Abstract
A series of 1,2,3-triazole analogues as novel fat mass and obesity-associated protein (FTO) inhibitors were synthesised in this study. Among all 1,2,3-triazoles, compound C6 exhibited the most robust inhibition of FTO with an IC50 value of 780 nM. It displayed the potent antiproliferative activity against KYSE-150, KYSE-270, TE-1, KYSE-510, and EC109 cell lines with IC50 value of 2.17, 1.35, 0.95, 4.15, and 0.83 μM, respectively. In addition, C6 arrested the cell cycle at G2 phase against TE-1 and EC109 cells in a concentration-dependent manner. Analysis of cellular mechanisms demonstrated that C6 concentration-dependently regulated epithelial mesenchymal transition (EMT) pathway and PI3K/AKT pathway against TE-1 and EC109 cells. Molecular docking studies that C6 formed important hydrogen-bond interaction with Lys107, Asn110, Tyr108, and Leu109 of FTO. These findings suggested that C6 as a novel FTO inhibitor and orally antitumor agent deserves further investigation to treat esophageal cancer.
GRAPHICAL ABSTRACT
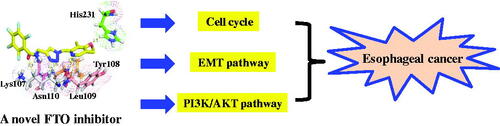
1. Introduction
Esophageal cancer as the eighth most common cancer in the world possesses the poor prognosis and poor survivalCitation1. It is necessary to develop effective and novel drugs to treat esophageal cancerCitation2. Fat mass and obesity-associated protein (FTO), a demethylase for N6-methyladenosine modification, has been implicated in esophageal cancerCitation3. Recent report demonstrated that esophageal cancer tissues had the increased FTO expression which correlated with clinical esophageal cancer prognosisCitation4. In addition, FTO could play oncogenic roles and promote cell proliferation and migration in esophageal cancerCitation5. Therefore, FTO might be a potential target and FTO inhibitors might be effective and novel anticancer agents for the treatment of esophageal cancer.
1,2,3-Triazole as one of the most important classes of nitrogen-containing heterocycle exhibits potent anticancer activityCitation6. 1,2,3-triazole-benzoxazole hybrid 1 () displayed antiproliferative activity against SKBr3, HepG2, and HeLa cells with IC50 values of 7.1, 11.2, and 6.8 μg/mLCitation7. 1,2,3-Triazole-benzisoxazole hybrid 2 showed antiproliferative activity against MOLM13, MOLM14, and MV4-11 cell linesCitation8. Hybrid 3 inhibited migration and mammosphere formation and induced cell cycle arrest at G2-M phase against breast cancer cellsCitation9. On the other hand, pyridine derivatives also have a wide-range of therapeutic applications in the area of drug discoveryCitation10. Pyridine analogue FTO-IN-5 () as a selective FTO inhibitor could decrease the viability of acute monocytic leukaemia cells and increase the level of N6-methyladenosine in mRNACitation11. Pyridine analogue FTO-IN-6 selectively inhibited FTO and formed hydrogen bonds with residues Ser318 and Tyr295Citation12.
Molecular hybridisation, involving a combination of two or more bioactive scaffolds to generate a single molecular architecture, has been a promising strategy in the drug discovery researchCitation13. Furthermore, fluorine as the most electronegative element plays a key role to design anticancer agentsCitation14. Therefore, a class of 1,2,3-triazole-pyridine hybrids containing a pentafluorobenzoyl moiety as potential FTO inhibitors was designed by the molecular hybridisation. In addition, these compounds were evaluated for their anticancer activity in vitro and in vivo against esophageal cancer cell lines. To the best of our knowledge, it is the first time to discover that 1,2,3-triazole-pyridine hybrids could be potential anticancer agents by targeting FTO for the treatment of esophageal cancer.
2. Materials and methods
2.1. Chemistry experimental procedures
Chemical reagents and organic solvents were purchased from commercial sources (Innochem, Beijing, China). Melting points of all compounds were measured using a melting point detector (Tianjin Jingtuo Instrument Technology Co., LTD, Tianjin, China). 1HNMR and 13CNMR spectrum of all new compounds were recorded on a Bruker spectrometer (Bruker, Karlsruhe, Germany). High-resolution mass spectra were recorded on a Waters Micromass spectrometer (Waters, Shanghai, China).
2.2. Synthesis of compound B
A mixture of 2,3,4,5,6-pentafluorobenzoyl chloride A (5 mmol), triethylamine (6 mmol), and prop-2-yn-1-amine (6 mmol) was refluxed in dichloromethane (25 ml) for 6 h. Upon completion, the reaction system was washed with dichloromethane to afford the crude product. The crude product was purified by silica gel chromatography (PE:EA = 7:1) to yield compound B.
2.3. Synthesis of compound C1∼C8
The mixture of compound B (1 mmol), azide derivatives (1 mmol), copper sulphate pentahydrate (0.2 mmol), and sodium ascorbate (0.2 mmol) in tetrahydrofuran (10 ml) and water (10 ml) was stirred at 25 °C for 12 h. After the filtration and vacuum concentration, residues were purified by silica gel chromatography (PE:EA = 9:1) to generate compound C1∼C8.
2.3.1. 2,3,4,5,6-Pentafluoro-N-(prop-2-yn-1-yl)benzamide (B)
White solid, yield: 79%. Mp: 83–85 °C. 1H NMR (400 MHz, DMSO-d6) δ 9.40 (s, 1H), 4.10 (dd, J = 5.5, 2.5 Hz, 2H), 3.21 (s, 1H). 13 C NMR (100 MHz, DMSO-d6) δ 156.41, 144.34, 141.87, 139.98, 138.16, 135.66, 111.95, 79.78, 73.67, 28.64. HR-MS (ESI): calcd for C10H5F5NO, [M + H]+ 250.0291; found: 250.0297. Purity: 96.82%.
2.3.2. N-((1-((2-chloropyridin-3-yl)methyl)-1H-1,2,3-triazol-4-yl)methyl)-2,3,4,5,6-pentafluorobenzamide (C1)
White solid, yield: 83%. Mp: 152–154 °C. 1H NMR (400 MHz, DMSO-d6) δ 9.44 (t, J = 5.4 Hz, 1H), 8.45 (d, J = 2.3 Hz, 1H), 8.13 (s, 1H), 7.81 (dd, J = 8.3, 2.5 Hz, 1H), 7.55 (d, J = 8.2 Hz, 1H), 5.69 (s, 2H), 4.53 (d, J = 5.6 Hz, 2H). 13 C NMR (100 MHz, DMSO-d6) δ 156.55, 150.08, 149.48, 144.01, 139.57, 131.36, 124.41, 123.34, 49.37, 34.87. HR-MS (ESI): calcd for C16H10ClF5N5O, [M + H]+ 418.0494; found: 418.0498. Purity: 97.43%.
2.3.3. N-((1-((6-chloropyridin-3-yl)methyl)-1H-1,2,3-triazol-4-yl)methyl)-2,3,4,5,6-pentafluorobenzamide (C2)
White solid, yield: 80%. Mp: 148–150 °C. 1H NMR (400 MHz, DMSO-d6) δ 9.44 (t, J = 5.4 Hz, 1H), 8.45 (d, J = 2.3 Hz, 1H), 8.14 (s, 1H), 7.81 (dd, J = 8.3, 2.5 Hz, 1H), 7.55 (d, J = 8.2 Hz, 1H), 5.69 (s, 2H), 4.53 (d, J = 5.6 Hz, 2H). 13 C NMR (100 MHz, DMSO-d6) δ 156.55, 150.08, 149.48, 144.01, 139.57, 131.35, 124.40, 123.33, 49.37, 34.87. HR-MS (ESI): calcd for C16H10ClF5N5O, [M + H]+ 418.0494; found: 418.0498. Purity: 97.57%.
2.3.4. 2,3,4,5,6-Pentafluoro-N-((1-(pyridin-4-ylmethyl)-1H-1,2,3-triazol-4-yl)methyl)benzamide (C3)
White solid, yield: 72%. Mp: 108–110 °C. 1H NMR (400 MHz, DMSO-d6) δ 9.49 (t, J = 5.4 Hz, 1H), 8.57 (d, J = 3.8 Hz, 2H), 8.16 (s, 1H), 7.21 (d, J = 5.6 Hz, 2H), 5.72 (s, 2H), 4.58 (d, J = 5.6 Hz, 2H). 13 C NMR (100 MHz, DMSO-d6) δ 156.58, 149.96, 144.90, 144.02, 123.76, 122.24, 51.44, 34.89. HR-MS (ESI): calcd for C16H11F5N5O, [M + H]+ 384.0884; found: 384.0889. Purity: 98.06%.
2.3.5. 2,3,4,5,6-Pentafluoro-N-((1-(pyridin-2-ylmethyl)-1H-1,2,3-triazol-4-yl)methyl)benzamide (C4)
White solid, yield: 88%. Mp: 149–151 °C. 1H NMR (400 MHz, DMSO-d6) δ 9.47 (t, J = 5.3 Hz, 1H), 8.55 (d, J = 4.2 Hz, 1H), 8.08 (s, 1H), 7.83 (td, J = 7.7, 1.7 Hz, 1H), 7.60 − 7.09 (m, 2H), 5.73 (s, 2H), 4.56 (d, J = 5.6 Hz, 2H). 13 C NMR (100 MHz, DMSO-d6) δ 156.55, 155.02, 149.40, 143.68, 137.27, 123.78, 123.20, 122.14, 54.34, 34.90. HR-MS (ESI): calcd for C16H11F5N5O, [M + H]+ 384.0884; found: 384.0887. Purity: 96.99%.
2.3.6. 2,3,4,5,6-Pentafluoro-N-((1-((3-methyl-4–(2,2,2-trifluoroethoxy)pyridin-2-yl)methyl)-1H-1,2,3-triazol-4-yl)methyl)benzamide (C5)
White solid, yield: 69%. Mp: 156–158 °C. 1H NMR (400 MHz, DMSO-d6) δ 9.44 (t, J = 5.5 Hz, 1H), 8.30 (d, J = 5.6 Hz, 1H), 7.92 (s, 1H), 7.13 (d, J = 5.7 Hz, 1H), 5.74 (s, 2H), 4.92 (q, J = 8.7 Hz, 2H), 4.54 (d, J = 5.6 Hz, 2H), 2.22 (s, 3H). 13 C NMR (100 MHz, DMSO-d6) δ 161.30, 156.55, 153.95, 148.03, 143.45, 123.62, 120.06, 107.33, 64.82, 64.48, 52.43, 34.90, 9.70. HR-MS (ESI): calcd for C19H14F8N5O2, [M + H]+ 496.1020; found: 496.1025. Purity: 97.43%.
2.3.7. 2,3,4,5,6-Pentafluoro-N-((1-((4-methoxy-3,5-dimethylpyridin-2-yl)methyl)-1H-1,2,3-triazol-4-yl)methyl)benzamide (C6)
White solid, yield: 62%. Mp: 135–137 °C. 1H NMR (400 MHz, DMSO-d6) δ 9.44 (t, J = 5.3 Hz, 1H), 8.18 (s, 1H), 7.90 (s, 1H), 5.69 (s, 2H), 4.53 (d, J = 5.6 Hz, 2H), 3.73 (s, 3H), 2.23 (d, J = 19.3 Hz, 6H). 13 C NMR (100 MHz, DMSO-d6) δ 163.57, 156.54, 152.66, 148.99, 143.45, 125.78, 124.66, 123.48, 59.79, 52.57, 34.91, 12.87, 10.39. HR-MS (ESI): calcd for C19H17F5N5O2, [M + H]+ 442.1302; found: 442.1307. Purity: 99.53%.
2.3.8. N-((1-((6-chloropyridin-2-yl)methyl)-1H-1,2,3-triazol-4-yl)methyl)-2,3,4,5,6-pentafluorobenzamide (C7)
White solid, yield: 88%. Mp: 148–150 °C. 1H NMR (400 MHz, DMSO-d6) δ 9.44 (t, J = 5.3 Hz, 1H), 8.45 (d, J = 2.3 Hz, 1H), 8.14 (s, 1H), 7.81 (dd, J = 8.3, 2.5 Hz, 1H), 7.55 (d, J = 8.2 Hz, 1H), 5.69 (s, 2H), 4.54 (d, J = 5.5 Hz, 2H). 13 C NMR (100 MHz, DMSO-d6) δ 156.55, 150.09, 149.47, 144.01, 139.55, 131.34, 124.39, 123.33, 49.38, 34.88. HR-MS (ESI): calcd for C16H10ClF5N5O, [M + H]+ 418.0494; found: 418.0497. Purity: 98.24%.
2.3.9. N-((1-((1H-benzo[d]imidazol-2-yl)methyl)-1H-1,2,3-triazol-4-yl)methyl)-2,3,4,5,6-pentafluorobenzamide (C8)
White solid, yield: 73%. Mp: 232–234 °C. 1H NMR (400 MHz, DMSO-d6) δ 12.68 (s, 1H), 9.46 (t, J = 5.4 Hz, 1H), 8.13 (s, 1H), 7.55 (dd, J = 35.7, 7.6 Hz, 2H), 7.34 − 6.97 (m, 2H), 5.88 (s, 2H), 4.57 (d, J = 5.6 Hz, 2H). 13 C NMR (100 MHz, DMSO-d6) δ 156.59, 148.24, 143.84, 123.66, 47.24, 34.89. HR-MS (ESI): calcd for C18H12F5N6O, [M + H]+ 423.0993; found: 423.0998. Purity: 98.15%.
2.4. In vitro enzymatic activity against FTO
Of 50 µL of buffer solution containing 2 mM L-ascorbic acid, 2 nM FTO, 2 M ssRNA, 280 µM (NH4)2Fe(SO4)2, 1 mM α-KG, and 50 mM Tris–HCl was prepared to perform the enzymatic reaction. Compounds with different concentrations were added into the solution and incubated at room temperature for 30 min. Then, the enzymatic reaction was quenched by heating at 95 °C for 5 min. ssRNA, nuclease P1, NH4OAc, NH4HCO3, and alkaline phosphatase were added and incubated at 37 °C for 3 h. Finally, the nucleosides were separated and detected using a Thermo TSQ Quantum Ultra LC/MS (Thermo Fisher Scientific, Waltham, America). Concentration-response curves were fitted with GraphPad Prism version 6.0 (GraphPad Software, San Diego, CA).
2.5. Cell proliferation assay
KYSE-150, KYSE-270, TE-1, KYSE-510, and EC109 cell lines were purchased from Shanghai Yuanye Biotechnology Co., LTD (Shanghai, China). EC109&shFTO and EC109&shControl cell lines were supported by Servicebio (Wuhan, China). All these cells were maintained in RPMI-1640 medium (Shanghai Yuanye Biotechnology Co., LTD, Shanghai, China) with 10% foetal bovine serum (Shanghai Yuanye Biotechnology Co., LTD, Shanghai, China) and 1% penicillin-streptomycin in a humidified atmosphere of 5% CO2 at 37 °C. Cells were cultured with compounds at different concentrations for 72 h. Next, 5 mg/mL MTT (Servicebio, Wuhan, China) was added and incubated for 4 h. DMSO was added into the system and shocked for 10 min. The absorbance at 490 nm was measured by using a multifunction microplate reader (Thermo Fisher Scientific, Waltham, MA).
2.6. Western blotting
RIPA buffer solution (Servicebio, Wuhan, China) was used to perform western blot. Of 20 µL of protein solution were subjected to SDS-PAGE, and then transferred to nitrocellulose membranes (Shanghai Yuanye Biotechnology Co., LTD, Shanghai, China). Of 5% non-fat milk (Servicebio, Wuhan, China) was used to block the system and nitrocellulose membranes were incubated at 4 °C with the first antibody overnight, followed by the incubation with a secondary antibody. Finally, blots were visualised by the chemiluminescence kit (Shanghai Yuanye Biotechnology Co., LTD, Shanghai, China).
2.7. Molecular docking studies
Studies of molecular modelling in this work were performed with Autodock software (The Scripps Research Institute, San Diego, CA). The crystal structure of FTO (PDB code: 5DAB) was downloaded from the RCSB protein database (http://www.rcsb.org/). Hydrogen-bond interaction between FTO and compounds was analysed by Pymol software (DeLano Scientific LLC, San Carlos, CA).
2.8. Cell cycle arrest
Cells were incubated with the targeted compound at different concentrations for 48 h and harvested. Of 70% cold ethanol was added and incubated at 4 °C for 12 h. Then, fixed cells were washed with PBS (Servicebio, Wuhan, China). Finally, the system was stained with PI (Servicebio, Wuhan, China) for 30 min under the dark condition and analysed by a Flow cytometer (Annoron, Beijing, China).
2.9. Xenograft study
BALB/c nude mice purchased from Shanghai Yuanye Biotechnology Co., LTD (Shanghai, China). All the animal experiments were performed according to approved guidelines from the ethics committee of Zhengzhou University (Approval number ZZU-2021–014). EC109 cell line was used to establish xenograft models in this work. Intragastric administration was adopted to finish the in vivo experiments. Organs (Heart, liver, spleen, lung, and kidney) from BALB/c mice for toxicity studies were fixed in 4% formaldehyde solution (Servicebio, Wuhan, China). Section of tissues was supported by Servicebio (Wuhan, China) and it was analysed by haematoxylin and eosin staining.
2.10. Statistical analysis
In order to maintain accuracy, each biological assay was repeated three times. In this work, **p < 0.01 was considered significant.
3. Results and discussion
3.1. Chemistry
Synthetic route of 1,2,3-triazole-pyridine hybrids C1∼C8 is displayed in Scheme 1. Intermediate B was obtained from the acylation reaction of prop-2-yn-1-amine with 2,3,4,5,6-pentafluorobenzoyl chloride in dichloromethane. Then, click reaction of intermediate B and appropriately substituted azide derivatives was performed to generate 1,2,3-triazole-pyridine hybrids C1 ∼ C8 in the mixed solvent system (tetrahydrofuran: water = 1: 1).
3.2. Antiproliferative activity of compound B and compound C1∼C8 against esophageal cancer
1,2,3-Triazole-pyridine hybrids C1∼C8 were evaluated for their antiproliferative activities against five esophageal cancer cell lines (KYSE-150, KYSE-270, TE-1, KYSE-510, and EC109) by the MTT assay. 5-Fluorouracil (5-Fu) was employed as the reference drug in the reported reference for the antiproliferative evaluation of 1,2,3-triazole derivativesCitation15. So, it also was used as the control to evaluate the accuracy of antiproliferative results. The antiproliferative results of compound B and 1,2,3-triazole-pyridine hybrids C1∼C8 are summarised in .
Table 1. Antiproliferative activities against esophageal cancer cell lines
As shown in , compound B without the triazole-pyridine unit displayed the weak inhibitory activity against all esophageal cancer cell lines. However, 1,2,3-triazole-pyridine hybrids C1∼C8 with the triazole-pyridine unit exhibited inhibitory activity against all esophageal cancer cell lines with IC50 values from 0.83 to 18.65 μM. These results indicated that triazole-pyridine unit might play the potential synergistic effects for inhibitory activity against esophageal cancer. Among all hybrids, compound C6 displayed the best antiproliferative activity against KYSE-150, KYSE-270, TE-1, KYSE-510, and EC109 cell lines with IC50 values of 2.17, 1.35, 0.95, 4.15, and 0.83 μM, respectively.
In order to investigate the effects of substituent groups attaching to pyridine ring for the antiproliferative activity, 1,2,3-triazole-pyridine hybrids C4∼C7 containing different substituent groups were synthesised and evaluated. Compound C4 displayed moderate inhibitory activity against TE-1 cell line with an IC50 value of 9.17 μM. Replacing the hydrogen atom with a chlorine atom (C7, 6.19 μM) led to a small increment of activity against TE-1 cancer cells. However, changing the 3,5-dimethyl-4-methoxy group (C6) to a hydrogen atom (C4) or a chlorine atom (C7) led to a decrease of activity against KYSE-150, KYSE-270, TE-1, KYSE-510, and EC109 cells. All these results illustrated that substituent groups attaching to pyridine ring played the important role for the antiproliferative activity against esophageal cancer.
Furthermore, replacing the pyridine fragment of compound C6 with 1H-benzo[d]imidazole of compound C8 led to a decrease of activity against all esophageal cancer cell lines. Compound C8 displayed the antiproliferative activity against KYSE-150, KYSE-270, TE-1, KYSE-510, and EC109 cell lines with IC50 values of 3.59, 2.04, 1.76, 5.13, and 1.66 μM, respectively. Therefore, 1,2,3-triazole-pyridine hybrid might be a promising scaffold to develop antitumor agents against esophageal cancer.
3.3. 1,2,3-Triazole-pyridine hybrids were novel FTO inhibitors
Because of potent antiproliferative activity against all esophageal cancer cell lines, compound C6 and C8 were selected to evaluate their inhibitory activity against FTO. From the results of enzymatic inhibitory activity in , compound C6 and C8, respectively, displayed the potent inhibitory effects against FTO with IC50 values of 780 and 8670 nM. These results indicated that 1,2,3-Triazole-pyridine hybrids might be potential FTO inhibitors.
Figure 2. 1,2,3-Triazole-pyridine hybrids were novel FTO inhibitors. (A) The inhibitory activity of compound C6 against FTO; (B) The inhibitory activity of compound C8 against FTO; (C) Expression of FTO in EC109&shFTO and EC109&shControl cells; (D) Cell viability of compound C6 against EC109&shFTO and EC109&shControl cells. FB23-2 as a reported FTO inhibitor was the reference molecule.

Recent references showed that FTO is aberrantly upregulated in various cancers, and down-regulation of LSD1 by RNAi or pharmacological inhibition has been an effective strategy to suppress the development of various cancerCitation16. In this work, EC109&shFTO (FTO knock-down cells) and EC109&shControl (control cells) cell lines were cultured to investigate the in vitro antiproliferative activity of FTO inhibitors. Firstly, the expression of FTO in EC109&shFTO and EC109&shControl cells was detected and the results are shown in . Then, MTT assay was performed to examine the antiproliferative activity of FTO inhibitor C6. From the results of , FTO inhibitor C6 exhibited inhibitory effects with an IC50 value of 1.06 μM against EC109&shControl cells. In contrast, compound C6 inhibited EC109&shFTO cells with an IC50 value of 3.97 μM, about 3–4 fold less potent against EC109&shControl cells. The activity discrepancy in demonstrated that antiproliferative effects of compound C6 against EC109 cells had a relationship with the FTO inhibition.
3.4. Molecular docking of FTO inhibitors
Due to different inhibitory effects of 1,2,3-Triazole-pyridine hybrids C6 and C8 against FTO, their molecular docking studies were investigated in this work. To predict the binding models between compounds and FTO, docking analysis using the Autodock software was performed. PDB code was 5DAB and FTO protein was downloaded from the RCSB protein database. As shown in , compound C8 locates into the active pocket of FTO and forms the hydrogen-bond interaction with Lys107, Leu109, and Asn235. As a reference molecule, FB23-2 was also docked using the same methods. From the results in , FB23-2 as a reported FTO inhibitor (yellow structure) was docked into a similar pocket as compound C8.
Figure 3. Molecular docking of compound C8 (PDB code: 5DAB). (A) Compound C8 binds to subunits of FTO; (B) Hydrogen-bond interaction of compound C8 and FTO; (C) Compound C8 locates into the active pocket of FTO; (D) a similar pocket between FB23-2 (yellow structure) and compound C8.

As shown in , compound C6 was docked into the active site of FTO and displayed the potent inhibitory activity against FTO. The amide group attaching to 1,2,3,4,5-pentafluorobenzene ring of 1,2,3-Triazole-pyridine hybrid C6 formed a hydrogen bond with Lys107. 1,2,3-Triazole unit of compound C6 formed two hydrogen bonds with Lys107 and Asn110 of FTO. In addition, pyridine unit of compound C6 also formed two hydrogen bonds with Tyr108 and Leu109 of FTO. From the results in , FB23-2 as a reported FTO inhibitor (red structure) was docked into a similar pocket as compound C6.
3.5. Cell cycle analysis
N6-Methyladenosine (m6A) modification is the major chemical modification in mRNA that controls cell proliferation and cell cycleCitation17. Recent studies reported that FTO demethylates Cyclin D1 mRNA and controls cell cycle progressionCitation18. In order to explore the effects of cell cycle of compound C6, TE-1 and EC109 cell lines were selected according to its antiproliferative activity results. From the cell cycle analysis of compound C6 at different concentrations against TE-1 and EC109 cells in , it arrested cell cycle at G2 phase accompanying with the decrease of cells at G1 and S phase in a concentration-dependent manner. These findings indicated that compound C6 as a novel FTO inhibitor could arrest cell cycle against esophageal cancer.
3.6. The regulation of epithelial mesenchymal transition (EMT) pathway
Epithelial mesenchymal transition (EMT) as a critical cellular programme in which epithelial cells undergo series of biochemical changes to acquire mesenchymal phenotype displays important roles in the development of esophageal cancerCitation19. EMT is characterised by the upregulation of N-cadherin and the downregulation of E-cadherin, which is regulated by a complex network of signalling pathways and transcription factorsCitation20. To further explore the anticancer mechanism of FTO inhibitor C6, TE-1, and EC109 cell lines were treated for 48 h. From the results in , the expression levels of N-cadherin and Vimentin were decreased and the expression level of E-cadherin was increased against TE-1 and EC109 cells in a concentration-dependent manner, demonstrating that FTO inhibitor C6 could inhibit EMT pathway against esophageal cancer.
3.7. The regulation of PI3K/AKT pathway
Dysregulation of FTO was implicated in multiple biological processes including proliferation and cell cycle against different tumorsCitation21. Importantly, these modulations might rely on the communications between FTO and PI3K/AKT signalling pathwayCitation22. In recent years, studies have shown that components of the PI3K/Akt signalling pathway are frequently altered in esophageal cancerCitation23. TE-1 and EC109 cell lines were treated with FTO inhibitor C6 at different concentrations for 48 h to perform western blot. From the results in , the expression levels of PI3K, p-PI3K, and p-AKT were decreased against TE-1 and EC109 cells in a concentration-dependent manner, indicating that FTO inhibitor C6 could regulate PI3K/AKT pathway against esophageal cancer.
3.8. In vivo antitumor study
Due to the potent inhibitory activity of FTO inhibitor C6 against esophageal cancer EC109 cell line, we also evaluated the in vivo anticancer effects of FTO inhibitor C6 on xenograft models bearing EC109 cells. After the treatment of FTO inhibitor C6 with the dose of 60 mg/kg, the tumour weight, the weight of mice and tumour volume were measured and recorded. From the results in , FTO inhibitor C6 inhibited tumour growth in esophageal cancer xenograft models remarkably. Weight of mice was almost unchanged in demonstrating that FTO inhibitor C6 displayed the potent anticancer effects without obvious toxicities. In addition, main organs (Heart, liver, spleen, lung, and kidney) of oesophageal cancer xenograft models were performed haematoxylin and eosin staining. As shown in , these results also suggested low global toxicities.
4. Conclusions
In summary, a novel series of 1,2,3-triazole-pyridine derivatives were synthesised according to click reaction and further evaluated for their inhibitory activity against five esophageal cancer cell lines (KYSE-150, KYSE-270, TE-1, KYSE-510, and EC109). Among them, compound C6 displayed the most potent antiproliferative activity against KYSE-150, KYSE-270, TE-1, KYSE-510, and EC109 cell lines with IC50 value of 2.17, 1.35, 0.95, 4.15, and 0.83 μM, respectively. In this work, compound C6 was identified as a novel FTO inhibitor and regulated EMT pathway and PI3K/AKT pathway against esophageal cancer. Importantly, In vivo antitumor effects of compound C6 showed that it was an orally anticancer agent with potent effects and low toxicities. Therefore, FTO might be a potential therapeutic target in esophageal cancer, and compound C6 could be a promising candidate for the drug discovery to treat esophageal cancer.
Supplemental Material
Download PDF (1 MB)Disclosure statement
No potential conflict of interest was reported by authors.
Additional information
Funding
References
- Bollschweiler E, Plum P, Mönig SP, et al. Current and future treatment options for esophageal cancer in the elderly. Expert Opin Pharmacother 2017;18:1001–10.
- Huang FL, Yu SJ. Esophageal cancer: risk factors, genetic association, and treatment. Asian J Surg 2018;41:210–5.
- Qin B, Dong M, Wang Z, et al. Long non-coding RNA CASC15 facilitates esophageal squamous cell carcinoma tumorigenesis via decreasing SIM2 stability via FTO-mediated demethylation. Oncol Rep 2021;45:1059–71.
- Cui Y, Zhang C, Ma S, et al. RNA m6A demethylase FTO-mediated epigenetic up-regulation of LINC00022 promotes tumorigenesis in esophageal squamous cell carcinoma. J Exp Clin Cancer Res 2021;40:294.
- Liu S, Huang M, Chen Z, et al. FTO promotes cell proliferation and migration in esophageal squamous cell carcinoma through up-regulation of MMP13. Exp Cell Res 2020;389:111894.
- Dobie C, Montgomery AP, Szabo R, et al. Synthesis and biological evaluation of selective phosphonate-bearing 1,2,3-triazole-linked sialyltransferase inhibitors. RSC Med Chem 2021;12:1680–9.
- Srivastava A, Aggarwal L, Jain N. One-pot sequential alkynylation and cycloaddition: regioselective construction and biological evaluation of novel benzoxazole-triazole derivatives. ACS Comb Sci 2015;17:39–48.
- Ashwini N, Garg M, Mohan CD, et al. Synthesis of 1,2-benzisoxazole tethered 1,2,3-triazoles that exhibit anticancer activity in acute myeloid leukemia cell lines by inhibiting histone deacetylases, and inducing p21 and tubulin acetylation. Bioorg Med Chem 2015;23:6157–65.
- Humphries-Bickley T, Castillo-Pichardo L, Hernandez-O’Farrill E, et al. Characterization of a dual Rac/Cdc42 inhibitor MBQ-167 in metastatic cancer. Mol Cancer Ther 2017;16:805–18.
- Sahu R, Mishra R, Kumar R, et al. Pyridine moiety: an insight into recent advances in the treatment of cancer. Mini Rev Med Chem 2022;22:248–72.
- Prakash M, Itoh Y, Fujiwara Y, et al. Identification of potent and selective inhibitors of fat mass obesity-associated protein using a fragment-merging approach. J Med Chem 2021;64:15810–24.
- Shishodia S, Demetriades M, Zhang D, et al. Structure-based design of selective fat mass and obesity associated protein (FTO) inhibitors. J Med Chem 2021;64:16609–25.
- Xu Z, Zhao SJ, Liu Y. 1,2,3-Triazole-containing hybrids as potential anticancer agents: current developments, action mechanisms and structure-activity relationships. Eur J Med Chem 2019;183:111700.
- Haranahalli K, Honda T, Ojima I. Recent progress in the strategic incorporation of fluorine into medicinally active compounds. J Fluor Chem 2019;217:29–40.
- Fu DJ, Liu YC, Yang JJ, et al. Design and synthesis of sulfonamide-1,2,3-triazole derivatives bearing a dithiocarbamate moiety as antiproliferative agents. J Chem Res 2017;41:523–5.
- Zhou LL, Xu H, Huang Y, et al. Targeting the RNA demethylase FTO for cancer therapy. RSC Chem Bio 2021;2:1352–69.
- Hirayama M, Wei FY, Chujo T, et al. FTO demethylates Cyclin D1 mRNA and controls cell-cycle progression. Cell Rep 2020;31:107464.
- Wu R, Liu Y, Yao Y, et al. FTO regulates adipogenesis by controlling cell cycle progression via m6A-YTHDF2 dependent mechanism. Biochim Biophys Acta Mol Cell Biol Lipids 2018;1863:1323–30.
- Tang Q, Lento A, Suzuki K, et al. Rab11-FIP1 mediates epithelial-mesenchymal transition and invasion in esophageal cancer. EMBO Rep 2021;22:e48351.
- Loh CY, Chai JY, Tang TF, et al. The E-cadherin and N-cadherin switch in epithelial-to-mesenchymal transition: signaling, therapeutic implications, and challenges. Cells 2019;8:1118.
- Zhang C, Zhang M, Ge S, et al. Reduced m6A modification predicts malignant phenotypes and augmented Wnt/PI3K-Akt signaling in gastric cancer. Cancer Med 2019;8:4766–81.
- Liu Y, Wang R, Zhang L, et al. The lipid metabolism gene FTO influences breast cancer cell energy metabolism via the PI3K/AKT signaling pathway. Oncol Lett 2017;13:4685–90.
- Zhang L, Tong Z, Sun Z, et al. MiR-25-3p targets PTEN to regulate the migration, invasion, and apoptosis of esophageal cancer cells via the PI3K/AKT pathway. Biosci Rep 2020;40:BSR20201901.