151.7, 149.2, 143.3, 132.7, 131.3, 130.0, 129.7, 129.4, 128.9, 127.6, 127.1, 126.1, 125.1, 124.1, 123.2, 122.3, 118.1, 115.3, 97.4, 33.9, 28.9, 12.5. HRMS (ESI) calcd for C23H20ClN4OS [M + H]+ 435.1046, found 435.1039, the error 1.61 ppm.
2.1.2.6. 1-((Benzo[d]thiazol-2-ylamino)(5-chloro-1-phenyl-3-(trifluoromethyl)-1H-pyrazol-4-yl)methyl)naphthalen-2-ol (8f)
White solid, yield 92%, Rf 0.38 (petroleum ether/ethyl acetate 2:1), mp 194–196 °C. 1H NMR (400 MHz, CDCl3) δ: 12.05 (br, 1H), 8.04 (d, J = 8.0 Hz, 1H), 7.77 (d, J = 8.0 Hz, 1H), 7.72 (d, J = 8.0 Hz, 1H), 7.62 (d, J = 8.0 Hz, 1H), 7.53–7.57 (m, 2H), 7.29–7.36 (m, 8H), 7.14 (d, J = 8.0 Hz, 1H), 6.89 (s, 1H); 13 C NMR (101 MHz, CDCl3) δ: 168.55, 154.72, 150.03, 140.51, 137.19, 132.08, 131.06, 129.54, 129.32, 129.10, 129.06, 128.86, 128.15, 127.10, 126.49, 125.87, 122.97, 122.34, 121.49, 121.37, 119.71, 118.76, 118.34, 115.98, 115.66, 50.15. HRMS (ESI) calcd for C28H19ClF3N4OS [M + H]+ 551.0920, found 551.0913, the error 1.27 ppm.
2.1.2.7. 1-((Benzo[d]thiazol-2-ylamino)(pyrimidin-5-yl)methyl)naphthalen-2-ol (8 g)
White solid, yield 77%, Rf 0.61 (dichloromethane/methanol 100:1), mp 216–219 °C. 1H NMR (400 MHz, DMSO-d6) δ: 10.08 (br, 1H), 9.03 (s, 1H), 8.94 (s, 1H), 8.64 (s, 2H), 7.99 (d, J = 7.4 Hz, 1H), 7.86 (d, J = 8.9 Hz, 2H), 7.71 (d, J = 7.8 Hz, 1H), 7.42–7.47 (m, 1H), 7.41 (d, J = 8.0 Hz, 1H), 7.28–7.35 (m, 2H), 7.20–7.26 (m, 2H), 7.03–7.07 (m, 1H); 13 C NMR (101 MHz, DMSO-d6) δ: 165.6, 156.5, 154.6, 153.6, 151.7, 135.9, 131.9, 130.9, 130.3, 128.7, 128.4, 126.9, 125.5, 122.9, 122.7, 121.3, 121.0, 118.4, 116.6, 99.1, 50.1. HRMS (ESI) calcd for C22H17N4OS [M + H]+ 385.1123, found 385.1118, the error 1.30 ppm.
2.1.2.8. 1-((Benzo[d]thiazol-2-ylamino)(3-bromo-1-(3-chloropyridin-2-yl)-1H-pyrazol-5-yl)methyl)naphthalen-2-ol (8 h)
White solid, yield 73%, Rf 0.68 (petroleum ether/ethyl acetate 10:1), mp 163–165 °C. 1H NMR (400 MHz, CDCl3) δ: 10.85 (br, 1H), 8.18 (d, J = 4.0 Hz, 1H), 7.76 (d, J = 8.3 Hz, 1H), 7.65 (d, J = 8.0 Hz, 1H), 7.51–7.57 (m, 3H), 7.36–7.46 (m, 2H), 7.30–7.34 (m, 2H), 7.26–7.29 (m, 1H), 7.06–7.18 (m, 2H), 6.93–7.01 (m, 2H), 6.57 (s, 1H); 13 C NMR (101 MHz, DMSO-d6) δ: 164.0, 163.8, 154.1, 148.0, 146.6, 139.0, 130.9, 128.9, 128.0, 126.8, 126.6, 125.1, 124.7, 123.2, 122.7, 122.1, 120.9, 119.7, 119.0, 115.7, 115.3, 112.2, 111.0, 110.6, 109.0, 50.1. HRMS (ESI) calcd for C26H16BrClN5OS [M-H]- 561.9927, found 561.9924, the error 0.53 ppm.
2.1.2.9. 1-((Benzo[d]thiazol-2-ylamino)(2-phenyl-2H-1,2,3-triazol-4-yl)methyl)naphthalen-2-ol (8i)
White solid, yield 91%, Rf 0.73 (dichloromethane/methanol 100:1), mp 198–200 °C. 1H NMR (400 MHz, CDCl3) δ: 11.56 (br, 1H), 8.14 (d, J = 7.8 Hz, 2H), 7.75–7.85 (m, 2H), 7.67 (d, J = 8.1 Hz, 1H), 7.56–7.62 (m, 2H), 7.52–7.55 (m, 2H), 7.40–7.44 (m, 1H), 7.35–7.40 (m, 2H), 7.28–7.34 (m, 4H), 7.14–7.16 (m, 1H), 7.06 (s, 1H); 13 C NMR (101 MHz, CDCl3, DMSO-d6) δ: 167.2, 154.3, 150.6, 149.9, 139.7, 134.1, 132.0, 130.7, 130.0, 129.6, 129.2, 128.9, 127.4, 126.6, 126.1, 123.0, 122.9, 122.1, 121.0, 120.2, 118.7, 118.6, 117.2, 49.9. HRMS (ESI) calcd for C26H18N5OS [M-H]- 448.1232, found 448.1235, the error 0.67 ppm.
2.1.2.10. 1-((Benzo[d]thiazol-2-ylamino)(benzofuran-2-yl)methyl)naphthalen-2-ol (8j)
Yellow solid, yield 87%, Rf 0.69 (dichloromethane/methanol 100:1), mp 166–168 °C. 1H NMR (400 MHz, DMSO-d6) δ: 10.35 (s, 1H), 9.07 (d, J = 5.6 Hz, 1H), 8.19 (d, J = 8.5 Hz, 1H), 7.85 (d, J = 8.9 Hz, 2H), 7.72 (d, J = 7.7 Hz, 1H), 7.53–7.59 (m, 1H), 7.51 (d, J = 4.6 Hz, 1H), 7.42–7.48 (m, 3H, Ph-H), 7.30–7.33 (m, 1H), 7.25 (d, J = 7.6 Hz, 1H), 7.17–7.22 (m, 2H), 7.05 (t, J = 7.5 Hz, 1H), 6.76 (s, 1H), 5.78 (s, 1H); 13 C NMR (101 MHz, DMSO-d6) δ: 166.4, 158.7, 154.7, 154.2, 152.4, 133.0, 131.3, 130.6, 129.1, 129.0, 128.7, 127.0, 126.0, 124.1, 123.6, 123.2, 123.1, 121.7, 121.5, 121.3, 118.9, 118.8, 115.9, 111.4, 103.6, 55.4. HRMS (ESI) calcd for C26H19N2O2S [M + H]+ 423.1167, found 423.1153, the error 3.31 ppm.
2.1.2.11. 1-(Benzo[b]thiophen-3-yl(benzo[d]thiazol-2-ylamino)methyl)naphthalen-2-ol (8k)
White solid, yield 86%, Rf 0.66 (dichloromethane/methanol 100:1), mp 199–200 °C. 1H NMR (400 MHz, DMSO-d6) δ: 10.36 (s, 1H), 9.01 (d, J = 6.9 Hz, 1H), 8.23 (d, J = 8.5 Hz, 1H), 7.94 (d, J = 7.9 Hz, 1H), 7.80 (d, J = 8.7 Hz, 2H), 7.68 (d, J = 7.7 Hz, 1H), 7.62 (d, J = 8.0 Hz, 1H), 7.50 (d, J = 6.7 Hz, 2H), 7.37 (d, J = 7.8 Hz, 2H), 7.30 (t, J = 7.8 Hz, 2H), 7.22–7.27 (m, 2H), 7.19 (d, J = 7.4 Hz, 1H), 7.01–7.05 (m, 1H); 13 C NMR (101 MHz, DMSO-d6) δ: 165.7, 153.2, 152.1, 140.2, 137.6, 136.4, 132.5, 130.7, 129.8, 128.6, 126.2, 125.4, 124.2, 124.0, 123.7, 123.5, 123.0, 122.4, 121.8, 121.0, 120.9, 118.4, 118.1, 116.9, 99.5, 50.1. HRMS (ESI) calcd for C26H19N2OS2 [M + H]+ 439.0939, found 439.0925, the error 3.19 ppm.
2.1.2.12. 1-((Benzo[d]thiazol-2-ylamino)(6-chloroimidazo[1,2-a]pyridin-3-yl)methyl)naphthalen-2-ol (8 l)
Yellow solid, yield 75%, Rf 0.13 (petroleum ether/ethyl acetate 3:2), mp 185–187 °C. 1H NMR (400 MHz, CDCl3) δ: 9.95 (s, 1H), 9.24 (s, 1H), 8.27 (s, 1H), 7.96 (d, J = 8.0 Hz, 1H), 7.84 (d, J = 8.0 Hz, 1H), 7.71–7.75 (m, 2H), 7.46–7.53 (m, 3H), 7.35–7.38 (m, 3H), 7.07–7.10 (m, 2H), 6.98 (s, 1H), 6.92 (s, 1H); 13 C NMR (101 MHz, CDCl3) δ: 178.1, 152.0, 148.2, 146.8, 143.3, 130.8, 130.5, 130.3, 129.6, 129.3, 127.9, 127.3, 126.6, 126.3, 126.0, 125.0, 123.6, 123.0, 122.8, 122.1, 121.7, 120.9, 118.1, 116.2, 33.4. HRMS (ESI) calcd for C25H16ClN4OS [M-H]- 455.0733, found 455.0735, the error 0.44 ppm.
2.1.2.13. 1-((Benzo[d]thiazol-2-ylamino)(6-bromoimidazo[1,2-a]pyridin-3-yl)methyl)naphthalen-2-ol (8 m)
Yellow solid, yield 72%, Rf 0.12 (petroleum ether/ethyl acetate 3:2), mp 186–188 °C. 1H NMR (400 MHz, CDCl3) δ: 9.95 (s, 2H), 9.68 (s, 2H), 8.31 (s, 2H), 7.81 (br, 1H), 7.61–7.70 (m, 5H), 7.44 (s, 1H), 7.35 (br, 1H), 7.17 (brs, 1H), 6.99 (s, 1H), 6.93 (s, 1H); 13 C NMR (101 MHz, CDCl3) δ: 178.1, 159.4, 149.2, 147.7, 146.6, 143.5, 133.6, 130.3, 129.6, 129.3, 129.1, 128.8, 128.2, 127.4, 124.9, 124.4, 123.1, 121.9, 118.4, 116.6, 110.4, 107.9, 107.3, 100.0, 33.4. HRMS (ESI) calcd for C25H16BrN4OS [M-H]- 499.0228, found 499.0232, the error 0.80 ppm.
2.1.2.14. 1-((3-Bromo-1-(3-chloropyridin-2-yl)-1H-pyrazol-5-yl)((6-chlorobenzo[d]thiazol-2-yl)amino)methyl)naphthalen-2-ol (8n)
White solid, yield 59%, Rf 0.65 (petroleum ether/ethyl acetate 10:1), mp 213–217 °C. 1H NMR (400 MHz, CDCl3, DMSO-d6) δ: 10.15 (br, 1H), 7.95 (s, 1H), 7.81 (d, J = 8.6 Hz, 1H), 7.41–7.48 (m, 1H), 7.33–7.38 (m, 1H), 7.20–7.32 (m, 5H), 7.13 (s, 1H), 6.96–7.09 (m, 2H), 6.68–6.88 (m, 2H), 6.42 (s, 1H); 13 C NMR (101 MHz, CDCl3, DMSO-d6) δ: 154.9, 153.9, 147.9, 147.7, 146.6, 145.5, 138.7, 137.5, 137.3, 131.6, 131.6, 130.3, 128.3, 127.2, 126.6, 126.6, 126.0, 124.6, 122.9, 122.4, 120.2, 119.4, 119.1, 116.2, 109.0, 48.6. HRMS (ESI) calcd for C26H17BrCl2N5OS [M + H]+ 595.9714, found 595.9702, the error 2.01 ppm.
2.1.2.15. 1-(((6-Chlorobenzo[d]thiazol-2-yl)amino)(2-phenyl-2H-1,2,3-triazol-4-yl)methyl)naphthalen-2-ol (8o)
White solid, yield 93%, Rf 0.72 (dichloromethane/methanol 100:1), mp 194–197 °C. 1H NMR (400 MHz, CDCl3) δ: 11.24 (br, 1H), 8.12 (d, J = 7.9 Hz, 2H), 7.76–7.83 (m, 2H), 7.60 (s, 1H), 7.50–7.58 (m, 4H), 7.38–7.43 (m, 2H), 7.35 (d, J = 8.9 Hz, 1H), 7.32 (d, J = 2.1 Hz, 1H), 7.30 (d, J = 2.1 Hz, 1H), 7.29 (s, 2H), 7.02 (s, 1H); 13 C NMR (101 MHz, CDCl3) δ: 174.9, 167.0, 154.6, 149.3, 148.7, 139.6, 134.0, 131.8, 131.3, 131.1, 130.2, 129.4, 129.0, 127.9, 127.7, 126.8, 126.5, 123.2, 123.1, 120.8, 119.5, 118.8, 100.0, 50.1. HRMS (ESI) calcd for C26H19ClN5OS [M + H]+ 484.0999, found 484.0985, the error 2.89 ppm.
2.1.2.16. 1-((3-Bromo-1-(3-chloropyridin-2-yl)-1H-pyrazol-5-yl)((6-(trifluoromethyl)benzo[d]thiazol-2-yl)amino)methyl)naphthalen-2-ol (8p)
White solid, yield 60%, Rf 0.64 (petroleum ether/ethyl acetate 10:1), mp 215–218 °C. 1H NMR (400 MHz, DMSO-d6) δ: 10.07 (s, 1H), 9.21 (d, J = 7.9 Hz, 1H), 8.13–8.16 (m, 2H), 7.92 (d, J = 8.5 Hz, 1H), 7.66 (t, J = 7.5 Hz, 2H), 7.52–7.63 (m, 3H), 7.46 (d, J = 7.5 Hz, 1H), 7.38 (t, J = 7.5 Hz, 1H), 7.22 (t, J = 7.2 Hz, 1H), 7.10 (s, 1H), 6.97 (d, J = 7.2 Hz, 1H), 6.59 (s, 1H); 13 C NMR (101 MHz, DMSO-d6) δ: 168.2, 154.8, 153.8, 148.8, 146.9, 139.2, 131.6, 130.1, 128.3, 128.2, 127.7, 127.4, 126.4, 125.5, 124.0 (q, J = 286.84 Hz, CF3), 123.5, 122.5, 122.5, 122.2, 121.2, 118.7, 118.2, 117.9, 114.5, 108.6, 99.5, 47.3. HRMS (ESI) calcd for C27H17BrClF3N5OS [M + H]+ 629.9978, found 629.9973, the error 0.79 ppm.
2.1.2.17. 1-((2-Phenyl-2H-1,2,3-triazol-4-yl)((6-(trifluoromethyl)benzo[d]thiazol-2-yl)amino)methyl)naphthalen-2-ol (8q)
White solid, yield 63%, Rf 0.73 (dichloromethane/methanol 100:1), mp 176–180 °C. 1H NMR (400 MHz, CDCl3) δ: 11.02 (br, 1H), 8.10 (d, J = 7.8 Hz, 2H), 7.87 (s, 1H), 7.76–7.83 (m, 2H), 7.69 (d, J = 8.5 Hz, 2H), 7.59 (d, J = 8.4 Hz, 2H), 7.49–7.54 (m, 2H), 7.36–7.42 (m, 3H), 7.27–7.33 (m, 2H), 7.06 (s, 1H); 13 C NMR (101 MHz, CDCl3) δ: 154.2, 152.5, 149.0, 139.6, 135.3, 135.3, 131.8, 131.4, 130.0, 129.4, 129.1, 127.7, 127.0, 126.8, 125.4, 124.3 (q, J = 272.70 Hz, CF3), 123.7, 123.7, 123.4, 118.8, 118.6, 116.6, 115.5, 113.8, 50.4. HRMS (ESI) calcd for C27H19F3N5OS [M + H]+ 518.1262, found 518.1254, the error 1.54 ppm.
2.2. Biological activity test
The larvicidal/acaricidal activities of the title compounds against oriental armyworm (Mythimna separata Walker), corn borer (Ostrinia nubilalis), diamondback moth (Plutella xylostella L.), mosquito (Culex pipiens pallen), bean aphid (Aphis craccivora), and spider mite (Tetranychus cinnabarinus) were investigated in relationship with the contrasts viii, ix, and chlorantraniliprole in a greenhouse in accordance with the published literature precedentsCitation36–42. Partial bioassay procedures can also be found in the supporting information. According to statistical requirements, each bioassay was repeated three times. Assessments were made on a dead-alive basis, and lethality rates were corrected using Abbott’s formulaCitation43. Evaluations were based on a percentage scale of 0–100%, where 0% equals no activity, and 100% equals total kill. The standard deviations of the tested biological values were within ±5%.
2.3. Calcium imaging experiments
Effects of 8 h, 8i, and viii on calcium ion channels in the central neurons isolated from the third-instar of M. separata were studied by calcium imaging techniques according to the referencesCitation34,Citation44. The detailed procedure is presented in the supporting information.
3. Results and discussion
3.1. Chemistry
As shown in Scheme 1, intermediate 5-chloro-1,3-disubstituted-1H-pyrazole-4-carbaldehydes (2a-b) were prepared via the cyclisation reaction of β-keto ester with hydrazine, and the subsequent POCl3-DMF Vilsmeier-Haack reaction of the generated pyrazolones (1a-b) with high yields following the known literature protocolsCitation28–32; using a similar procedure in the earlier reportCitation34, pyridylpyrazole carbaldehyde 2c was prepared via LiAlH4-assisted reduction of pyrazole ester 3, followed by subsequent PCC-mediated oxidation of the resultant pyrazole alcohol 4; phenyltriazole carbaldehyde 2d was smoothly synthesised from the intermediate triazolyl tetraol 5 using NaIO4-oxidation methodCitation35.
The synthetic route for the title compounds 1-(((6-substitutedbenzo[d]thiazol-2-yl)amino)(heteroaryl)methyl) naphthalen-2-ol (8a-q) is shown in Scheme 2. Using a one-pot pattern of the three component Betti reactionCitation45–47, naphthalen-2-ol (6), heteroaryl aldehyde (2) and 6-substitutedbenzo[d]thiazol-2-amine (7) were reacted at 115–125 °C without any solvent for 1–2 h to give the title compounds 8a-q in 57–93% yields. In the workup part of the reaction, the products were purified through either adding solvent-filtering-washing operation sequence, or direct column chromatography of the reaction mixture, indicating a simple, convenient and efficient procedure. Based on an Agilent 1260-II high performance liquid chromatography (HPLC) analysis, the representative compounds 8a, 8d-8i, 8 l, 8o, and 8q were found to possess high purities of 90.11–100.00%.
The possible mechanism for this three-component reaction is presented in Scheme 3. In the second step of the process, the nucleophilic attack by the NH2 group of the reactant 2-aminobenzothiazole to the planar C=C double bond of α,β-unsaturated ketone should generate the corresponding intermediate containing a chiral carbon atom in the form of racemic mixture, due to the equal probability of the attack from both sides of the plane. Meanwhile, considering that the final compounds 8 were prepared under achiral condition, and taking into account their respective 1H NMR and 13 C NMR spectra as well, the obtained products 8a-8q should be considered as racemic mixtures.
The novel title compounds 8 were identified and characterised by means of 1H NMR, 13 C NMR, HRMS and the melting point analyses. In their 1H NMR spectra, the chemical shifts of the –OH protons appeared at δ 9.95–12.44 ppm; the CH proton signals in the –CH–NH– part were observed at δ 5.59–7.11 ppm due to the presence of different heteroaryl substituents. In the 13 C NMR spectra, the signals of carbon at 2- position (between N and S atoms) of the benzothiazole moiety, in majority of cases were found in the most high frequency area, at δ 165.6–178.1 ppm; the carbon signals in the –CH–NH– part were observed at δ 31.2–60.9 ppm, likewise, thanks to the various heteroaryl motifs.
3.2. Biological activities
The larvicidal/acaricidal activities of the title compounds against oriental armyworm (Mythimna separata Walker), corn borer (Ostrinia nubilalis), diamondback moth (Plutella xylostella L.), mosquito (Culex pipiens pallen), bean aphid (Aphis craccivora), and spider mite (Tetranychus cinnabarinus) were investigated in relationship with the contrasts viii, ix, and chlorantraniliprole in a greenhouse.
The insecticidal activities of the title compounds against larvae of oriental armyworm, corn borer, bean aphid, and mosquito are shown in . At a test concentration of 200 mg·L−1, most of the compounds were found to exhibit obvious larvicidal activities against oriental armyworm, among which 8a-8c, 8f, 8 h-8k, 8 m, 8o, and 8p possessed lethality rates of 50–100%, thus showing better insecticidal activities than the control viii (30%). Compounds 8a-8c, 8 h, and 8j could still display favourable activities of 50–70% at a 100 mg·L−1 concentration, similar to that of the control ix (60%). Towards corn borer, most of the compounds also showed apparent larvicidal activities at a 200 mg·L−1 concentration with lethality rates of 25–65%, and were better than the control viii (10%), but less effective than ix (75%). Compounds 8 h, 8i, 8 m, and 8p exhibited insecticidal activities of 25–60% against bean aphid at a concentration of 200 mg·L−1, among which 8 h and 8i lethality rates of which were 40% and 60%, respectively, had similar insecticidal effects as for ix (50%). Surprisingly, the control viii showed no activity against bean aphid at this test concentration. The bioassay result for partial compounds (8d, 8 h, 8i, etc.) against mosquito indicated they have moderate to weak insecticidal activities (5–35%) at 10 mg·L−1 concentration, likewise with those of the two controls – viii and ix.
Table 1. Insecticidall activity (% lethality rate) of the title compounds against larvae of oriental armyworm (Mythimna separata Walker), corn borer (Ostrinia nubilalis), bean aphid (Aphis craccivora), and mosquito (Culex pipiens pallens) at different test concentrations.
As shown in , most of the title compounds and the controls viii and ix displayed excellent insecticidal activities against diamondback moth larvae at the test concentrations of 200 mg·L−1 and 100 mg·L−1. At lower dosage, compounds 8a-8c, 8f-8h, 8j-8l, 8n, and 8o had 50–95% insecticidal activities at a concentration of 10 mg·L−1, even higher than those of above two controls; on top of this, 8 b, 8f, 8 g, 8j, 8k, 8n, and 8o also possessed lethality rates of 65–88% at a concentration of 1 mg·L−1, indicating that these compounds have better insecticidal effect than those of the two controls towards diamondback moth.
Table 2. Insecticidal activity (% lethality rate) against larvae of diamondback moth (Plutella xylostella L.) and acaricidal activity against adult spider mite (Tetranychus cinnabarinus) of the title compounds at different test concentrations.
Moreover, the acaricidal activity results of the compounds against adult spider mite are also listed in . As shown in the table, some of the compounds exhibited good acaricidal activities at 200 mg·L−1 concentration, such as 8 h − 50%, 8i − 100%, 8 l − 60%, 8 m − 80%, and were comparable with the controls viii (90%) and ix (70%). At a concentration of 100 mg·L−1, compounds 8 l and 8 m lethality rates of which were 30% and 40%, respectively, had the similar acaricidal levels as for ix (30%), while being less effective than viii (60%). It is worth noting that compound 8i with 90% activity at 100 mg·L−1 concentration is superior to all the controls. Interestingly, the control chlorantraniliprole which exhibited excellent and the best insecticidal activity in all these biological tests, just showed 40% and 0% acaricidal activities against spider mite at 200 mg·L−1 and 100 mg·L−1 concentrations, respectively.
3.3. Structure-activity relationships
Based on the preliminary biological assay results, the following structure-activity relationships could be found:
Generally, when R = H, the compound bearing benzofuryl group (8j) displayed remarkable insecticidal activities. The other heterocyclic substituents (Het), such as imidazolyl, (substituted)pyrazolyl, (Ph)triazolyl and benzothienyl groups also exhibited different degrees of favourable insecticidal potentials. Moreover, when R = H, the Het group in corresponding compounds against spider mite showed an acaricidal activity trend: (Ph)triazolyl > (Br)imidazopyridyl > (Cl)imidazopyridyl > (Br)(2-ClPy)pyrazolyl (i.e. in , 8i > 8 m > 8 l > 8 h). Among these compounds, 8i with a heterocyclic substituent being a (Ph)triazolyl group possessed the best acaricidal potential. These results indicate that the smaller and less electronegative groups would be more favourable for the increase of acaricidal activities of the compounds.
When R = Cl and CF3, respectively, the Het group in corresponding compounds against both oriental armyworm and corn borer showed the activity sequence of (Ph)triazolyl ≫ (Br)(2-ClPy)pyrazolyl (i.e. 8o ≫ 8n), and (Ph)triazolyl ≈ (Br)(2-ClPy)pyrazolyl (i.e. 8q ≈ 8p), respectively, indicating the similar positive influence of the smaller and less electronegative Het groups on the insecticidal activities; when R = Cl or CF3, the Het group in the related compounds against diamondback moth showed an opposite activity trend of (Br)(2-ClPy)pyrazolyl > (Ph)triazolyl (i.e. 8n > 8o; 8p > 8q). When the Het group is fixed as (Br)(2-ClPy)pyrazolyl or (Ph)triazolyl, the activity trend for R group is Cl > H > CF3 (i.e. 8n > 8 h > 8p; 8o > 8i > 8q) in diamondback moth tests.
When the group R in benzothiazole ring is H, the heterocycle substituents (Het) such as imidazole, pyridine, pyrimidine and simply-substituted pyrazole moiety are beneficial for the maintenance of the insecticidal activities towards diamondback moth, e.g., in the case of compounds 8a-8c and 8 g. In particular, pyrazole ring bearing three electron-donating Me groups (i.e., 8c) greatly contributed to the activity improvement compared to those bearing electron-withdrawing groups CF3 (i.e., 8d) and Cl (i.e., 8e), indicating that groups with stronger electronegativity in pyrazole ring generally was unfavourable. Furthermore, the fused-heterocycle substituents such as benzofuran and benzothiophene groups could lead to high insecticidal activities of the related compounds. While the former exhibited a better trend for the activity enhancement than that of the latter, hence indicating that the smaller and more electronegative groups would be favourable. Bi-(hetero)aryl substituents also showed similar trend, that is, the activity sequence corresponding to the Het groups being as follows: (Ph)(Cl,CF3)pyrazolyl (8f) > (Br)(2-ClPy)pyrazolyl (8 h) > (Ph)triazolyl (8i).
In addition, OH and NH groups present in the skeleton of this type of compounds can both serve as hydrogen bond donors as well as acceptors. Besides this, there are also one or more O/N atoms in the Het substituents that could be hydrogen bond acceptors. Whereas according to the preliminary bioassay results, it seems that the type and number of these hydrogen bond acceptors do not have much influence on the insecticidal activities of the corresponding compounds.
Taking it by and large, these compounds towards oriental armyworm showed a consistent activity trend against diamondback moth. Interestingly, there are a few exceptions, e.g., compounds 8n and 8 g which possessed very high insecticidal activities towards diamondback moth, while showing rather weak activities in oriental armyworm tests at 200 mg·L−1 concentration. This may be due to the different selectivity of the compounds to different insects, Mythimna separata Walker and Plutella xylostella L.
Compounds 8 b, 8f, 8 g, 8j, 8k, 8n, and 8o with favourable insecticidal potentials were also determined the LC50 values against diamondback moth (Plutella xylostella L.) with compounds ix and chlorantraniliprole as controls. As shown in , the tested compounds held LC50 values of 0.0988–5.8864 mg·L−1 and were all superior to the control ix (LC50: 13.5493 mg·L−1) towards diamondback moth.
Table 3. LC50 values of the compounds against diamondback moth (Plutella xylostella L.)a
In general, compared with the phenyl groups in the structures of the controls viii and ix, some of the heterocyclic groups in these synthesised compounds showed favourable potential for the enhancement of the insecticidal and acaricidal activities of the parent structures. Compounds 8 b, 8c, 8f, 8 g, 8 h, 8i, 8j, 8k, 8n, and 8o could be considered as new insecticidal leading structures, meanwhile compounds 8i, 8 l, and 8 m could also be viewed as novel acaricidal leading structures for further investigations.
3.4. The effects of β-naphthol derivatives on the insect calcium ion channels
Taking into account the promising bioactivities of these synthesised novel β-naphthol derivatives, the effects on the calcium ion channels of the representative compounds 8 h, 8i, and viii with high bioactivities were further tested. The experiments were performed by using the calcium imaging techniquesCitation34,Citation44 on central neurons isolated from the third-instar of oriental armyworm (M. separata) after neurons being loaded with fluo-3 AM (The detailed procedure was presented in the supporting information). shows the free calcium ion change in neuron cytoplasm versus recorded time when the central neurons were treated with each of 8 h, 8i, viii, and chlorantraniliprole in the absence of extracellular calcium ions.
Figure 2. Effects of compounds 8 h (a), 8i (b), and viii (c) at different concentrations on [Ca2+]i on the central neurons of M. separata when extracellular calcium was absent (The central neurons of third-instar larvae of M. separata were dyed with fluo-3 AM).
![Figure 2. Effects of compounds 8 h (a), 8i (b), and viii (c) at different concentrations on [Ca2+]i on the central neurons of M. separata when extracellular calcium was absent (The central neurons of third-instar larvae of M. separata were dyed with fluo-3 AM).](/cms/asset/10cda45c-2d93-4e04-98f4-f7e6c8933c6c/ienz_a_2032687_f0002_c.jpg)
According to the information shown in , the following results could be found: when treating neurons with each of compounds 8 h, 8i, and viii at a low concentration of 1 mg·L−1, no significant increase in [Ca2+]i was noticed; however, when being treated with each of these three compounds and chlorantraniliprole at 5 mg·L−1 concentration, increase of about 3.27% for 8 h (103.27 ± 1.85%, n = 12), 1.90% for 8i (101.90 ± 1.31%, n = 8), and 0.96% for viii (100.96 ± 0.60%, n = 7) in [Ca2+]i was detected, respectively, which in turn is lower than that of the chlorantraniliprole (5 mg·L−1, 105.22 ± 1.86%, n = 4); when treating the neurons with each of the three compounds (8 h, 8i, and viii) at a higher concentration of 50 mg·L−1, the increase of [Ca2+]i caused by these compounds was more notable, that is, there was an increase of about 5.92% of [Ca2+]i for 8 h (105.92 ± 2.42%, n = 10), 3.85% for 8i (103.85 ± 1.44%, n = 9), and 3.09% for viii (103.09 ± 0.54%, n = 5). These results indicate that the three compounds could activate the release of calcium ions in insect central neurons at a comparatively higher concentration.
Furthermore, when the neurons were preincubated with 0.5 µM ryanodine (a potent RyR activator) for 5 min, the free calcium ions were found to be elevated about 1.56% after treating with 5 mg·L−1 of 8i (101.56 ± 1.00, n = 5), signifying that the calcium ions released from the endoplasmic reticulum increased to a certain degree compared to those of unincubated with ryanodineCitation44,Citation48. However, there were no significant changes for free calcium ion concentration in the cases of 8 h (5 mg·L−1, 100.63 ± 0.62, n = 3) and viii (5 mg·L−1, 100.38 ± 0.54, n = 3). Based on the stress time, the increase of the calcium signal triggered by these novel compounds, is more likely to be a stress response, and probably not derived from the activation of RyR as in case of treatment with chlorantraniliprole.
Compared with chlorantraniliprole, all three compounds overall exhibited a modest effect on activating the release of calcium ions in insect central neurons. Besides the possible reason of being weak RyR modulators, very high liposolubility of these compounds as seen in CLogP values predicted by the ChemDraw Professional software (6.468 for 8 h, 5.977 for 8i, 5.799 for viii, and 3.194 for chlorantraniprole) could be another key factor determining the observed outcome. Among the three compounds, 8 h showed better effect on activating the release of calcium ions at higher test concentrations than those of 8i and viii, may be due to the presence of more electronegative and bulkier groups in the heterocyclic moieties (Br, Cl Vs. H, and OMe; (Py)pyrazole ring Vs. (Ph)triazole ring, and benzene ring).
4. Conclusions
In summary, a series of novel β-naphthol derivatives containing benzothiazolylamino and heteroaryl groups 8a-q were efficiently synthesised via a one-pot pattern of the three component Betti reaction, and their structures were identified and characterised by melting points, 1H NMR, 13 C NMR and HRMS analyses. The insecticidal and acaricidal activities of these new compounds towards a variety of agricultural pests were evaluated. The bioassay results showed that most of the title compounds possessed favourable insecticidal activities particularly towards oriental armyworm (50–100% at 200 mg·L−1) and diamondback moth (50–95% at 10 mg·L−1). Compounds 8 b, 8f, 8 g, 8j, 8k, 8n, and 8o possessed LC50 values of 0.0988–5.8864 mg·L−1 against diamondback moth. Moreover, compounds 8 h, 8i, 8 l, and 8 m held lethality rates of 50–100% against spider mite at 200 mg·L−1 concentration, especially 8i, 8 l, and 8 m featuring activities of 30–90% at 100 mg·L−1 concentration which were comparable with those of the controls. On the whole, compounds 8 b, 8c, 8f, 8 g, 8 h, 8i, 8j, 8k, 8n, and 8o could be viewed as new insecticidal leading structures, and on top of that, compounds 8i, 8 l, and 8 m could also serve as novel acaricidal leading structures for further investigations. The calcium imaging experiments revealed that 8 h, 8i, and viii could activate the release of calcium ions in insect central neurons at a higher concentration (50 mg·L−1). It was speculated that this change is more likely to be a stress response in the present study. In view of the promising application potentials of phenolic and heterocyclic compounds in medicinal and pesticidal chemistry, more novel β-naphthol compounds could hopefully be developed with potent insecticidal/acaricidal activities and low toxicities with the further optimisation of the leading structures in this research. These studies will give the significant input for the design and development of new generation of insecticidal/acaricidal agrochemicals.
Supplemental Material
Download PDF (3.7 MB)Acknowledgement
The authors express heartfelt appreciation to Dr. Shorena Gelozia for the kind linguistic modification of this article. Dedicated to the 60th anniversary of Institute of Elemento-Organic Chemistry (Nankai University).
Disclosure statement
No potential conflict of interest was reported by the authors.
Additional information
Funding
References
- Oerke EC, Dehne HW. Safe guarding production - losses in major crops and the role of crop. Crop Prot 2004;23:275–85.
- Sparks TC, Lorsbach BA. Perspectives on the agrochemical industry and agrochemical discovery. Pest Manag Sci 2017;73:672–7.
- Liu X-H, Yu W, Min L-J, et al. Synthesis and pesticidal activities of new quinoxalines. J Agric Food Chem 2020;68:7324–32.
- Fu Q, Cai P-P, Cheng L, et al. Synthesis and herbicidal activity of novel pyrazole aromatic ketone analogs as HPPD inhibitor. Pest Manag Sci 2020;76:868–79.
- Jenner AM, Rafter J, Halliwell B. Human fecal water content of phenolics: The extent of colonic exposure to aromatic compounds. Free Radical Bio Med 2005;38:763–72.
- Li F, Nitteranon V, Tang X, et al. In vitro antioxidant and anti-inflammatory activities of 1-dehydro-[6]-gingerdione, 6-shogaol, 6-dehydroshogaol and hexahydrocurcumin. Food Chem 2012;135:332–7.
- Chen C-Y, Yang W-L, Kuo S-Y. Cytotoxic activity and cell cycle analysis of hexahydrocurcumin on SW 480 human colorectal cancer cells. Nat Prod Commun 2011;6:1671–2.
- Tevyashova AN. Olivomycin A - an antitumor antibiotic of the aureolic acid group (Review). Pharm Chem J 2016;50:425–30.
- Shahabadi N, Akbari A, Karampour F, et al. Cytotoxicity and antibacterial activities of new chemically synthesized magnetic nanoparticles containing eugenol. J Drug Deliv Sci Tec 2019;49:113–22.
- Heusinkveld HJ, van Vliet AC, Nijssen PCG, et al. In vitro neurotoxic hazard characterisation of dinitrophenolic herbicides. Toxicol Lett 2016;252:62–9.
- Kataria HR, Gisi U. Interactions of fungicide-herbicide combinations against plant pathogens and weeds. Crop Prot 1990;9:403–9.
- Ma W, Fan Y, Liu Z, et al. The acaricidal activity and mechanism of eugenol on Psoroptes cuniculi. Vet Parasitol 2019;266:56–62.
- Khan SN, Khan S, Misba L, et al. Synergistic fungicidal activity with low doses of eugenol and amphotericin B against Candida albicans. Biochem Biophys Res Commun 2019;518:459–64.
- Zertal A, Jacquet M, Lavédrine B, et al. Photodegradation of chlorinated pesticides dispersed on sand. Chemosphere 2005;58:1431–7.
- Vinciguerra V, Rojas F, Tedesco V, et al. Chemical characterization and antifungal activity of Origanum vulgare, Thymus vulgaris essential oils and carvacrol against Malassezia furfur. Nat Prod Res 2019;33:3273–7.
- Lu L, Zhang M, Di Y, et al. Insecticidal effects of thymol against Caenorhabditis elegans at different stages. J Agr Sci Tech 2019;21:97–103.
- Rojas MG, Morales-Ramos JA, Wan PJ, Use of gossypol and related terpenes for control of urban and agricultural pests. US6773727, 2004.
- Puckhaber LS, Dowd MK, Stipanovic RD, et al. Toxicity of (+)- and (-)-gossypol to the plant pathogen, Rhizoctonia solani. J Agric Food Chem 2002;50:7017–21.
- Mellon JE, Zelaya CA, Dowd MK, et al. Inhibitory effects of gossypol, gossypolone, and apogossypolone on a collection of economically important filamentous fungi. J Agric Food Chem 2012;60:2740–5.
- Kalavagunta PK, Pala R, Pathipati UR, et al. Identification of naphthol derivatives as novel antifeedants and insecticides. 1. J Agric Food Chem 2014;62:6571–6.
- Kalavagunta PK, Bagul PK, Jallapally A, et al. Design and green synthesis of 2-(diarylalkyl)aminobenzothiazole derivatives and their dual activities as angiotensin converting enzyme inhibitors and calcium channel blockers. Eur J Med Chem 2014;83:344–54.
- Tohnishi M, Nakao H, Furuya T, et al. Flubendiamide, a novel insecticide highly active against lepidopterous insect pests. J Pestic Sci 2005;30:354–60.
- Lahm GP, Selby TP, Freudenberger JH, et al. Insecticidal anthranilic diamides: a new class of potent ryanodine receptor activators. Bioorg Med Chem Lett 2005;15:4898–906.
- Lamberth C. Heterocyclic chemistry in crop protection. Pest Manag Sci 2013;69:1106–14.
- Yu C-S, Wang Q, Bajsa-Hirschel J, et al. Synthesis, crystal structure, herbicidal Activity, and SAR Study of Novel N-(Arylmethoxy)-2-chloronicotinamides Derived from Nicotinic Acid. J Agric Food Chem 2021;69:6423–30.
- Lahm GP, Stevenson TM, Selby TP, et al. Rynaxypyr: a new insecticidal anthranilic diamide that acts as a potent and selective ryanodine receptor activator. Bioorg Med Chem Lett 2007;17:6274–9.
- Dong W, Xu J, Xiong L, et al. Synthesis, structure and biological activities of some novel anthranilic acid esters containing N-pyridylpyrazole. Chin J Chem 2009;27:579–86.
- Wang B-L, Li Q-N, Zhan Y-Z, et al. The synthesis and biological activities of [5-(4-substitutedphenylsulfinyl/sulfonyl)-1,3-dimethyl-1H-pyrazol-4-yl]-arylmethanones. Phosphorus Sulfur Silicon Relat Elem 2014;189:483–91.
- Snavely FA, Trahanovsky WS, Suydam FH. Infrared study of arylazopyrazolone compounds and their copper derivatives. J Org Chem 1962;27:994–7.
- Grillot GF, Aftergut S, Botteron DG. Trifluoroantipyrene. J Org Chem 1958;23:119–20.
- Porai-Koshits BA, Kvitko IYa, Shutkova EA. Synthesis and transformations of chloropyrazolealdehydes. Pharm Chem J 1970;4:138–43.
- Nayak M, Batchu H, Batra S. Straightforward copper-catalyzed synthesis of pyrrolopyrazoles from halogenated pyrazolecarbaldehydes. Tetrahedron Lett 2012;53:4206–8.
- Palka B, Di Capua A, Anzini M, et al. Synthesis of trifluoromethyl-substituted pyrazolo[4,3-c]pyridines - sequential versus multicomponent reaction approach. Beilstein J Org Chem 2014;10:1759–64.
- Zhou Y, Feng Q, Di F, et al. Synthesis and insecticidal activities of 2,3-dihydroquinazolin-4(1H)-one derivatives targeting calcium channel. Bioorg Med Chem 2013;21:4968–75.
- Hann RM, Hudson CS. The action of copper sulfate on phenylosazones of the sugars. Phenyl-D-glucosotriazole. J Am Chem Soc 1944;66:735–8.
- Wang B-L, Zhu H-W, Li Z-M, et al. One-pot synthesis, structure and structure-activity relationship of novel bioactive diphenyl/diethyl (3-bromo-1-(3-chloropyridin-2-yl)-1H-pyrazol-5-yl)(arylamino)methylphosphonates. Pest Manag Sci 2019;75:3273–81.
- Wang B, Wang H, Liu H, et al. Synthesis and structure-insecticidal activity relationship of novel phenylpyrazole carboxylic acid derivatives containing fluorine moiety. Chin Chem Lett 2020;31:739–45.
- Sun R, Li Y, Xiong L, et al. Design, synthesis, and insecticidal evaluation of new benzoylureas containing isoxazoline and isoxazole group. J Agric Food Chem 2011;59:4851–9.
- Wang B-L, Zhu H-W, Ma Y, et al. Synthesis, insecticidal activities, and SAR studies of novel pyridylpyrazole acid derivatives based on amide bridge modification of anthranilic diamide insecticides. J Agric Food Chem 2013;61:5483–93.
- Yu X, Liu Y, Li Y, et al. Design, synthesis, acaricidal/insecticidal activity, and structure-activity relationship studies of novel oxazolines containing sulfone/sulfoxide groups based on the sulfonylurea receptor protein-binding site. J Agric Food Chem 2016;64:3034–40.
- Chen L, Huang Z, Wang Q, et al. Insecticidal benzoylphenylurea-S-carbamate: a new propesticide with two effects of both benzoylphenylureas and carbamates. J Agric Food Chem 2007;55:2659–63.
- Yu X, Zhang Y, Liu Y, et al. Synthesis and acaricidal- and insecticidal-activity evaluation of novel oxazolines containing sulfiliminyl moieties and their derivatives. J Agric Food Chem 2019;67:4224–31.
- Abbott WS. A method of computing the effectiveness of an insecticide. J Econ Entomol 1925;18:265–7.
- Liu J-B, Li F-Y, Li Y-X, et al. Synthesis, insecticidal evaluation and 3D-QSAR study of novel anthranilic diamide derivatives as potential ryanodine receptor modulators. Pest Manag Sci 2019;75:1034–44.
- Betti M. Condensation between β-naphthol, aldehydes, and amines. Gazz Chim Ital 1900;30:310–6.
- Cardellicchio C, Capozzi MAM, Naso F. The Betti base: the awakening of a sleeping beauty. Tetrahedron-Asymmetr 2010;21:507–17.
- Shahrisa A, Teimuri-Mofrad R, Gholamhosseini-Nazari M. Synthesis of a new class of Betti bases by the Mannich-type reaction: efficient, facile, solvent-free and one-pot protocol. Mol Divers 2015;19:87–101.
- Liu J-B, Li F-Y, Dong J-Y, et al. Anthranilic diamides derivatives as potential ryanodine receptor modulators: Synthesis, biological evaluation and structure activity relationship. Bioorg Med Chem 2018;26:3541–50.