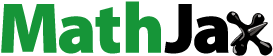
Abstract
Inhibition of c-Src is considered one of the most studied approaches to cancer treatment, with several heterocyclic compounds approved during the last 15 years as chemotherapeutic agents. Starting from the biological evaluation of an in-house collection of small molecules, indolinone was selected as the most promising scaffold. In this work, several functionalised indolinones were synthesised and their inhibitory potency and cytotoxic activity were assayed. The pharmacological profile of the most active compounds, supported by molecular modelling studies, revealed that the presence of an amino group increased the affinity towards the ATP-binding site of c-Src. At the same time, bulkier derivatizations seemed to improve the interactions within the enzymatic pocket. Overall, these data represent an early stage towards the optimisation of new, easy-to-be functionalised indolinones as potential c-Src inhibitors.
1. Introduction
c-Src is the first discovered and most studied member of the Src kinase familyCitation1. It is a cytoplasmic non-receptor protein kinaseCitation2, highly involved in intracellular signal transmission for the regulation of proliferation, migration, adhesion, invasion, and drug resistance of cancer cellsCitation3,Citation4. c-Src is a 60-kDa protein anchored to the plasmatic and endosomal membranes, as well as in the nuclear compartment, through its N-terminal tail, in which basic residues are particularly abundantCitation5. The protein structure consists of four domains: SH1, the kinase region in which the autophosphorylation site is present; SH2, which hosts the phosphorylated Tyr530 (in the C-terminal tail) and binds to PDGFR; SH3, responsible for the closed and inactive conformation of the protein; SH4, which presents the myristoylation site, essential for the membrane localisationCitation6. c-Src is constitutively inactive, with a close conformation in which SH2 and SH3 protect a phosphorylated Tyr530, preventing interactions within the catalytic site. Dephosphorylation of Tyr530 triggers the activation of c-Src, whose open conformation allows Tyr419 phosphorylation and full availability to ligand-protein interactionsCitation7,Citation8 ().
Figure 1. (a) Inactive conformation of c-Src shows phosphorylated Tyr530 on the SH2 domain; (b) Phosphorylation of Tyr419 on the kinase domain SH1 allows to activate of the enzyme.

Overexpression and/or high kinase activity of c-Src is associated with cancer progression and metastasis processes, mainly because of the reduced cell-cell adhesionCitation9 and their increased motility, migration, and invasivenessCitation10. Moreover, interaction with other overexpressed receptor tyrosine kinases (such as EGFRCitation11, PDGFRCitation12, ERBBCitation13, FGFRCitation12, and HGFCitation14) promotes the disruption of the closed conformation and exacerbates the activation of c-Src; such a condition is widely observed in glioblastomaCitation15, breastCitation16, lungCitation17, thyroidCitation18, bonesCitation19,Citation20, colonCitation21, pancreasCitation22, and prostate cancersCitation23.
In this context, many efforts have been made to design and discover new molecules acting as c-Src inhibitors, blocking its downstream pathways and impairing tumour progressionCitation24–27. To date, five molecules targeting Src have been approved by FDA (), and several others are in clinical trials for the treatment of solid tumours and leukemias, often in association with other cytotoxic agents. In particular, Dasatinib and Bosutinib have been approved for the treatment of chronic myeloid leukaemia (CML) in adult and paediatric patientsCitation28,Citation29. Saracatinib, characterised by a more selective profile in terms of Src family inhibitionCitation30, is in clinical trials for the treatment of several solid tumoursCitation24. Vandetanib and Ponatinib are multi-tyrosine kinase inhibitors, approved by FDA to treat CML, thyroid carcinoma, and Philadelphia chromosome-positive acute lymphoblastic leukaemia in adultsCitation31,Citation32. Other promising c-Src inhibitors (in phase I or II, () are DGY-06–116Citation33, eCF506Citation34, Elzovantinib (TPX-0022)Citation35, and Tirbanibulin, the latter targeting the Src substrate binding siteCitation36 and recently approved for the topical treatment of actinic keratosisCitation37.
Figure 2. Structures of the molecules approved by the FDA or in clinical trials as Src-family inhibitors.

As part of an ongoing research program aimed at identifying new chemical entities for anticancer chemotherapy, we focussed our efforts on the search of c-Src inhibitors. For this purpose, we initially screened an in-house collection library of small molecules characterised by great structural diversity. Analogues of the most promising compounds, containing the indolinone scaffold, were synthesised to highlight the structural determinants for the activity. Molecular modelling studies were also performed to propose a binding mode within the c-Src binding site.
2. Experimental
2.1. Computational details
Docking studies were performed on the three-dimensional structure of the complex between c-Src and AP23464 (entry 2BDJ of the protein data bank, 2.5 Å resolution). The structure of c-Src was prepared using the Protein Preparation Wizard of the Maestro Software Package (Schrödinger suite, version 19.2). The software corrects bond orders and partial charges of the ligands and adds hydrogens to all atoms. Water molecules were removed, except those useful for stable binding interactions with the protein or ligand. Prime software was applied to define the correct conformation of missed side chains. Pre-processed protein was optimised with PROPKA and then minimised with an OPSL3 force field (convergence of heavy atoms set to a rmsd 0.3 Å).
The 2 D structures of all synthesised compounds and native ligand AP23464 were designed using the sketcher module of the Maestro suite and prepared with the Ligprep tool, which used the Epik module to find protonation and tautomerization states of compounds at pH of 7.4 ± 0.5. To optimise the three-dimensional structures of the ligands, the Minimisation tool of the Macro model package was applied, using OPLS3 force field, while the other parameters were set to default values. Glide’s receptor grid generation wizard was used to generate a three-dimensional grid with default settings. Then, flexible docking was performed with standard precision docking mode.
2.2. Biological assays
N-Terminal His6-tagged Src was purchased from Merck-Millipore (cat. 14–326). The reaction was performed according to the manufacturer’s instructions with minor modifications: 500 μM Src-peptide (KVEKIGEGTYGVVYK), 100 μM ATP, 0.00087% NP-40, 10 ng of the enzyme, 10% DMSO in 10 μL at 30 °C for 10 min. To avoid peptide adsorption to the plastic surface, protein low-binding tubes were used. ADP-Glo Kinase Assay (Promega) was then used to detect kinase activity accordingly with manufacturer’s instruction with minor modifications. In details, reactions were transferred to white 384 well-plates and stopped by adding 10 µL of ADP-Glo Reagent (Promega) for 50 min at rt. 20 μL of Detection Reagent (Promega) was then added for 30 min and the reaction read using GloMax Discover microplate reader (Promega). Data were plotted using GraphPad Prism 5.0. ID50 values were obtained according to EquationEquation (1)(1)
(1)
(1)
(1)
where v is the measured reaction velocity, V is the apparent maximal velocity in the absence of an inhibitor, I is the inhibitor concentration, and ID50 is the 50% inhibitory dose. Compounds tested were assumed to act as fully ATP-competitive inhibitors. Therefore, Ki values were calculated accordingly to EquationEquation (2)
(2)
(2) .
(2)
(2)
where Ki is the affinity of the inhibitor to the enzyme, [S] is the substrate (namely, ATP) concentration, and Km is the affinity of ATP calculated accordingly to Michaelis-Menten equation.
Cytotoxicity experiments were carried out using the human MCF7 breast cancer cell line (ATCC HTB-22). Cells were cultured in RPMI1640 plus 10% foetal bovine serum at 37 °C and 5% CO2. Compound potency was determined by a growth inhibition assay (CellTiter 96® Aqueous One Solution Cell Proliferation Assay MTS, Promega). 24 h after seeding in a 96-well plate, cells were exposed to the compounds (concentration range 1–100 µM) and 72 h later, 20 µL of 3–(4,5-dimethylthiazol-2-yl)-5–(3-carboxymethoxyphenyl)-2–(4-sulfophenyl)-2H-tetrazolium salt (MTS) was added to each well. The absorbance was measured using a FLUOstar OPTIMA plate reader (BMG Labtech GmbH, Offenburg, Germany) at 492 nm after 4 h of incubation at 37 °C in 5% CO2. The IC50 is the drug concentration causing 50% cell growth inhibition determined by the dose-response curves. Experiments were performed in triplicate.
2.3. Chemistry
All reagents and solvents were reagent grade or were purified by standard methods before use. Melting points were determined in open capillaries by an SMP3 apparatus and are uncorrected. 1H and 13 C NMR spectra were recorded on Bruker AV600 spectrophotometer at 600 and 150 MHz, respectively. Chemical shifts are reported in ppm (δ) relative to TMS. The coupling constants, J are reported in Hertz (Hz). All compounds were routinely checked by thin layer chromatography (TLC) using precoated silica gel 60 F254, aluminium foil and the spots were detected under UV light at 254 nm and 365 nm or were revealed by spraying with 10% phosphomolybdic acid in ethanol.
Compounds 1, 2, 4Citation38, 13, 14Citation39, 15, 16Citation40, 7, 8, 23, 24Citation41, 9Citation42, 10, 11Citation43, 17Citation44, 18Citation45, 19Citation45, 25Citation46, 28Citation47 are part of an in-house library. The synthetic procedures relative to the above-mentioned products have been already described and reported in the cited references.
2.4. General procedure for preparation of compounds 22, 27, 29, 30, 32–38, 43–50
To a solution of the benzaldehyde (0.9 mmol) in EtOH (9 ml), indolinone (100 mg, 0.6 mmol) was added, followed by piperidine (0.06 mmol). After heating at reflux for 4 h, the crude was concentrated under reduced pressure and purified by flash chromatography.
1-hydroxy-3–(3-phenoxybenzylidene)indolin-2-one (22) was prepared from 1-hydroxy-3H-indol-2-oneCitation41 and 3-phenoxybenzaldehyde. Purification by column chromatography (CH2Cl2/CH3OH 97:3) gave the title compound in 85% yield, as a yellow oil. 1H NMR (600 MHz, DMSO-d6, major isomer (E), δ: 10.90 (1H, s); 7.67 (1H, s); 7.57–7.53–7.73 (1H, m); 7.48–7.41 (2H, m); 7.40–7.37 (1H, m); 7.33–7.7.28 (1H, m); 7.26 (1H, s); 7.21–7.15 (2H, m); 7.14–7.11 (2H, m); 6.95 (1H, d, J = 7.6 Hz); 6.88–6.82 (1H, m). 13 C NMR (150 MHz, DMSO-d6), major isomer (E), δ: 162.9, 157.3, 156.1, 142.4, 136.0, 135.9, 130.6, 130.4, 130.2 (× 2 C), 130.1, 124.6, 124.1, 122.1, 121.8, 119.9, 119.4 (× 2 C), 118.6, 118.3, 107.5.
3-((5-phenylthiophen-2-yl)methylene)indolin-2-one (27) was prepared from commercially available 2-oxindole and 5-phenylthiophene-2-carbaldehyde. Purification by column chromatography (CH2Cl2/CH3OH 95:5) gave the title compound in 95% yield, as an orange solid (m.p. 257–258 °C). 1H NMR (600 MHz, DMSO-d6), major isomer (E), δ: 10.63 (1H, s); 8.06 (1H, s); 7.90 (1H, d, J = 3.8 Hz); 7.79–7.73 (2H, m); 7.68 (1H, d, J = 7.7 Hz); 7.64 (1H, d, J = 3.8 Hz); 7.50–7.43 (2H, m); 7.41–7.35 (1H, m); 7.24–7.16 (1H, m); 7.02–6.97 (1H, m); 6.86 (1H, d, J = 7.6 Hz). 13 C NMR (150 MHz, DMSO-d6), major isomer (E), δ: 167.4, 150.2, 140.5, 139.0, 136.9, 133.5, 129.3 (× 2 C), 128.6, 128.5.128.0, 125.8 (× 2 C), 124.4, 124.2, 121.6, 121.0, 119.4, 109.5.
3–(3,5-dibromo-4-hydroxybenzylidene)-5,6-dimethoxyindolin-2-one (29) was prepared from commercially available 5,6-dimethoxy-2-oxindole and 3,5-dibromo-4-hydroxybenzaldehyde. Purification by column chromatography (CH2Cl2/CH3OH 95:5) gave the title compound in 52% yield, as a yellow solid (291–292 °C). 1H NMR (600 MHz, DMSO-d6), E/Z 76/24, δ: 10.52 (1H, bs); 10.36 (1H isomer Z, bs); 10.32 (1H isomer E, bs); 8.75–8.69 (2H isomer Z, m); 7.95–7.89 (2H isomer E, m); 7.52 (1H isomer Z, s); 7.34 (1H isomer Z, s); 7.28 (1H isomer E, s); 7.17 (1H isomer E, s); 6.51 (1H isomer E, s); 6.45 (1H isomer Z, s); 3.79 (3H isomer E, s); 3.77 (3H isomer Z, s); 3.76 (3H isomer Z, s); 3.64 (3H isomer E, s). 13 C-NMR (150 MHz, DMSO-d6), isomer E + Z, δ: 169.1, 167.8, 151.2 (× 2 C), 144.2 (× 2 C), 143.2 (× 2 C), 139.3, 135.6 (× 2 C), 133.1 (× 4 C), 131.5, 129.6, 128.5, 116.0, 111.8 (× 2 C), 111.4, 111.3, 107.0, 105.1, 95.5 (× 2 C), 95.0 (× 2 C), 56.3 (× 2 C), 55.7 (× 2 C).
3-([1,1′-biphenyl]-4-ylmethylene)-5,6-dimethoxyindolin-2-one (30) was prepared from 5,6-dimethoxy-2-oxindole and (1,1′-biphenyl)-4-carbaldehyde. Purification by column chromatography (CH2Cl2/CH3OH 95:5) gave the title compound an 83% yield, as a yellow solid (m.p. 231–232 °C). 1H NMR (600 MHz, DMSO-d6), major isomer (E), δ: 10.36 (1H, bs); 7.87–7.78 (4H, m); 7.78–7.72 (2H, m); 7.54–7.45 (4H, m); 7.43–7.36 (1H, m); 7.23 (1H, s); 3.79 (3H, s); 3.58 (3H, s). 13 C NMR (150 MHz, DMSO-d6), major isomer (E), δ: 169.3, 151.4, 143.2, 140.9, 139.3, 138.3, 133.8, 131.8, 130.0 (× 2 C), 129.0 (× 2 C), 127.9 (× 2 C),126.8 (× 2 C), 126.7 (× 2 C), 111.7, 107.9, 95.5.
4-((6-chloro-2-oxoindolin-3-ylidene)methyl)benzonitrile (32) was prepared from 31 and 4-cyanobenzaldehyde. Purification by column chromatography (hexane/EtOAc 90:10 → 20:80) gave the title compound in 45% yield, as a yellow solid (m.p. 310 °C, dec.). 1H NMR (600 MHz, DMSO-d6), E/Z 73/27, δ:10.88 (1H isomer Z, s); 10.86 (1H isomer E, s); 8.48–8.42 (2H isomer Z, m); 8.04–7.98 (2H isomer E, m); 7.96–7.92 (3H isomer Z); 7.91–7.86 (2H isomer E, m); 7.80–7.75 (1H isomer Z, d, J = 8.3 Hz); 7.70 (1H isomer E, s); 7.37 (1H isomer E, d, J = 8.9 Hz); 7.10 (1H isomer Z, dd, J = 8.3 Hz, 1.8 Hz); 6.96–6.90 (2H isomer E, m); 6.87 (1 H isomer Z, d, J = 1.8 Hz). 13 C NMR (150 MHz, DMSO-d6), major isomer (E), δ: 168.2, 144.7, 139.2, 134.8, 134.3, 132.7 (× 2 C), 132.0, 130.0 (× 2 C), 128.6, 124.0, 121.1, 119.3, 118.5, 110.3.
6-chloro-3–(4-nitrobenzylidene)indolin-2-one (33) was prepared from 31 and 4-nitrobenzaldehyde. Purification by column chromatography (CH2Cl2 + 0.1% Et3N, CH2Cl2/CH3OH 99:1 → 80:20, EtOAc/CH3OH 80:20) gave the title compound in 45% yield, as a red solid (m.p. 322–323 °C, dec.). 1H NMR (600 MHz, DMSO-d6), major isomer (E), δ: 10.89 (1H, s); 8.40–8.33 (2H, m); 8.00–7.92 (2H, m); 7.74 (1H, s); 7.40 (1H, d, J = 8.2 Hz); 6.95–6.90 (2H, m). 13 C NMR (150 MHz, DMSO-d6), major isomer (E), δ: 168.1, 147.6, 144.8, 141.1, 135.0, 133.8, 130.5 (× 2 C), 129.0, 124.0 (× 2 C), 123.2, 121.2, 119.2, 110.3.
6-chloro-3–(4-(dimethylamino)benzylidene)indolin-2-one (34) was prepared from 31 and 4-(dimethylamino)benzaldehyde. Purification by column chromatography (petroleum ether/EtOAc 95:5 → 20:80, CH2Cl2/CH3OH 95:5) gave the title compound an 84% yield, as an orange solid (m.p. 253–254 °C). 1H NMR (600 MHz, DMSO-d6), major isomer (E), δ: 10.62 (bs,1H); 7.78 (1H d, J = 8.3 Hz); 7.68–7.64 (2H, m); 7.57 (1H, s); 6.96 (1H, dd, J = 8.3 Hz, 1.6 Hz); 6.88 (1H, d, J = 1.6 Hz); 6.86–6.82 (2H, m); 3.02 (6H, s). 13 C NMR (150 MHz, DMSO-d6), δ: 169.8, 152.0, 143.8, 138.7, 139.9, 132.6 (× 2 C), 123.2, 121.3 (× 2 C), 121.2, 121.0, 112.1 (× 2 C), 110.1, 40.1 (× 2 C).
6-chloro-3–(4-hydroxybenzylidene)indolin-2-one (35) was prepared from 31 and 4-hydroxybenzaldehyde. Purification by column chromatography (hexane/EtOAc 90:10, CH2Cl2/CH3OH 95:5 → 90:10) gave the title compound in 94% yield, as a yellow solid (m.p. 330–331 °C, dec.). 1H NMR (600 MHz, DMSO-d6), major isomer (E), δ: 10.70 (1H, bs); 10.27 (1H, bs); 7.69 (1H, d, J = 8.2 Hz); 7.66–7.60 (2H, m); 7.59 (1H, s); 6.97–6.91 (3H, m); 6.90–6.99 (1H, m). 13 C NMR (150 MHz, DMSO-d6), isomer E + Z, δ: 169.0, 167.3, 160.5, 159.6, 143.8, 141.1, 138.5, 137.5, 135.0, 133.3, 132.0 (× 4 C), 125.4, 124.7, 124.6, 123.4, 123.2, 121.6, 120.7, 120.5, 120.4, 120.3, 115.7 (× 2 C), 115.3 (× 2 C), 109.8, 109.0.
4-((6-chloro-2-oxoindolin-3-ylidene)methyl)benzoic acid (36) was prepared from 31 and 4-formylbenzoic acid. Purification by column chromatography (hexane/EtOAc 90:10 → 75:25, CH2Cl2/CH3OH 95:5 → 80:20, EtOAc/CH3OH 80:20) gave the title compound in 22% yield, as a yellow solid (m.p. 271–272 °C, dec.). 1H NMR (600 MHz, DMSO-d6), major isomer (E), δ: 10.86 (1H, bs); 8.10–8.03 (2H, m); 7.81–7.73 (2H, m); 7.71 (1H, s); 7.48 (1H, d, J = 7.48 Hz); 6.96–6.89 (2H, m). 13 C NMR (150 MHz, DMSO-d6), major isomer (E), δ: 168.4, 168.0, 144.5, 137.5, 135.6, 134.4, 131.6, 129.7 (× 2 C), 129.1 (× 2 C), 127.5, 123.8, 121.0, 119.6, 110.2.
3–(4-(benzyloxy)benzylidene)-6-chloroindolin-2-one (37) was prepared from 31 and 4-benzyloxybenzaldehyde. Purification by column chromatography (hexane/EtOAc 90:10 → 75:25) gave the title compound in 50% yield, as a yellow solid (m.p. 239–240 °C, dec.). 1H NMR (600 MHz, DMSO-d6), E/Z 75/25, δ:10.74 (1H isomer E + 1H isomer Z, s); 8.48 (2H isomer Z, m); 7.82 (1H isomer Z, s); 7.78–7.69 (2H isomer E + 1H isomer Z, m); 7.68– 7.61 (2H, m); 7.54–7.48 (2H isomer E, m); 7.47–7.40 (2H isomer E + 2H isomer Z, m); 7.39–7.34 (2H isomer Z, m); 7.22–7.16 (2H isomer E, m); 7.16–7.11 (2H isomer Z, m); 7.07–7.02 (1H isomer Z, m); 6.95 (1H isomer E, dd, J = 8.4 Hz, 2.1 Hz); 6.90 (1H isomer E, d, J = 2.1 Hz); 6.86–6.83 (1H isomer Z, m); 5.21 (2H isomer E + 2H isomer Z, s). 13 C NMR (150 MHz, DMSO-d6), isomer E + Z, δ: 168.8, 167.2, 160.51, 59.8, 144.0, 141.3, 137.9, 136.9, 136.6, 134.6, 133.6, 132.2, 131.7 (× 2 C), 128.5 (× 6 C), 128.0 (× 2 C), 127.8 (× 4 C), 127.0, 126.6, 124.5, 124.3, 123.3, 122.9, 120.8, 120.7 (× 2 C), 120.1, 115.1 (× 2 C), 114.6 (× 2 C), 110.0, 109.1.
6-chloro-3–(3-phenoxybenzylidene)indolin-2-one (38) was prepared from 31 and 3-phenoxybenzaldehyde. Purification by column chromatography (hexane/EtOAc 90:10 + 0.1% Et3N, hexane/EtOAc 80:20 → 50:50, CH2Cl2/CH3OH 99:1 → 80:20) gave the title compound in 19% yield, as a yellow solid (m.p. 188–189 °C). 1H NMR (600 MHz, DMSO-d6), major isomer (E), δ: 10.78 (1H, bs); 7.63 (1H, s); 7.58–7.52 (1H, m); 7.49–7.41 (3H, m); 7.40–7.35 (1H, m); 7.25–7.19 (2H, m); 7.18–7.10 (3H, m); 6.91–6.82 (1H, m). 13 C NMR (150 MHz, DMSO-d6), major isomer (E), δ: 168.4, 157.3, 156.0, 144.4, 136.0, 135.7, 134.2, 130.6, 130.2 (× 2 C), 126.9, 124.4, 124.1, 123.7, 120.8, 119.8, 119.5 (× 2 C), 119.4, 118.2, 110.1.
2.4.1. Ethyl 2–(4-((6-chloro-2-oxoindolin-3-ylidene)methyl)phenoxy) acetate (43)
To a solution of 4-hydroxybenzaldehyde (100 mg, 0.82 mmol) and bromoethyl acetate (0.14 ml, 1.23 mmol) in dry acetone (3 ml), K2CO3 (170 mg, 1.64 mmol) was added, and the reaction was left stirring at reflux. After 4 h, the crude was concentrated under reduced pressure, treated with EtOAc and washed with water. The resulting organic phase was washed with brine and dried over Na2SO4, then evaporated under reduced pressure. Purification by flash chromatography (petroleum ether/EtOAc 90:10 → 85:15) afforded ethyl 2–(4-formylphenoxy)acetate 40 (150 mg, 88% yield) as a yellow oil. 1H NMR (600 MHz, DMSO-d6), δ: 9.93 (1H, s); 7.91–7.85 (2H, m); 7.07–7.02 (2H, m); 4.73 (2H, s); 4.31 (2H, q, J = 6.6 Hz); 1.33 (3H, t, J = 6.6 Hz).
Compound 43 was prepared from 31 and the above compound. Purification by column chromatography (hexane/EtOAc 90:10 → 75:25) gave the title compound in 64% yield, as a yellow solid (m.p. 158–159 °C). 1H NMR (600 MHz, DMSO-d6), E/Z 90/10, δ: 10.74 (1H isomer E, bs); 8.49–8.44 (2H isomer Z, m); 7.82 (1H isomer Z, s); 7.75–7.68 (2H isomer E, m); 7.76–7.58 (2H isomer E, m); 7.13–7.07 (2H isomer E, m); 7.06–7.03 (1H isomer Z, m); 6.94 (1H isomer E, dd, J = 1.9 Hz, 8.4 Hz); 6.90 (1H isomer E, d, J = 1.9 Hz); 6.84 (1H isomer Z, d, J = 1.8 Hz); 4.90 (2H isomer E + 2H isomer Z, s); 4.21 (2H isomer E + 2H isomer Z, q, J = 6.9 Hz); 1.24 (3H isomer E + 3H isomer Z, t, J = 6.9 Hz). 13 C NMR (150 MHz, DMSO-d6), isomer E + Z, δ: 168.8, 168.4 (× 2 C), 167.2, 159.6, 159.0, 144.0, (× 2 C), 141.4, 137.7, 136.7 (× 2 C), 134.4 (× 2 C), 133.7, 132.3, 131.5 (× 2 C), 127.4, 127.0, 124.8, 124.3, 123.3 (× 2 C), 120.8 (× 3 C), 120.0, 114.9 (× 2 C), 114.3 (× 2 C), 64.7, 64.6, 60.7 (× 2 C), 14.0 (× 2 C).
2.4.2. Methyl-4-((4-((6-chloro-2-oxoindolin-3-ylidene)methyl) phenoxy)methyl)benzoate (44)
To a solution of 4-hydroxybenzaldehyde (100 mg, 0.82 mmol) and methyl-4-bromomethyl benzoate (205 mg, 0.9 mmol) in dry DMF (3 ml), Cs2CO3 (200 mg, 0.57 mmol) was added, and the reaction was left stirring at rt. After 16 h, the crude was concentrated under reduced pressure, treated with EtOAc and washed with water. The resulting organic phase was washed with brine and dried over Na2SO4, then evaporated under reduced pressure. Purification by flash chromatography (petroleum ether/EtOAc 90:10 → 75:25) afforded methyl 4-((4-formylphenoxy)methyl)benzoate 41 (175 mg, 79% yield) as a white solid. 1H NMR (600 MHz, DMSO-d6), δ: 9.93 (1H, s); 8.14–8.08 (2H, m); 7.91–7.84 (2H, m); 7.56–7.50 (2H, m); 7.14–7.07 (2H, m); 5.24 (2H, s); 3.95 (3H, s).
Compound 44 was prepared from 31 and the above compound. Purification by column chromatography (hexane/EtOAc 90:10 → 50:50) gave the title compound in 93% yield, as a yellow solid (m.p. 201–202 °C). 1H NMR (600 MHz, DMSO-d6), E/Z 70/30, δ: 10.73 (1H isomer Z, bs); 10.71 (1H isomer E, bs); 8.49–8.43 (2H, isomer Z, m); 8.05–7.96 (2H isomer E, m); 7.79 (1H isomer Z, s); 7.74–7.66 (2H isomer E + 2H isomer Z, m); 7.65–7.58 (4H isomer E + 3H isomer Z, m); 7.19–7.15 (2H isomer E, m); 7.14–7.11 (2H isomer Z, m); 7.02 (1H isomer Z, dd, J = 1.6 Hz, 8.2 Hz); 6.92 (1H isomer E, dd, J = 1.6 Hz, 8.3 Hz); 6.87 (1H isomer E, d, J = 1.6 Hz); 6.81 (1H isomer z, d, J = 1.6 Hz); 5.29 (2H isomer E + 2H isomer Z, s); 3.85 (3H isomer E + 2H isomer Z, s). 13 C NMR (150 MHz, DMSO-d6), isomer E + Z, δ: 168.8, 167.2, 166.0 (× 2 C), 160.2, 159.6, 144.0, 142.2 (× 2 C), 141.4 137.8, 136.7, 134.6, 133.6, 131.7, 131.6 (× 2 C), 129.4 (× 6 C), 129.1 (× 2 C), 127.2 (× 4 C), 127.2, 126.8, 124.6, 124.3, 123.3, 123.1, 120.8 (× 3 C), 120.1, 115.2 (× 2 C), 114.6 (× 2 C), 110.0, 109.1, 68.8 (× 2 C), 52.1 (× 2 C).
2.4.3. 6-Chloro-3–(4-(cyclopropylmethoxy)benzylidene)indolin-2-one (45)
To a solution of 4-hydroxybenzaldehyde (100 mg, 0.82 mmol) and bromomethyl cyclopropane (0.16 ml, 1.64 mmol) in dry acetone (3 ml), K2CO3 (450 mg, 3.28 mmol) was added, and the reaction was left stirring at reflux. After 16 h, the crude was concentrated under reduced pressure, treated with EtOAc and washed with water. The resulting organic phase was washed with brine and dried over Na2SO4, then evaporated under reduced pressure. Purification by flash chromatography (petroleum ether/EtOAc 95:5 → 90:10) afforded compound 42 (110 mg, 76% yield) as a colourless oil. 1H NMR (600 MHz, DMSO-d6), δ: 9.90 (1H, s); 7.93–7.81 (2H, m); 7.06–6.95 (2H, m); 3.92 (2H, d, J = 6.9 Hz); 1.37–1.26 (1H, m); 0.77–0.62 (2H, m); 0.50–0.34 (2H, m).
Compound 45 was prepared from 31 and the above compound. Purification by column chromatography (hexane/EtOAc 90:10 → 70:30) gave the title compound in 47% yield, as a yellow solid (m.p. 231–232 °C). 1H NMR (600 MHz, DMSO-d6), isomer E, δ: 10.74 (1H, bs), 8.42–8.50 (2H, m); 7.81 (1H, s); 7.71 (1H, d, J = 8.1 Hz); 7.10–6.99 (3H, m), 6.81 (1H, s); 3.93 (2H, d, J = 6.7 Hz); 1.32–1.22 (1H, m); 0.67–0.53 (2H, m); 0.42–0.31 (2H, m). 13 C NMR (150 MHz, DMSO-d6), major isomer (E), δ: 167.2, 160.9, 141.3, 138.0, 134.6 (× 2 C), 132.1, 131.6, 126.7, 124.4, 122.7, 120.6, 114.2 (× 2 C), 109.1, 72.3, 10.0, 3.1 (× 2 C).
2.4.4. 2–(4-((6-Chloro-2-oxoindolin-3-ylidene)methyl)phenoxy)acetic acid (46)
To a solution of compound 43 (60 mg, 0.17 mmol) in THF/water 2:1 (6 ml), LiOH monohydrate (18 mg, 0.42 mmol) was added and the reaction was left stirring at rt. After 16 h, the mixture was concentrated under reduced pressure and extracted twice with Et2O. The resulting aqueous phase was treated with HCl 37% until pH = 2, then extracted three times with EtOAc, washed with brine, dried over Na2SO4, then evaporated under reduced pressure to afford 40 mg of 46 in 71% yield, as an orange solid (m.p. 197 °C, dec.). 1H NMR (600 MHz, DMSO-d6), E/Z 74/26, δ: 13.08 (1H isomer E + 1H isomer Z, bs); 10.75 (1H isomer Z, bs); 10.73 (1H isomer E, bs); 7.90–7.86 (2H isomer Z, m); 7.82 (1H isomer Z, s); 7.74–7.68 (2H, isomer Z, m); 7.66–7.61 (1H isomer E + 1H isomer Z, m); 7.48 (1H, d, J = 8.3 Hz); 7.11–7.15 (2H isomer Z, m); 7.10–7.06 (2H isomer E + 1H isomer Z, m); 6.97–6.93 (1H isomer E + 1H isomer Z); 6.90 (1H isomer E, d, J = 2.2 Hz); 4.84 (2H isomer Z, s); 4.80 (2H isomer E, s). 13 C NMR (150 MHz, DMSO-d6), major isomer (E), δ: 169.9, 168.8, 159.2, 144.0, 136.7, 133.7, 131.6 (× 2 C), 126.8, 124.6, 123.3, 120.8, 120.0, 114.8, 110.0, 64.6.
4-((4-((6-chloro-2-oxoindolin-3-ylidene)methyl)phenoxy)methyl)benzoic acid (47). To a solution of compound 44 (70 mg, 0.17 mmol) in THF/water 2:1 (6 ml), LiOH monohydrate (18 mg, 0.42 mmol) was added and the reaction was left stirring at rt. After 16 h, the mixture was concentrated under reduced pressure and extracted twice with Et2O. The resulting aqueous phase was treated with HCl 37% until pH = 2, then extracted three times with EtOAc, washed with brine, dried over Na2SO4, then evaporated under reduced pressure to afford 60 mg of 47 in 87% yield, as a yellow solid (m.p. 279–280 °C). 1H NMR (600 MHz, DMSO-d6), major isomer (E), δ: 13.08 (1H, bs); 10.73 (1H, bs); 8.04–7.96 (2H, m); 7.77–7.71 (2H, m); 7.66–7.56 (4H, m); 7.22–7.17 (2H, m); 6.94 (1H, dd, J = 1.4 Hz, 8.6 Hz); 6.90 (1H, d, J = 1.4 Hz); 5.32 (2H, s). 13 C NMR (150 MHz, DMSO-d6), major isomer (E), δ: 168.8, 167.0, 159.6, 144.0, 141.7, 136.8, 133.6, 131.8 (× 2 C), 130.3, 129.5 (× 2 C), 127.5 (× 2 C), 126.7, 124.6, 123.3, 120.8, 120.1, 110.0, 69.0.
6-chloro-3-((5-phenylthiophen-2-yl)methylene)indolin-2-one (48) was prepared from 31 and 5-phenylthiophene-2-carbaldehyde. Purification by column chromatography (hexane/EtOAc 90:10, CH2Cl2/CH3OH 95:5 → 90:10) gave the title compound 98% yield, as a red solid (m.p. 233–234 °C). 1H NMR (600 MHz, DMSO-d6), major isomer (E), δ: 10.80 (1H, bs); 8.18 (1H, s); 7.93 (1H, d, J = 3.4 Hz); 7.81–7.76 (2H, m); 7.72 (1H, d, J = 8.3 Hz); 7.68 (1H, d, J = 3.4 Hz); 7.53–7.47 (2H, m); 7.44–7.38 (1H, m); 7.07 (1H, dd, J = 8.3 Hz, 1.9 Hz); 6.89 (1H, d, J = 1.9 Hz). 13 C NMR (150 MHz, DMSO-d6), major isomer (E), δ: 167.2, 150.7, 141.5, 139.5, 136.7, 133.4, 132.4, 129.3 (× 2 C), 129.0, 128.7, 125.8 (× 2 C), 124.3, 123.4, 120.8 (× 2 C), 120.3, 109.4.
3-((2-bromothiazol-5-yl)methylene)-6-chloroindolin-2-one (49) was prepared from 31 and 2-bromo-5-formylthiazole. Purification by column chromatography (petroleum ether/EtOAc 95:5 → 10:90, CH2Cl2/CH3OH 95:5 → 85:15) gave the title compound an 81% yield, as a red solid (m.p. 262–263 °C, dec.). 1H NMR (600 MHz, DMSO-d6), major isomer (E), δ: 10.99 (bs, 1H); 8.23 (2H, s); 7.71 (1H, d, J = 8.4 Hz); 7.11 (1H, dd, J = 8.4 Hz, 1.9 Hz); 6.92 (1H, d, J = 1.9 Hz). 13 C NMR (150 MHz, DMSO-d6), major isomer (E), δ: 167.4, 151.2, 143.7, 142.1, 135.6, 133.5, 124.8, 123.0, 122.3, 121.5, 121.3, 109.9.
3-((1H-indol-5-yl)methylene)-6-chloroindolin-2-one (50) was prepared from 31 and 1H-indole-5-carbaldehyde. Purification by column chromatography (hexane/EtOAc 95:5 → 20:80, CH2Cl2:CH3OH 95:5 → 90:10) gave the title compound in 34% yield, as a yellow solid (m.p. 238–239 °C). 1H NMR (600 MHz, DMSO-d6), major isomer (E), δ: 11.46 (1H, bs); 10.70 (1H, s); 7.98 (1H, s); 7.82 (1H, s); 7.77 (1H, d, J = 8.1 Hz); 7.55 (1H, d, J = 8.6 Hz); 7.51 (1H, d, J = 8.6 Hz); 7.49–7.46 (1H, m); 6.96–6.92 (1H, m); 6.92–6.88 (1H, m). 13 C NMR (150 MHz, DMSO-d6), major isomer (E), δ: 169.1, 152.5, 143.8, 139.6, 136.8, 133.2, 127.8, 127.0124.8, 123.4, 122.9 (× 3 C), 120.7, 120.5, 111.8, 109.8, 102.1.
3. Results and discussion
3.1. Synthesis and biological activity evaluation
A small collection of molecules containing highly diverse chemical scaffolds was initially screened on c-Src to evaluate their inhibiting activity at a 100 μM concentration (). Triazolo-triazine 13 showed the best inhibition potency (90%). However, its closely related analogue 14 did not affect the enzyme activity. Indolinone and N-hydroxyl indolinone derivatives 7–9 exhibited a significant activity (percent inhibition ranging from 77 to 84%). A modest inhibition profile was highlighted for azaindole 1 and kynurenic derivative 17 (about 58%), followed by thiazolylbenzoquinone 10 (43%) and dihydroquinolin-2-one 12 (40%), whereas all the other entries of the library showed low to no activity towards c-Src. ID50 of both triazolo-triazine 13 and indolinone 8 were in the two-digit micromolar concentration (50 and 12.5 µM, respectively).
Table 1. Evaluation of the inhibitory activity of c-Src by the in-house prepared collection of small molecules.
These results prompted us to focus on the indolinone core for further investigations. This skeleton can be considered a very attractive target for biological activity evaluation and SAR studies, offering the possibility of easy chemical accessibility and several points for chemical decoration. We thus prepared and tested a second-generation set of compounds containing the indolinone scaffold substituted at positions 5 and/or 6 and bearing an arylidene side chain appended to position 3. We also prepared 24 to evaluate the role of the heteroaromatic system and both 22 and 23 to investigate the effect of the OH group on the nitrogen atom. All the compounds showed a good dose-dependent activity at 100 μM and 10 μM concentrations, with 26 having the best inhibitory potency of the series (95 and 67% at 100 and 10 μM, respectively) ().
Table 2. Inhibitory activity evaluation of indolinones 22–30.
Compound 26 was prioritised and its structure was used as the starting point to prepare new analogues, maintaining unaltered the 6-chloro oxindole moiety and introducing decorations on the aromatic ring connected by a methylene bridge to C3. Knoevenagel condensation conditions were exploited to react 6-chloro oxindole 31 with substituted aromatic/heteroaromatic aldehydes (Scheme 1), obtaining 15 new variously substituted derivatives.
Scheme 1. General synthesis by Knoevenagel condensation starting from 6-chloro oxindole and different aromatic and heteroaromatic compounds.

All the analogues contained a phenyl ring connected by a conjugated vinyl fragment to C3. Various substituents with different stereoelectronic properties were introduced in the para position of the ring: cyano (32), nitro (33), dimethylamino (34), phenolic (35) and carboxylic (36) groups. Commercially available p-benzyloxy benzaldehyde and m-phenoxy benzaldehyde were employed for the preparation of 37 and 38. Additionally, alkylation of 4-hydroxy benzaldehyde 39 as in 43–47 gave the opportunity to increase the overall compound length and eventually fill some empty regions of the cavity, either in presence of aromatic or aliphatic moieties, as well as with a terminal acidic or ester portion. Finally, compounds containing heterocyclic substituents were synthesised (48–50) to investigate their potential role as hydrogen bond acceptors/donors.
All the obtained compounds were characterised by NMR analysis, showing the presence of isomeric mixtures (from 9:1 to 7:3 E/Z isomers). The relative ratios of the E and Z stereoisomers within this series (32–50) were assigned based on the chemical shifts of protons at the C2’ and C6’ positionsCitation41. Previous investigations on 3-arylideneoxindoles have reported the E/Z isomerisation to be solvent-, temperature-, time-, and light-dependentCitation48–50. For this reason, the compounds were tested as mixtures of the two isomers.
The percentage of inhibition at the concentrations of 100 μM and 10 μM, ID50 of the most active compounds, and their Ki were evaluated and reported in . Compounds 32–34 showed a good inhibitory activity towards the enzyme, with 34 having the best profile of inhibition (85% at 10 µM). Conversely, 36, with a carboxylic group directly linked to the phenyl ring, had very low activity. However, the percentage of inhibition was enhanced by increasing the length of the spacer between the aromatic ring and the acidic group (46, 70% at 100 µM, 47 94% at 100 µM), with the corresponding esters (43 and 44) showing a good activity as well. Replacement of the acidic group of 46 with a hydrophobic cyclopropyl substituent led to an almost inactive compound (45). Moreover, the phenolic derivative 35 and the corresponding benzyl ether 37 maintained high inhibitory activity at the highest dose, which significantly decreased at 10 µM. The shift of a lipophilic aromatic ring to meta position on the phenyl group (38) did not influence the activity. Among compounds containing heterocyclic rings, 50 showed the best activity at both concentrations, whereas the thiophene- and thiazole-containing compounds 48 and 49, respectively, evidenced a drop in activity, in particular at the lowest concentration.
Table 3. Evaluation of the inhibitory activity of the novel indolinone derivatives.
Compound 34 resulted to be the best of the series, showing significant inhibition of the enzyme at 10 µM (85%), with ID50 and Ki in the sub-micromolar range (0.71 and 0.48 µM, respectively). These findings suggest that the presence of an anilino nitrogen could have an important role in the inhibitory activity of the compounds.
With these results in our hands, the cytotoxicity test of selected molecules was carried out on the MCF-7 breast cancer cell line and compared to the c-Src inhibitor Dasatinib used as standard (). Thiazole 49 showed potency comparable to Dasatinib. Ester 43 and indole derivative 50 were able to exert some relevant but not excellent cytotoxic activity. It is worth noting that 34, the best inhibitor in the cell-free assay, did not show any cytotoxic activity, probably due to pharmacokinetic issues.
Table 4. Cytotoxicity evaluation of selected compounds on human MCF-7 breast cancer cell line.
3.2. Molecular docking studies
In the attempt to find a putative binding mode of the new indolinone derivatives within the ATP-binding site of c-Src, molecular docking simulations were performed using the software Glide. For this purpose, the three-dimensional structure of the co-crystallized complex between c-Src and AP23464 (entry 2BDJ of the protein data bank, 2.50 Å resolution) was used for calculations. Preliminary validation of the docking protocol was done by re-docking AP23464 into the binding site. The resulting rmsd = 0.903 Å, which was calculated on the atomic coordinates of the inhibitor, confirmed the reliability of the docking protocol. Two highly conserved hydrogen bonds were found between N7 and aniline nitrogen of AP23464 with the amide nitrogen and the carbonyl of the backbone of the Met341, respectively (). The 3-hydroxyphenylethyl group in position N9 on the purine scaffold protruded into the hydrophobic pocket and mediated a series of interactions. Specifically, the hydroxyl substituent formed hydrogen bonds with the carboxyl group of the Glu310 side chain and the backbone NH group of Asp404.
Figure 3. Graphical representation of the best-scored binding pose of 33 (magenta) within the c-Src ATP binding site, in comparison to the co-crystallized inhibitor AP23464 (green). Yellow dashed lines represent hydrogen bonds between Met341, Glu310, Asp404, and both ligands.

Considering that the new compounds were assayed as E/Z isomers, isomerisation was allowed during calculations. Docking scores showed that for each compound, the E-isomer was preferred to the Z-isomer. Overall, the indolinone derivatives showed a common binding pose with the lactam moiety that mimicked the hydrogen bond acceptor-donor motif represented by the N9 and the anilino NH group of AP23464. They served as hydrogen bond acceptor-donor system to give the classical interactions with Met341 of the hinge region (). Moreover, the chlorophenyl moiety was superimposable to the cyclopentyl ring of AP23464. Finally, the aryl appendage at C3 could mimic the phenol portion of the co-crystallized inhibitor. As an example, the predicted binding mode of 33, which showed single-digit micromolar ID50 and Ki, was able to maintain the two hydrogen bonds with Met341 in its best-scored pose (). In addition, one of the oxygen atoms of the nitro group matched the phenol oxygen of AP23464, thus mimicking its ability to make an additional hydrogen bond with Asp404. A very similar binding mode was found for 50, where the NH group of the pendant indole nucleus was able to build a hydrogen bond with the side chain of Glu310, in addition to the classical interactions with Met341 (Figure S1). On the other hand, molecular docking and scoring were not able to justify the binding affinity of 34, whose peripheral dimethylanilino moiety gave hydrophobic interactions with Ala403, whereas other interactions were not highlighted, in addition to the classical contacts with Met341 (Figure S2).
Increasing the length and bulkiness of the substituent at the pendant phenyl ring forced the terminal molecular edge outside the hydrophobic side pocket where the phenol ring of AP23464 was accommodated. As an example, the benzoic carboxyl group of the long side chain of 47, which retained the two hydrogen bonds with Met341, made two additional hydrogen bonds with Cys277 and Phe278. On the contrary, the docking pose of the corresponding methyl ester 44 lacked the fundamental anchor points on the binding site to avoid steric clashes due to the terminal methyl group of the ester function.
4. Conclusion
An in-house library of structurally diverse small molecules was screened with the aim of finding new c-Src inhibitors. It emerged that the most active compounds contained an indolinone core, which was selected as a promising scaffold for further investigations. Variously substituted 3-(hetero)arylideneindolin-2-ones were designed and synthesised to highlight the structural determinants for the activity. Most of the compounds showed a percentage of inhibition of the enzyme >70% at 100 μM and some of them also had a significant activity at 10 μM. Selected molecules were assayed on the human MCF-7 breast cancer cell line, showing moderate antiproliferative activity. Among the tested compounds, 49 demonstrated cytotoxicity comparable to that of the reference compound Dasatinib, but lacked any significant activity in the cell-based assay, indicating the possibility that some off-target effect could take place. Conversely, 34, which showed the best inhibition profile towards the isolated enzyme, resulted ineffective (IC50>100 μM) on the cell line. This finding likely depends on pharmacokinetic issues probably related to the difficulty of the compound to reach the intracellular target. Therefore, further studies will be necessary to improve the pharmacological profile of this class of compounds. The results of molecular docking studies, which were performed to propose a binding mode within the c-Src binding site, will guide and complement future activities.
Supplemental Material
Download PDF (1.5 MB)Acknowledgements
We thank AIRC Foundation for Cancer Research in Italy (Fondazione AIRC per la Ricerca sul Cancro, AIRC): Grant IG-2017, project code 20762, PI Giovanni Maga iii) Grant IG-2020, project code 24448, PI Emmanuele Crespan; E.C. wishes to thank Cariplo Foundation, project 2019–1836.
Disclosure statement
No potential conflict of interest was reported by the author(s).
Correction Statement
This article was originally published with errors, which have now been corrected in the online version. Please see Correction (http://dx.doi.org/[10.1080/14756366.2022.2133503)
Additional information
Funding
References
- Simatou A, Simatos G, Goulielmaki M, et al. Historical retrospective of the SRC oncogene and new perspectives. Mol Clin Oncol 2020;13:1–10.
- Parsons SJ, Parsons JT. Src family kinases, key regulators of signal transduction. Oncogene 2004;23:7906–9.
- Brown MT, Cooper JA. Regulation, substrates and functions of src. Biochimica et Biophysica Acta (BBA) – Reviews on Cancer 1996;1287:121–49.
- Playford MP, Schaller MD. The interplay between Src and integrins in normal and tumor biology. Oncogene 2004;23:7928–46.
- Sigal CT, Zhou W, Buser CA, et al. Amino-terminal basic residues of Src mediate membrane binding through electrostatic interaction with acidic phospholipids. Proc Natl Acad Sci USA 1994;91:12253–7.
- Boggon TJ, Eck MJ. Structure and regulation of Src family kinases. Oncogene 2004;23:7918–27.
- Amata I, Maffei M, Pons M. Phosphorylation of unique domains of Src family kinases. Front Genet 2014;5:181.
- Roskoski R. Src kinase regulation by phosphorylation and dephosphorylation. Biochem Biophys Res Commun 2005;331:1–14.
- McLachlan RW, Kraemer A, Helwani FM, et al. E-cadherin adhesion activates c-Src signaling at cell–cell contacts. Mol Biol Cell 2007;18:3214–23.
- Patel A, Sabbineni H, Clarke A, Somanath PR. Novel roles of Src in cancer cell epithelial-to-mesenchymal transition, vascular permeability, microinvasion and metastasis. Life Sci 2016;157:52–61.
- Maa MC, Leu TH, McCarley DJ, et al. Potentiation of epidermal growth factor receptor-mediated oncogenesis by c-Src: implications for the etiology of multiple human cancers. Proc Natl Acad Sci 1995;92:6981–5.
- Shah K, Vincent F. Divergent roles of c-src in controlling platelet-derived growth factor-dependent signaling in fibroblasts. Mol Biol Cell 2005;16:5418–32.
- Ishizawar RC, Miyake T, Parsons SJ. c-Src modulates ErbB2 and ErbB3 heterocomplex formation and function. Oncogene 2007;26:3503–10.
- Rahimi N, Hung W, Tremblay E, et al. c-Src kinase activity is required for hepatocyte growth factor-induced motility and anchorage-independent growth of mammary carcinoma cells*. J Biol Chem 1998;273:33714–21.
- Calgani A, Vignaroli G, Zamperini C, et al. Suppression of SRC signaling is effective in reducing synergy between glioblastoma and stromal cells. Mol Cancer Ther 2016;15:1535–44.
- González L, Agulló-Ortuño MT, García-Martínez JM, et al. Role of c-Src in human MCF7 breast cancer cell tumorigenesis*. J Biol Chem 2006;281:20851–64.
- Zhang J, Kalyankrishna S, Wislez M, et al. Src-family kinases are activated in non-small cell lung cancer and promote the survival of epidermal growth factor receptor-dependent cell lines. Am J Pathol 2007;170:366–76.
- Schweppe RE, Kerege AA, French JD, et al. Inhibition of Src with AZD0530 reveals the Src-focal adhesion kinase complex as a novel therapeutic target in papillary and anaplastic thyroid cancer. J Clin Endocrinol Metabolism 2009;94:2199–203.
- Lowe C, Yoneda T, Boyce BF, et al. Osteopetrosis in Src-deficient mice is due to an autonomous defect of osteoclasts. Proc Natl Acad Sci 1993;90:4485–9.
- Rucci N, Susa M, Teti A. Inhibition of protein kinase c-Src as a therapeutic approach for cancer and bone metastases. Anti-Cancer Agents Med Chem 2008;8:342–9.
- Jin W. Regulation of Src family kinases during colorectal cancer development and its clinical implications. Cancers 2020;12:1339.
- Lutz MP, Eßer IBS, Flossmann-Kast BBM, et al. Overexpression and activation of the tyrosine kinase Src in human pancreatic carcinoma. Biochem Biophys Res Commun 1998;243:503–8.
- Varkaris A, Katsiampoura AD, Araujo JC, et al. Src signaling pathways in prostate cancer. Cancer Metastasis Rev 2014;33:595–606.
- Belli S, Esposito D, Servetto A, et al. c-Src and EGFR inhibition in molecular cancer therapy: what else can we improve? Cancers. 2020;12:1489.
- Rivera-Torres J, San José E. Src tyrosine kinase inhibitors: new perspectives on their immune, antiviral, and senotherapeutic potential. Front Pharmacol 2019;10:1011.
- Musumeci F, Brullo C, Grossi G, et al. Identification of new pyrrolo[2,3-d]pyrimidines as Src tyrosine kinase inhibitors in vitro active against Glioblastoma. Eur J Med Chem 2017;127:369–78.
- Ceccherini E, Indovina P, Zamperini C, et al. SRC family kinase inhibition through a new pyrazolo[3,4- d] pyrimidine derivative as a feasible approach for glioblastoma treatment. J Cell Biochem 2015;116:856–63.
- Gnoni A, Marech I, Silvestris N, et al. Dasatinib: an anti-tumour agent via Src inhibition. Current Drug Targets 2011;12:563–78.
- Steinbach A, Clark SM, Clemmons AB. Bosutinib: a novel src/abl kinase inhibitor for chronic myelogenous leukemia. J Adv Pract Oncol 2013;4:451–5.
- Hennequin LF, Allen J, Breed J, et al. N-(5-Chloro-1,3-benzodioxol-4-yl)-7-[2-(4-methylpiperazin-1-yl)ethoxy]-5- (tetrahydro-2H-pyran-4-yloxy)quinazolin-4-amine, a novel, highly selective, orally available, dual-specific c-Src/Abl kinase inhibitor. J Med Chem 2006;49:6465–88.
- Cooper MR, Yi SY, Alghamdi W, et al. Vandetanib for the treatment of medullary thyroid carcinoma. Ann Pharmacother 2014;48:387–94.
- Tanimura K, Yamasaki K, Okuhiro Y, et al. Monitoring ponatinib in a child with philadelphia chromosome-positive acute lymphoblastic leukemia. Case Rep Oncol 2021;14:24–8.
- Gurbani D, Du G, Henning NJ, et al. Structure and characterization of a covalent inhibitor of Src kinase. Front Mol Biosci 2020;7:81–9.
- Temps C, Lietha D, Webb ER, et al. A conformation selective mode of inhibiting SRC improves drug efficacy and tolerability. Cancer Res 2021;81:5438–50.
- Hong DS, Sen S, Park H, et al. A phase I, open-label, multicenter, first-in-human study of the safety, tolerability, pharmacokinetics, and antitumor activity of TPX-0022, a novel MET/CSF1R/SRC inhibitor, in patients with advanced solid tumors harboring genetic alterations in MET. J Clin Oncol 2020;38:TPS3663–TPS3663.
- Wang X, Ren R, Xu Z, et al. Tirbanibulin attenuates pulmonary fibrosis by modulating Src/STAT3 signaling. Front Pharmacol 2021;12:693906.
- Markham A, Duggan S. Tirbanibulin: first approval. Drugs 2021;81:509–13.
- Cincinelli R, Musso L, Merlini L, et al. 7-Azaindole-1-carboxamides as a new class of PARP-1 inhibitors. Bioorg Med Chem 2014;22:1089–103.
- Aiwale TS, Dallavalle S. Efficient synthesis of 3,7-Diaryl-1,4-dihydro[1,2,4]triazolo[5,1-c][1,2,4]triazines. Synthesis 2012;44:3055–8.
- Cincinelli R, Cassinelli G, Dallavalle S, et al. Synthesis, modeling, and RET protein kinase inhibitory activity of 3- and 4-Substituted β-Carbolin-1-ones. J Med Chem 2008;51:7777–87.
- Musso L, Cincinelli R, Zuco V, et al. 3-Arylidene-N-hydroxyoxindoles: a new class of compounds endowed with antitumor activity. ChemMedChem 2016;11:1700–4.
- Rizzi E, Cassinelli G, Dallavalle S, et al. Synthesis and RET protein kinase inhibitory activity of 3-arylureidobenzylidene-indolin-2-ones. Bioorg Med Chem Lett 2007;17:3962–8.
- Cautain B, Castillo F, Musso L, et al. Discovery of a novel, isothiazolonaphthoquinone-based small molecule activator of FOXO nuclear-cytoplasmic shuttling. PLOS One 2016;11:e0167491.
- Cincinelli R, Musso L, Beretta G, Dallavalle S. 4-Quinolone fused heterocyclic ring systems by intramolecular reactions of 4-quinolone-2-carboxamides. Tetrahedron 2014;70:9797–804.
- Musso L, Cincinelli R, Giannini G, et al. Synthesis of 5,6-dihydro-4H-benzo[d]isoxazol-7-one and 5,6-dihydro-4H-isoxazolo[5,4-c]pyridin-7-one Derivatives as Potential Hsp90 Inhibitors. Chemical Biology & Drug Design 2015;86:1030–5.
- Höttecke N, Liebeck M, Baumann K, et al. Inhibition of γ-secretase by the CK1 inhibitor IC261 does not depend on CK1δ. Bioorg Med Chem Lett 2010;20:2958–63.
- la Pietra V, Sartini S, Botta L, et al. Challenging clinically unresponsive medullary thyroid cancer: discovery and pharmacological activity of novel RET inhibitors. Eur J Med Chem 2018;150:491–505.
- Chu W, Zhou D, Gaba V, et al. Design, synthesis, and characterization of 3-(Benzylidene)indolin-2-one derivatives as ligands for α-synuclein fibrils. J Med Chem 2015;58:6002–17.
- Ngai MH, So CL, Sullivan MB, et al. Photoinduced isomerization and hepatoxicities of semaxanib, sunitinib and related 3-substituted indolin-2-ones. ChemMedChem 2016;11:72–80.
- Chen X, Yang T, Deivasigamani A, et al. N′-Alkylaminosulfonyl analogues of 6-fluorobenzylideneindolinones with desirable physicochemical profiles and potent growth inhibitory activities on hepatocellular carcinoma. ChemMedChem 2015;10:1548–58.