Abstract
Human respiratory syncytial virus (RSV) is a leading cause of lower respiratory tract infection worldwide. Until now, there are no licenced vaccines or effective antiviral drugs against RSV infections. In our previous work, we found 2-((1H-indol-3-yl)thio/sulfinyl)-N-pheny acetamide derivatives (4-49 C and 1-HB-63) being a novel inhibitor against RSV in vitro. Here, we explored the underlying mechanism of 2-((1H-indol-3-yl)thio/sulfinyl)-N-pheny acetamide derivatives to inhibit RSV replication in vitro and disclosed that 4–49 C worked as the inhibitor of membrane fusion and 1-HB-63 functioned at the stage of RSV genome replication/transcription. Yet, both of them could not inhibit RSV infection of BALB/c mice by using RSV-Luc, in vivo imaging and RT-qPCR analyses, for which it may be due to the fast metabolism in vivo. Our work suggests that further structural modification and optimisation of 2-((1H-indol-3-yl) thio/sulfinyl)-N-pheny acetamide derivative are needed to obtain drug candidates with effective anti-RSV activities in vivo.
Graphical Abstract
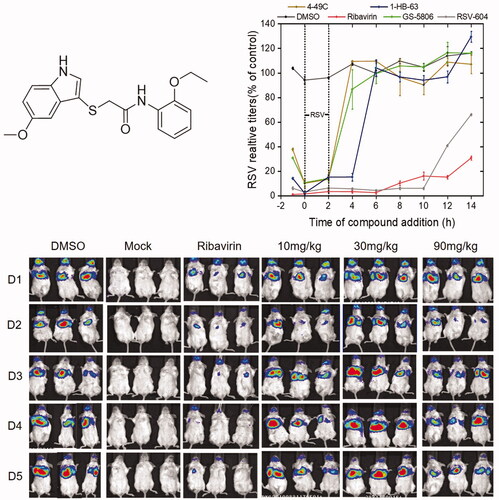
Introduction
Human respiratory syncytial virus (RSV) is an enveloped, negative-sense, single-stranded RNA virus and belongs to the Pneumovirus family and the Orthopneumovirus genusCitation1–4. It is 15.2 kb in length and contains 10 genes encoding 11 proteins, NS1, NS2, N, P, M, SH, G, F, M2-1, M2-2, and LCitation5–8. RSV is a leading cause of lower respiratory tract infection in nearly all children by 2-year-old globally and it was estimated that there were 3.2 million hospitalised cases and about 60,000 deaths annuallyCitation9–12. RSV is also an important pathogen of severe lower respiratory tract disease in the elderly and adults with immunodeficiency disordersCitation13,Citation14. Unfortunately, there is no safe and effective vaccine licenced against RSV infection. Only ribavirin and palivizumab are the available drugs for the treatment of RSV infectionCitation15–18. Yet, being a broad-spectrum antiviral drug, ribavirin is not recommended due to its poor efficacy and side effects. Palivizumab, a costly humanised monoclonal antibody for RSV, can only be used to prevent RSV infection for the young and high-risk children born prematurely, with chronic lung disease or congenital heart diseaseCitation19–21. Therefore, the development of safe and effective anti RSV drugs has important clinical significanceCitation22–24.
In our previous work, we established an rRSV-mGFP high-throughput screening platform for the potential compounds active against RSVCitation25, from which 29 compounds were identified as potent RSV inhibitors and two of them were selected for further structural optimisation following the evaluation of their toxicity, druggability and feasibility of synthesisCitation26. Among the developed 35 derivatives, 4–49 C and 1-HB-63, both of the derivatives of 2-((1H-indol-3-yl)thio/sulfinyl)-N-pheny acetamide, exhibited excellent activity against RSV and IAVCitation26. In this paper, we investigated the underlying mechanism in vitro and pharmacodynamics in vivo of the two 2-((1H-indol-3-yl)thio/sulfinyl)-N-pheny acetamide derivatives as inhibitor against RSV.
Materials and methods
Cells and viruses
HEp-2 cell and wild-type subgroup A RSV Long strain (wtRSV) were purchased from ATCC (Rockefeller, MD, USA). BHK-T7 was a gift from Prof. W. Y. Zhu (National institute for viral disease control and prevention, Chinese centre for disease control and prevention, Beijing, China). And RSV-Luc was kindly provided by Dr. Marie-Anne Rameix-Welti (University of Versailles Saint-Quentin, Versailles, France). RSV-Luc and wtRSV were propagated in HEp-2 cells using DMEM (Gibco BRL, Gaithersburg, MD, USA) with 2% FBS (Hyclone, Logan, UT, USA), L-glutamine (2 mM final concentration), penicillin G (40 U/ml), streptomycin (100 μg/ml) and 0.2% sodium bicarbonate. Then RSV-Luc and wtRSV were purified by sucrose ultracentrifugation and titrated for infectivity by immunoplaque assay, and expressed as plaque-forming units (PFU) per ml.
Drugs and compounds
P13 and Ribavirin were purchased from Topscience Company (Shanghai, China). GS-5806 (fusion inhibitor) and RSV-604 (N protein inhibitor) were purchased from MCE (MedChemExpress, Monmouth Junction, NJ, USA). D-luciferin potassium salt was purchased from Sigma (Sigma, St. Louis, MO, USA). Ribavirin was dissolved in deionised water, and the synthetic compounds (4–49 C and 1-HB-63) were dissolved in DMSO to prepare the working solution.
Time-of-addition assay
The time-of-addition assay was performed as follows according to our previous work and literatureCitation25,Citation27. HEp-2 cells were plated in 96 well plates at 1.5 × 104 cells per well in 100 µL culture medium and incubated for 24 h (37 °C, 5% CO2). Then, HEp-2 cells were infected with wtRSV at a multiplication of infection (MOI) of 5 for 2 h (37 °C, 5% CO2). The cells were washed with PBS and the media containing drugs and compounds of 4–49 C, 1-HB-63, RSV-604, GS-5806 and Ribavirin at 1 mM, 200 μM, 10 μM, 1 mM and 100 μM, respectively, were added at −1, 0, 2, 4, 6, 8, 10, 12 and 14 h post infection. After the treated cells were further cultured and collected at 24 h post infection, the total RNA was isolated from the HEp-2 cells using TRIzol reagent (Thermo Fisher Scientific, Waltham, MA, USA) and reverse transcribed with GoScript Reverse Transcriptase kit (Promega, Madison, WI, USA). Then, RT-qPCR was performed as described previouslyCitation28.
RSV minigenome assay
BHK-T7 cells were seeded into 24 well plates at a density of 2 × 104 cells/well and incubated for 24 h. Then the RSV minigenome plasmid encoding firefly luciferase (pBR322B-RSV-Gluc) was mixed with the helper plasmids (pCITE-N, pCITE-P, pCITE-L and pCITE-M2-1) in an Eppendorf tube containing 250 μL Opti-MEM. Meanwhile, the transfection reagent of Lipofectamine 2000 (Invitrogen, CA, USA) was diluted in another tube containing 250 μL Opti-MEM and incubated 5 min at room temperature. Then, the plasmids were mixed with the transfection reagent and incubated at room temperature for 30 min. After that, the BHK-T7 culture media were replaced with the DNA-lipo-optiMEM mixture and incubated for 4 ∼ 6 h (37 °C, 5% CO2). Then the DNA-lipo-optiMEM mixture was replaced with media containing the compounds (4–49 C at 1 mM and 1-HB-63 at 200 μM) and further incubated for 24 h (37 °C, 5% CO2). Finally, after the supernatant of the BHK-T7 cells was transferred into 96 well plate, the Gluc activity was determined by using LUMI star (BMG ABTECH, Ortenberg, Germany) following the manufacturer’s instructions after adding the chromogenic substrate of Gaussia Luciferase Flex Assay Kit (NEB, Ipswich, USA). The results were recorded by photography and/or evaluated with OPTIMA software (BMG LABTECH). The resultant signal strength was quantified as relative light unit (RLU).
In vivo mouse imaging
Female BALB/c mice of 6-8 weeks were purchased from Charles River Laboratories, Beijing, China and randomly divided into different groups with six mice in each group. All animal experiments were performed in compliance with protocols approved by the Institutional Animal Care and Use Committees of Tsinghua University (No. 17-DZJ1). The compounds (DMSO and ribavirin of 90 mg/kg was used as negative and positive control, respectively) with three different dose groups of 10, 30 and 90 mg/kg were administered to mice by intraperitoneal (i.p.) injection or oral route (anaesthesia with 2% halothane) at zero day and 1 h prior to the mice infected with 50 μL RSV-Luc (1 × 106 PFU), and then once a day for 5 consecutive days post RSV-Luc infection. For the in vivo mouse imaging, 50 μL PBS containing D-Luciferin Potassium Salt (75 mg/kg) was administered to BALB/c mice via nasal drip at days 1, 2, 3, 4 and 5 since RSV-Luc infection, and 5 min later, the whole-body images of BALB/c mice were acquired using an IVIS Lumina II in vivo imaging system (PE, Waltham, MA, USA). And the signal intensity was quantified as the total flux (photons/seconds) using Living Image software (PE).
Determination of RSV titre from lung tissues
Following the experiment of the in vivo mouse imaging mentioned above, the mice were sacrificed. The lung tissues were collected, weighed, quickly frozen under liquid nitrogen and ground with a tissue homogeniser. Then the total RNA was extracted by Trizol from 100 µL homogenates. The viral RNA sample (1 µL) was mixed with the SYBR Green supermix (10 µL), plasmid pMD-18T-N (1 µL, copy number from 1 × 107 copies/μL to 1 × 105 copies/μL), the upstream and downstream primers, respectively (10 µM, 1 µL) and water (1 µL). Reactions were performed in SLAN-96P Real-Time PCR System (Shanghai Hongshi Medical Technology) as follows: 42 °C for 60 min (for reverse transcription), 95 °C for 1.5 min, 95 °C for 15 s and 60 °C for 1 min, for 40 cycles. The reaction data were analysed by determining the threshold cycle (Ct).
Pharmacokinetic study in rat
Six male Sprague − Dawley (SD) rats, randomly divided into two groups of 3 each, were treated with 4–49 C predissolved in 5% DMSO, 5% Solutol and 90% Saline by intravenous injection (iv, 5 mg/kg) and in 5% DMSO and 95% (0.5%MC) by intragastric administration (ig, 10 mg/kg), respectively. Blood samples (100 μL) were collected into K2-EDTA tubes by retro‐orbital bleeding under anaesthesia using isoflurane at 0.033, 0.083, 0.25, 0.5, 1, 2, 4, 7 and 24 h after dosing. And then plasma samples were obtained by centrifuging at 5000 rpm for 10 min at 4 °C and stored at −80 °C until analysis using HPLC–MS/MS.
Data processing and statistics
Graphpad prism 7 software was used for statistical analysis. The t-test was used when there was one comparison, while one-way ANOVA was performed for multiple comparisons. P < 0.05 was considered statistically significant.
Results and discussion
The time-of-addition assay, hence, was carried out to specify replication stage (s) at which 4–49 C and 1-HB-63 () inhibit RSV infection, respectively. As the results shown in , compared with DMSO (negative control), ribavirin (genome replication stage-targeted inhibitor), RSV604 (N protein inhibitor), and GS-5806 (fusion inhibitor), 4-49 C significantly inhibited virus replication when added at the time points of −1, 0 and 2 h, similarly to the GS-5806 having inhibition activity only when added up to 0 and 2 h. While 1-HB-63 significantly inhibited virus replication when added at the time points of −1, 0, 2 and 4 h. Thus, the results indicated that 4-49 C inhibits virus replication in the initial stage of RSV infection or before entry of the targeted cells, and 1-HB-63 acts in the late stage of RSV infection or after entering the cells.
Figure 2. Investigation on the underlying mechanism 4–49 C and 1-HB-63 inhibiting wtRSV replication. (A) Time-of-addition analyses of 4-49C and 1-HB-63 replication. The HEp-2 cells were infected with wtRSV at a multiplication of infection (MOI) of 5 for 2 h (37 °C, 5% CO2). The cells were washed with PBS and the media containing drug (4-49C, 1-HB-63, RSV-604, GS-5806 and Ribavirin at 1 mM, 200 μM, 10 μM, 1 mM and 100 μM, respectively.) were added at −1, 0, 2, 4, 6, 8, 10, 12 and 14 h separately and incubated till 24 h post infection. Then, the cells were harvested and lysed for RNA extraction and RT-qPCR assay. The results were a representative of three independent experiments, and shown as means ± standard and expressed as % negative control, although in many cases the bars for the standard deviation were obscured by the symbols given the small margin of values. (B) RSV adsorption analyses. After HEp-2 cells seeded into 24 well plates for 24 h, the cold media containing RSV (5 MOI) and compounds of 4–49C, 1-HB-63 and P13 at 1 mM, 200 μM and 100 μM, respectively, was added and incubated for 1 h at 4 °C. Then, the total RNA was isolated and RT-qPCR was performed. (C) RSV minigenome assay. After 24 h, we cotransfected RSV minigenome plasmid of pBR322B-RSV-Gluc with four auxiliary plasmids into BHK/T7 cells and detected the expression of Gluc in the supernatant. The results were shown as means ± standard deviations for three independent experiments, *p < 0.05, ***p < 0.001.

Further, the experiment on whether the chemicals inhibited RSV replication by affecting the attachment of RSV to cells was performed (). We found that neither of 4-49 C and 1-HB-63 inhibited RSV replication by preventing virus adsorption. Then, RSV minigenome assay by co-transfecting RSV minigenome plasmid of pBR322B-RSV-Gluc and four auxiliary plasmids into BHK/T7 cells was also carried out. After 24 h, the expressed Gluc in the supernatant of BHK/T7 cells was detected and shown in . Compared with the negative control of DMSO and the positive controls of GS-5806 and ribavirin, the expression of Gluc gene could not be inhibited by 4–49 C, similar to GS-5086, which is sharply contrast to the effect of 1-HB-63 or ribavirin, thus suggesting that 4–49 C and 1-HB-63 may play anti-RSV roles by inhibiting membrane fusion and by inhibiting RSV replication at the stage of RSV genome replication/transcription, respectively. Therefore, the study described above is invaluable to clarify the underlying mechanism of these two anti-RSV compounds, 4–49 C and 1-HB-63, and lays an important foundation for further investigation of their in vivo antiviral activities.
Although BALB/c mouse is semi-permissive to RSV infection, the weight loss and the lung pathological changes as well as the virus replication in lung tissue are observable following the infection intranasally. In addition to the traditional immuno-plaque or RT-qPCR assays, in vivo non-invasively mouse imaging can be used to investigate the pharmacokinetics and pharmacodynamics of anti-RSV drugs. Thus, in this paper, RSV-Luc, the recombinant RSV expressing the luciferase reporter gene, was selected to infect BALB/c mice and combined with an in vivo imaging technology to monitor the in vivo pharmacodynamics of 4–49 C and 1-HB-63.
Female BALB/c mice of 6–8 weeks were randomly divided into different groups with 6 mice in each group. All animal experiments were performed in compliance with protocols approved by the Institutional Animal Care and Use Committees of Tsinghua University (No. 17-DZJ1). At day 0, three different doses of 4–49 C and 1-HB-63 (10 mg/kg, 30 mg/kg, 90 mg/kg) were administered to mice by intraperitoneal (i.p.) injection, respectively, with DMSO and ribavirin of 90 mg/kg separately used as negative and positive control. One-hour later, the mice were infected with 50 µL RSV-Luc (1 × 106 PFU). Then two different compounds were administered once a day for 5 consecutive days and the replication levels of RSV-Luc in the lungs of BALB/c mice were observed using an IVIS Lumina II in vivo imaging system. As shown in and , except for the groups administered 4–49 C of 10 mg/kg and 90 mg/kg on the second day, the fluorescence signals from the groups both 4–49 C and 1-HB-63 did not display significant differences at any doses and any time points compared with DMSO-treated group, and the results of statistical analysis shown in and were also proved this. Furthermore, the virus titres in lung tissues at day 5 post-infection were also detected by RT-qPCR and no significant differences were found either between the groups of treatments and DMSO ( and ). Thus, the results indicated that 4–49 C and 1-HB-63 injected intraperitoneally had no inhibitory effect on RSV replication in the infected mice.
Figure 3. The in vivo anti-RSV activity of 4–49 C administered by intraperitoneal injection. A, in vivo imaging of BALB/c mice following infection of RSV-Luc and treatment with 4-49C pre- and post-infection; B, the replication levels of RSV-Luc, represented by luciferase activity, in each group of mice following infection and treatments at different dosages of 10, 30 and 90 mg/kg and at different time points; C, viral titres in the lung tissues from each group of mice analysed by RT-qPCR. The results were shown as means ± standard deviations, *p < 0.05, **p < 0.01.

Figure 4. The in vivo anti-RSV activity of 1-HB-63 administered by intraperitoneal injection. A, in vivo imaging of BALB/c mice following infection of RSV-Luc and treatment with 1-HB-63 pre- and post-infection; B, the replication levels of RSV-Luc, represented by luciferase activity, in each group of mice following infection and treatments at different dosages of 10, 30 and 90 mg/kg and at different time points; C, viral titres in the lung from each group of mice analysed by RT-qPCR. The results were shown as means ± standard deviations, *p < 0.05, **p < 0.01.

Figure 5. The in vivo anti-RSV activity of 4-49 C and 1-HB-63 administered by oral route. A, in vivo imaging of BALB/c mice following infection of RSV-Luc and treatment with 4-49 C and 1-HB-63 pre- and post-infection, respectively; the replication levels of RSV-Luc, represented by luciferase activity, in each group of mice following infection and treatments with 4-49C (B) or 1-HB-63 (C) at different dosages of 30 and 90 mg/kg and at different time points. The results were shown as means ± standard deviations, *p < 0.05, **p < 0.01.

In order to further make clear whether the anti-RSV activity of 4–49 C and 1-HB-63 is dependent on the administration route or not, these two compounds were given intragastrically (). Except for the doses of 30 mg/kg, 90 mg/kg and gavage twice a day, other procedures were the same as those in intraperitoneal injection experiment. As shown in , compared with DMSO negative control group, the fluorescence signals from the groups of 4–49 C and 1-HB-63 did not exhibit significant differences, which was consistent with the above results by using intraperitoneal injection. Therefore, 4–49 C and 1-HB-63 could not inhibit RSV replication in vivo following intragastric application.
To investigate the pharmacokinetic parameters in vivo, 4–49 C was selected for the pharmacokinetic experiments in SD rat model. The SD rats were divided into two groups (n = 3) and treated by iv at 5 mg/kg dose and by ig at 10 mg/kg dose, respectively. Plasma samples were collected up to 24 h postdosing and the concentration of 4–49 C was measured by using HPLC-MS/MS. As shown in , the T1/2 was 0.167 ± 0.3 h and AUC0-t was 69.3 ± 17 h⋅ng/mL after iv administration implying that 4–49 C was rapidly metabolised in plasma. Unfortunately, there is no 4–49 C detected in plasma samples after ig administration (data not shown). The PK results indicate that the poor in vivo efficacy of 4–49 C may due to their overly fast metabolism.
Table 1. Pharmacokinetic parameters of 4–49 C (iv, 5 mg/kg)
In summary, 2-((1H-indol-3-yl) thio/sulfinyl)-N-pheny acetamide derivatives as a novel inhibitor against RSV replication were screened successfully, and the underlying antiviral mechanism was elucidated in vitro. However, no in vivo inhibitive activity on RSV infection was observed based on BALB/c infection model. And the PK results showed that there was a fast metabolism in vivo after dosing. Our work proves the difficulty and complexity of drug discovery and development and suggests that further structural modification of 2-((1H-indol-3-yl) thio/sulfinyl)-N-pheny acetamide derivative is needed to improve their stability in vivo, and intranasal administration strategy should also be explored.
Disclosure statement
No potential conflict of interest was reported by the author(s).
Additional information
Funding
References
- Collins PL, Fearns R, Graham BS. Respiratory syncytial virus: virology, reverse genetics, and pathogenesis of disease. Curr Top Microbiol Immunol 2013;372:3–38.
- Branche AR, Falsey AR. Respiratory syncytial virus infection in older adults: an under-recognized problem. Drugs Aging 2015;32:261–269.
- Amarasinghe GK, Arechiga Ceballos NG, Banyard AC, et al. Taxonomy of the order Mononegavirales: update 2018. Arch Virol 2018;163:2283–2294.
- Nam HH, Ison MG. Respiratory syncytial virus infection in adults. Bmj 2019;366:l5021.
- Noton SL, Nagendra K, Dunn EF, et al. Respiratory syncytial virus inhibitor AZ-27 differentially inhibits different polymerase activities at the promoter. J Virol 2015;89:7786–7798.
- Battles MB, McLellan JS. Respiratory syncytial virus entry and how to block it. Nat Rev Microbiol 2019;17:233–245.
- Cartee TL, Megaw AG, Oomens AG, et al. Identification of a single amino acid change in the human respiratory syncytial virus L protein that affects transcriptional termination. J Virol 2003;77:7352–7360.
- Krempl C, Murphy BR, Collins PL. Recombinant respiratory syncytial virus with the G and F genes shifted to the promoter-proximal positions. J Virol 2002;76:11931–11942.
- Barr R, Green CA, Sande CJ, et al. Respiratory syncytial virus: diagnosis, prevention and management. Ther Adv Infect Dis 2019;6:2049936119865798.
- Modjarrad K, Giersing B, Kaslow DC, WHO RSV Vaccine Consultation Expert Group, et al. WHO consultation on respiratory syncytial virus vaccine development report from a World Health Organization meeting held on 23-24 March 2015. Vaccine 2016;34:190–197.
- Glezen WP, Taber LH, Frank AL, et al. Risk of primary infection and reinfection with respiratory syncytial virus. Am J Dis Child 1986;140:543–5466.
- Shi T, McAllister DA, O'Brien KL, RSV Global Epidemiology Network, et al. Global, regional, and national disease burden estimates of acute lower respiratory infections due to respiratory syncytial virus in young children in 2015: a systematic review and modelling study. Lancet 2017;390:946–958.
- Falsey AR, Walsh EE. Respiratory syncytial virus infection in adults. Clin Microbiol Rev 2000;13:371–384.
- Falsey AR. Respiratory syncytial virus infection in adults. Semin Respir Crit Care Med 2007;28:171–181.
- Gavin PJ, Katz BZ. Intravenous ribavirin treatment for severe adenovirus disease in immunocompromised children. Pediatrics 2002;110:e9.
- Perron M, Stray K, Kinkade A, et al. GS-5806 inhibits a broad range of respiratory syncytial virus clinical isolates by blocking the virus-cell fusion process. Antimicrob Agents Chemother 2015;60:1264–1273.
- Xiong H, Foulk M, Aschenbrenner L, et al. Discovery of a potent respiratory syncytial virus RNA polymerase inhibitor. Bioorg Med Chem Lett 2013;23:6789–6793.
- Mitchell I, Joffe A, Newth CJ. Ribavirin. Red Book Committee recommendations questioned. Pediatrics 1995;95:319–320.
- Hu J, Robinson JL. Treatment of respiratory syncytial virus with palivizumab: a systematic review. World J Pediatr 2010;6:296–300.
- Fenton C, Scott LJ, Plosker GL. Palivizumab: a review of its use as prophylaxis for serious respiratory syncytial virus infection. Paediatr Drugs 2004;6:177–197.
- Helmink BJ, Ragsdale CE, Peterson EJ, et al. Comparison of intravenous palivizumab and standard of care for treatment of respiratory syncytial virus infection in mechanically ventilated pediatric patients. J Pediatr Pharmacol Ther 2016;21:146–154.
- Wat D. Impact of respiratory viral infections on cystic fibrosis. Postgrad Med J 2003;79:201–203.
- Empey KM, Peebles RS, Jr., Kolls JK. Pharmacologic advances in the treatment and prevention of respiratory syncytial virus. Clin Infect Dis 2010;50:1258–1267.
- Olszewska W, Openshaw P. Emerging drugs for respiratory syncytial virus infection. Expert Opin Emerg Drugs 2009;14:207–217.
- Fu YH, Xu ZX, Jiang N, et al. High-throughput screening of active compounds against human respiratory syncytial virus. Virology 2019;535:171–178.
- Zhang GN, Li Q, Zhao J, et al. Design and synthesis of 2-((1H-indol-3-yl)thio)-N-phenyl-acetamides as novel dual inhibitors of respiratory syncytial virus and influenza virus A. Eur J Med Chem 2020;186:111861.
- Rameix-Welti MA, Le Goffic R, Herve PL, et al. Visualizing the replication of respiratory syncytial virus in cells and in living mice. Nat Commun 2014;5:5104.
- Jiao YY, Fu YH, Yan YF, et al. A single intranasal administration of virus-like particle vaccine induces an efficient protection for mice against human respiratory syncytial virus. Antiviral Res 2017;144:57–69.