Abstract
Based on the multitarget-directed ligands (MTDLs) strategy, a series of chromone-hydroxypyridinone hybrids were designed, synthesised, and evaluated as potential multimodal anti-AD ligands. Prospective iron-chelating effects and favourable monoamine oxidase B (MAO-B) inhibitory activities were observed for most of the compounds. Pharmacological assays led to the identification of compound 17d, which exhibited favourable iron-chelating potential (pFe3+ = 18.52) and selective hMAO-B inhibitory activity (IC50 = 67.02 ± 4.3 nM, SI = 11). Docking simulation showed that 17d occupied both the substrate and the entrance cavity of MAO-B, and established several key interactions with the pocket residues. Moreover, 17d was determined to cross the blood–brain barrier (BBB), and can significantly ameliorate scopolamine-induced cognitive impairment in AD mice. Despite its undesired pharmacokinetic property, 17d remains a promising multifaceted agent that is worth further investigation.
Introduction
Disease‐modifying therapy for Alzheimer’s disease (AD) is far from satisfactoryCitation1. The underlying pathogenesis of AD remained frustratingly under debate despite significant efforts over the last 50 yearsCitation2,Citation3. Interrelated and multifaced etiopathology among AD pathological factors was proposed, especially for iron dyshomeostasis and abnormal MAO-B activitiesCitation4–6. Elevated iron has been proved to deposit within the vulnerable neuronal populations and potentiates oxidative stress via the Fenton– and Haber–Weiss reactions, as well as by increasing lipid peroxidative stressCitation7–9. Increased neuronal iron in AD is known to enhance Aβ production and oligomerisation, further facilitating tau dysfunction and neurofibrillary tanglesCitation10,Citation11. As such, human monoamine oxidases (MAOs) are flavin adenine dinucleotide (FAD)-containing enzymes responsible for the oxidative deamination of monoamine neurotransmitters, and the elevated MAO-B activity led to a higher level of neurotoxic byproducts accelerates neurotransmitters consumption, and neuronal damageCitation12,Citation13.
Recently, following the “one molecule, multiple target” paradigm, the emerged multitarget-directed ligands (MTDLs) strategy has been suggested as a powerful and promising alternative paradigm for developing effective anti-AD agentsCitation14,Citation15. MAO-B has been paid increasing attention as the multifunctional anti-AD agent design target as a consequence of the neuroprotective and neurorescue effects of MAO-B inhibitorsCitation16. Currently, dozens of MAO-B inhibitors with auxiliary beneficial properties or target affinity (e.g. antioxidative ability, inhibit Aβ aggregation, AChE inhibition, and metal chelation) have been developed by integrating pharmacophores or scaffolds from two or more molecules and were proved to undergo unique anti-AD mechanisms ()Citation17–21.
Several pieces of evidence reported by our research group have revealed that coumarin/benzamide-based multi-target ligands with the iron-chelating ability and MAO-B inhibitory activity have an attractive anti-AD potentialCitation20,Citation22. Aiming at identifying new multipotent ligands, in this work, a new series of chromone-hydroxypyridinone hybrids were rationally designed by linking chromone core and hydroxypyridinone moiety with the appropriate linkers (). Since studies have demonstrated that 3-carboxyl chromone is a privileged scaffold of MAO-B and possesses specific selectivity over MAO-A isoform, accordingly, the hydroxypyridinone fragment was assembled to 3-position of chromone coreCitation23–25. From the induce-fit perspective, the flexible ethylenediamine linker was chosen to accommodate the hydrophobic cavity as well as partially diminish the rigidity of the moleculeCitation12,Citation26. Modifications on the 7-position of the chromone ring were also investigated since studies prove that benzyloxy decoration can promote selectivity and efficacyCitation27,Citation28. Thus, we synthesised and evaluated chromone-hydroxypyridinone hybrids to explore their bioactivity in association with AD.
Results and discussion
Chemistry
According to the difference in linkers, two series of chromone derivatives were outlined: (I) amide-bonded compounds, and (II) C–-N bonded compounds. The amide-bonded chromone derivatives (8a–e, 15a–g) were efficiently obtained according to the procedure shown in Schemes 1–3. Intermediate 2 was synthesised starting from commercially available maltol through the protection of the 3-hydroxyl group. Subsequently, the protected maltol was converted into intermediate 3 by reaction with ethylenediamine under reflux conditions (Scheme 1).
Scheme 1. Reagents and conditions: (i) 4-Methoxybenzyl chloride, K2CO3, acetone, reflux, 3–8 h. (ii) Ethane-1,2-diamine, Ethanol: H2O = 1:1, 70 °C, 1.5 h.
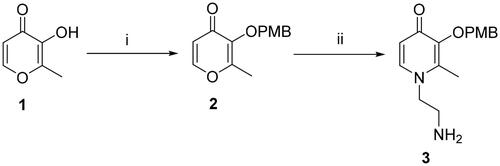
Appropriate chromone-carbaldehydes (5a–e) were synthesised by corresponding acetophenone through the Vilsmeier–Haack reactionCitation23. Afterward, the chromone carboxylic acids (6a-e) were prepared by Pinnick oxidation, in the presence of sodium chloriteCitation29. The chromone-carboxamide compounds (7a–e) were obtained through a reaction that included the generation of an acyl chloride intermediate and the subsequent addition of intermediate 3 (Scheme 2).
Scheme 2. Reagents and conditions: (i) POCl3, DMF, −10 °C, 15 h. (ii) NH2SO3H, NaClO2, 0 °C, 12 h. (iii) POCl3, DMF, 3, r.t., 10 h. (iv) BCl3, anhydrous DCM, −48 °C to r.t., 12 h.
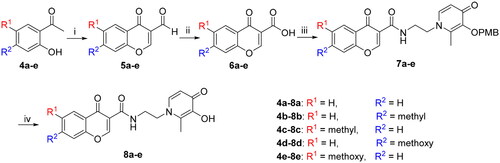
The synthetic pathway followed to obtain the first series of compounds (15a–g) involved seven steps (Scheme 3). Except for the difference in acyl chloride reagents, the synthetic procedure of compound 13 was the same as that of the chromone-carboxamide derivatives (7a–e). Subsequently, the ester group of compound 13 was hydrolysed with potassium carbonate and treated with appropriate benzyl bromide to afford the compounds (14a–g).
Scheme 3. Reagents and conditions: (i) POCl3, DMF, −10 °C 15 h. (ii) Acetyl chloride, Triethylamine, anhydrous DCM, 0 °C to r.t., 3 h. (iii) NH2SO3H, NaClO2, 0 °C, 12 h. (iv) Oxalyl chloride, DMF, anhydrous DCM, 3, r.t., 3 h. (v) K2CO3, acetonitrile: H2O = 1:1, reflux, 0.5 h. (vi) corresponding substituted benzyl bromide, K2CO3, DMF, 12 h (vi) BCl3, anhydrous DCM, −48 °C to r.t., 12 h.
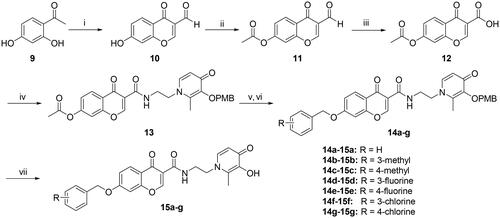
In turn, the second series of compounds (16a–l) were synthesised by reductive amination, starting from chromone-carbaldehydes (5a–l) to yield the imine intermediate (Scheme 4). Finally, the chromone-based compounds (8a–e, 15a–g, and 16a–l) were performed by a selective debenzylation reaction with boron trichloride to remove the 4-methoxybenzyl protecting groups.
Iron-chelating ability test
Recently, iron has been determined as a pro-oxidant involved in oxidative damage due to Fenton– and Haber–Weiss reactions and ferroptosisCitation30. To evaluate the iron chelating capacity of chromone derivatives, all of the compounds were then subjected to determine the pKa values and iron affinity constants for iron (III) by an automated spectrophotometric titration systemCitation20. The obtained data are provided in . All derivatives displayed impressive pFe3+ values ranging from 15.87 to 19.08 (). Compound 8e revealed the most potent iron-chelating ability (pFe3+ = 19.08). Overall, no discernible difference in iron-chelating activity exists between the two series of compounds (compounds 8a–e, 15a–g, and 17a–l), both of which are outstanding.
Table 1. Physicochemical parameters for compounds 8a–e, 15a–g, and 17a–l.
Typically, 3-hydroxypyridin4-one derivatives are bidentate ligands (3:1 complex) and their proton ionisation characteristics, the UV spectrums yielded two pKa values (pKa1 < 3.40, pKa2 = 9.32 − 10.05). The 2D UV titration profile of 17d over the pH range 1.3–11.0 () yielded two pKa Values, 2.86 and 9.78 demonstrating the pH dependence of the ligand ionisation equilibrium (). Repeated the titration in the presence of iron (III), over the pH range of 2.1 − 9.0, the visible spectrum reveals three species, FeL, FeL2, and FeL3, respectively, in the solution system. The spectral resolution of these three substances led to the determination of the corresponding three equilibrium constants (compound 17d): Log β1 (13.50), Log β2 (25.21) (), and Log β3 (35.13) (), which were the basis for calculating the final pFe3+ value. The abundance of each species varied with pH as illustrated in .
Figure 4. The pH-dependent UV spectra of compound 17d. (A) The pH-dependence of the spectrum of compound 17d in the presence of Fe3+ over the pH range 0.8–2.1 in 0.1 M KCl at 25 °C, [Fe3+] = 1.0 μM, [17d] = 1.1 μM. (B) The pH-dependence of the spectrum of compound 17d in the presence of Fe3+ over the pH range 2.1 and 9.0 in 0.1 M KCl: DMSO = 3:2 (v/v) at 25 °C, [Fe3+] = 1.0 μM, [17d] = 5.0 μM. C. Speciation plot of Fe3+/17d as measured by the percentage formation relative to [Fe3+]total as a function of pH. This plot was calculated from the affinity constants reported in and the Fe3+ hydrolysis constants were as follows: FeOH = –2.563, Fe (OH)2 = –6.205, Fe (OH)3 = –15.100, Fe2 (OH)2 = –2.843, Fe3 (OH)4 = –6.059, and Fe (OH)4 = –21.883.
![Figure 4. The pH-dependent UV spectra of compound 17d. (A) The pH-dependence of the spectrum of compound 17d in the presence of Fe3+ over the pH range 0.8–2.1 in 0.1 M KCl at 25 °C, [Fe3+] = 1.0 μM, [17d] = 1.1 μM. (B) The pH-dependence of the spectrum of compound 17d in the presence of Fe3+ over the pH range 2.1 and 9.0 in 0.1 M KCl: DMSO = 3:2 (v/v) at 25 °C, [Fe3+] = 1.0 μM, [17d] = 5.0 μM. C. Speciation plot of Fe3+/17d as measured by the percentage formation relative to [Fe3+]total as a function of pH. This plot was calculated from the affinity constants reported in Table 1 and the Fe3+ hydrolysis constants were as follows: FeOH = –2.563, Fe (OH)2 = –6.205, Fe (OH)3 = –15.100, Fe2 (OH)2 = –2.843, Fe3 (OH)4 = –6.059, and Fe (OH)4 = –21.883.](/cms/asset/43bf30e7-dbaf-4ef4-b690-26d10e86f00c/ienz_a_2134358_f0004_c.jpg)
Figure 5. Kinetic study and cytotoxicity assay of compound 17d. Mode of MAO-B inhibition saturation curves (A) and Lineweaver − Burk plot (B) of the inhibition of MAO-B enzyme by different concentrations of 17d (0, 33.3, 100, and 300 nM) in the presence of p-tyramine (0.05, 0.1, 0.25, 0.5, 1.0, and 1.25 mM) as a substrate. The Cytotoxicity effect of compound 17d on PC-12 cells (C).
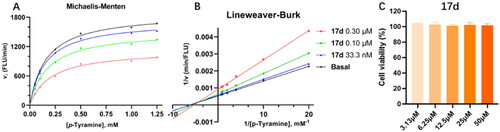
As shown in , all of the compounds well inherited the iron-chelating ability of the hydroxypyridinone pharmacophore. Compounds with 6,7-alkyl and 6,7-alkoxy substitutions on the benzene ring showed better iron chelation than benzyloxy substitutions. Meanwhile, amide bonds linked compounds exhibited better iron chelation than C–N bond-linked derivatives.
HMAO-B/a inhibition assay and selectivity
Subsequently, the MAOs inhibitory activity of the compounds was measured, as shown in and . As detailed below, generally, hybrids with C–N bond linkers were more potent than those amide bond-linked compounds, with most of them having inhibition rates over 40%. Chromones bearing substituents in position C-7 of chromone exhibited better MAO-B inhibitory activity with IC50 values ranging from micromolar concentration to nanomolar concentration. Furthermore, when the benzyloxy group was inserted into the C-7 of chromone, the inclusion of electron-withdrawing substituents (F, Cl) in the benzyloxy counterposition demonstrated higher activity than interposition replacement. The most promising chromone derivatives, on the other hand, are changed by alkyl groups at C-6 or C-7 of chromone nuclear (compounds 17a–e and IC50 values range from 67 to 88 nM). Among them, compound 17d showed the best inhibitory activity compared with the reference drug (Pargyline).
Table 2. The hMAO-B inhibitory activities of compounds.
Table 3. IC50 and SI for the chromone derivatives on the enzyme activity of MAO isoforms.
Further, the inhibitory activity of compounds against hMAO-A isoform was tested and calculated as a selectivity index [Selectivity index (SI): IC50 (hMAO-A)/IC50 (hMAO-B)]. As indicated in , all compounds demonstrated varying degrees of selectivity, with compound 17b exhibiting high selectivity for MAO-B. Comprehensively considering the iron-chelating ability and the inhibitory activity over enzyme isoforms, compounds 17a and 17d could be performed in subsequent assays.
PAMPA-BBB assay
Good blood–brain barrier (BBB) permeability is the key to exerting drug efficacy in the treatment of central nervous system diseases. To evaluate whether the target compound could penetrate BBB, a parallel artificial membrane permeability assay (PAMPA) was used to predictCitation32,Citation33. As outlined in , The Pe value of compound 17a measured by this method was 1.22 ± 0.22 × 1 0−6 cm/s (CNS-), indicating that compound 17a had poor permeability and could not penetrate the BBB. However, the Pe value of compound 17d was between 2 and 4, suggesting that compound 17d had the possibility of penetrating the BBB. To further confirm these results, a prediction platform (ADMETlab) was selected for verification. Both compounds displayed the desired CNS drug-like properties (MW ≤ 450, HBD ≤ 5, HBA ≤ 10, and Log p ≤ 5) and were predicted to pass through the BBB, which is classified as BBB+. Therefore, due to its potential ability to cross the BBB, compound 17d was selected for subsequent assay.
Table 4. Predicted drug-like properties and PAMPA-BBB results for 17a and 17d.
Kinetics study and cytotoxicity assay of compound 17d
To examine the interaction mode of 17d on MAO-B, kinetic studies were carried out. From the Lineweaver–Burk plots (), we observed that 17d operated by a non-competitive inhibition mechanism. Using GraphPad Prism, the Michaelis constant (Km), the maximal velocity (Vmax) and the inhibition constant (Ki) were calculated (Km = 117.7 μM, Vmax = 1701, and Ki = 74.71 nM). Subsequently, the potential toxic effect of 17d was investigated on pheochromocytoma (PC-12) cells. Compound 17d exhibited no cytotoxicity even at the concentration of 50 µM ().
Molecular modelling
The potential binding conformation of compound 17d in MAO-B (PDB: 2V5Z) was investigated by molecular docking simulation in Discovery studio version 4.0 (BIOVIA, USA). As shown in , 17d occupied both the entrance and substrate cavities of MAO-B with the 7-methoxy-chromone ring oriented towards the highly hydrophobic entrance cavity and formed Pi–Pi interaction with TYR 326. Moreover, the chromone-carbonyl oxygen established two hydrogen bonds with residues TYR 326 (3.3 Å) and GLN 206 (3.0 Å). Accordingly, The pyridinone moiety of 17d occupied the substrate cavity in front of the FAD cofactor. Pi–Pi interaction was observed between the pyridinone plane and TYR 435, and a hydrogen bond interaction was monitored between pyridinone-carbonyl oxygen and the residue TYR 188. The above binding mode indicated significant interactions between pyridinone moiety and surrounding amino acid residues, indicating that pyridinone moiety, not only provided chelating ability but also functioned as a critical piece in binding with MAO-B.
Cognitive and memory assays in vivo
Towards this end, compound 17d exhibited favourable multipotent activity. To further explore the in vivo anti-AD effect of compound 17d on the scopolamine-induced cognitive impairment mice model, the Morris Water Maze experiment was selected; data are shown in and .
Figure 7. Pargyline (15 mg/kg), Memantine (15 mg/kg), and compound 17d (15 mg/kg) were evaluated for scopolamine-induced (15 mg/kg) memory impairment in ICR mice in the Morris water maze. The mouse trajectories of the mice are shown as the control (D–1), model (D–2), pargyline (D–3), Memantine (D–4), and 17d (D–5) groups. Data are presented as the mean ± SEM (n = 15; #p < 0.05, ##p < 0.01, ###p < 0.001, and ####p < 0.0001, Control group vs. scopolamine model group; *p < 0.05, **p < 0.01, ***p < 0.001, and ****p < 0.0001, Pargyline group, Memantine group, 17d vs. scopolamine model group).
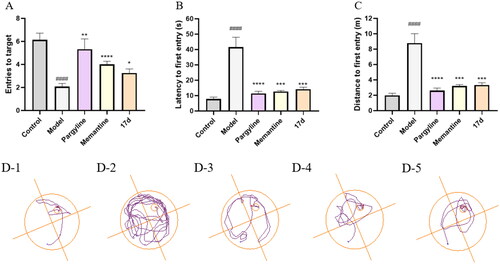
Table 5. Effects of compound 17d (15 mg/kg) on scopolamine-induced memory impairment in ICR mice evaluated by the Morris water maze test.
According to the latency () and distance () to the first entry, the time (42 ± 6 vs. 8 ± 1, ####p < 0.0001) and distance (8.9 ± 1.2 vs. 2.0 ± 1.3, ####p < 0.0001) of reaching the platform of mice treated with scopolamine were significantly prolonged, indicating that the mice model of cognitive impairment has been well-established. According to the entries to target (), treatment with pargyline and memantine notably increased the entries they crossed the platform (5.3 ± 0.9 vs. 2.1 ± 0.3, #p < 0.05; 4.0 ± 0.3 vs. 2.1 ± 0.3, ####p < 0.0001). As shown in , treatment with pargyline markedly improved the cognitive impairment and reduced the latency to reach the platform compared with the model group (11 ± 1 vs. 42 ± 6, ****p < 0.0001), so as memantine (13 ± 1 vs. 42 ± 6, ***p < 0.001). Furthermore, the latency to target was significantly shortened when treated with 17d (14 ± 1 vs. 42 ± 6, ***p < 0.001). In terms of the distance to the first entry (), mice of the pargyline group led to a remarkably shorter distance to the first entry (2.6 ± 0.3 vs. 8.9 ± 1.2, ****p < 0.0001). The group of 17d exhibited a comparable activity to pargyline (3.3 ± 0.3 vs. 2.6 ± 0.3).
According to the trajectory diagram (), the trajectory of pargyline, memantine, and compound 17d were very clear. In contrast, the trajectory of the model group was very chaotic, which demonstrated that the scopolamine-induced cognitive impairment was significantly ameliorated by compound 17d. As illustrated in , except for the control group, all groups of mice showed a significant decrease in body weight after administration on the first day. However, the body weight of mice in group 17d increased after ten days, partially indicating that 17d had no obvious cytotoxicity.
Pharmacokinetic property and BBB permeability investigation
UHPLC-MS/MS method was adopted to investigate the pharmacokinetic profiles of its metabolites after intravenous (i.v.) administration (2.34 mg/kg) and intragastric (i.g.) administration (15 mg/kg) of 17d in the tail vein of rats. The main plasma pharmacokinetic parameters are listed in . As we can see, compound 17d has higher bioavailability in the case of gavage administration (F = 9.25%), and can be absorbed quickly (Tmax = 0.25 h) after i.g. administration. Although shorter half-time and lower brain districts, the above results indicated that 17d would be instructive for further study.
Table 6. Pharmacokinetic parameters of 17d after its administrationa.
Conclusion
Herein, a novel series of chromone-pyridinone hybrids as dual-target-directed agents were designed and synthesised by pharmacophore fusion strategy. The designed derivatives displayed preferable biometal chelating effects and anti-MAO-B activities. Compound 17d was proved to be the most potent MAO-B inhibitor (hMAO-B IC50 = 67.02 ± 4.3 nM), outperforming the reference drug pargyline (hMAO-B IC50 = 111.3 ± 0.6 nM). The molecular docking research revealed that 17d may bind to both the substrate cavity and the entrance cavity of MAO-B. Furthermore, compound 17d was demonstrated as a noncytotoxic agent and was able to considerably ameliorate cognitive dysfunction in a scopolamine-induced mice cognition-impaired model. Despite its undesired PK property, 17d remains a promising multifaceted agent that is worth further investigation.
Experimental section
General information
Unless otherwise indicated, all reagents and solvents were used without further purification acquired from commercial suppliers. NMR spectra were acquired on Bruker and Varian spectrometers at 600, 400 MHz for 1H and 150, 100 MHz for 13C, respectively. Melting points (m.p., uncorrected) were measured with a Büchi B-540 m.p. apparatus. High-resolution mass spectra (HRMS) were recorded with a Shimadzu LCMS-IT-TOF mass spectrometer equipped with an electrospray ionisation (ESI) source. Routinely, the procedure of reactions was monitored on silica gel by thin-layer chromatography. The purity of the final compounds (>97%) was verified by high-performance liquid chromatography (HPLC) equipped with a UV-diode array detector.
General procedure for the preparation of intermediate 4f–l
Anhydrous potassium carbonate (7.5 mmol) and compound 9 (5 mmol) were dissolved in acetone (20 ml), and appropriately substituted benzyl bromide derivatives (5.5 mmol) were added to the mixture and refluxed for 8 h. Upon completion, the solution of reaction was filtered and the filtrate was removed under reduced pressure. The target compounds were purified by recrystallisation with MeOH/DCM to yield solid powder 4f–l.
1–(4-(Benzyloxy)-2-hydroxyphenyl)ethan-1-one (4f)
Yield: 87%, white solid, m.p. = 105–107 °C; 1H NMR (400 MHz, CDCl3) δ 12.73 (s, 1H), 7.64 (d, J = 8.4 Hz, 1H), 7.46–7.34 (m, 5H), 6.53–6.50 (m, 2H), 5.10 (s, 2H), 2.56 (s, 3H); 13C NMR (100 MHz, CDCl3) δ 202.7, 165.3, 136.0, 132.5, 128.9, 128.5, 127.7, 114.2, 108.3, 102.0, 70.4, 26.4.
1–(2-Hydroxy-4-((3-methylbenzyl)oxy)phenyl)ethan-1-one (4g)
Yield: 52%, white solid, m.p. = 98–99 °C; 1H NMR (400 MHz, CDCl3) δ 12.74 (s, 1H), 7.64 (d, J = 8.4 Hz, 1H), 7.29 (t, J = 7.6 Hz, 1H), 7.23 (d, J = 4.8 Hz, 2H), 7.16 (d, J = 7.6 Hz, 1H), 6.53–6.05 (m, 2H), 5.05 (s, 2H), 2.56 (s, 3H), 2.38 (s, 3H); 13C NMR (100 MHz, CDCl3) δ 202.7, 165.4, 165.3, 138.6, 135.9, 132.5, 129.2, 128.8, 128.4, 124.8, 114.2, 108.3, 102.0, 70.4, 26.3, 21.5.
1–(2-Hydroxy-4-((4-methylbenzyl)oxy)phenyl)ethan-1-one (4h)
Yield: 83%, white solid, m.p. = 99–101 °C; 1H NMR (400 MHz, CDCl3) δ 12.73 (s, 1H), 7.63 (d, J = 9.6 Hz, 1H), 7.31 (d, J = 7.6 Hz, 2H), 7.20 (d, J = 7.6 Hz, 2H), 6.52–6.49 (m, 2H), 5.05 (s, 2H), 2.55 (s, 3H), 2.37 (s, 3H); 13C NMR (100 MHz, CDCl3) δ 202.7, 165.4, 165.3, 138.3, 133.0, 132.4, 129.5, 127.8, 114.2, 108.3, 102.0, 70.3, 26.3, 21.3.
1–(4-((3-Fluorobenzyl)oxy)-2-hydroxyphenyl)ethan-1-one (4i)
Yield: 93%, white solid, m.p. = 108–110 °C; 1H NMR (400 MHz, CDCl3) δ 12.72 (s, 1H), 7.65 (d, J = 8.8 Hz, 1H), 7.36 (q, J = 7.2 Hz, 1H), 7.18 (d, J = 7.6 Hz, 1H), 7.13 (d, J = 9.2 Hz, 1H), 7.03 (t, J = 8.4 Hz, 1H), 6.51 (dd, J = 8.8, 2.0 Hz, 1H), 6.47 (d, J = 1.2 Hz, 1H), 5.09 (s, 2H), 2.56 (s, 3H); 13C NMR (100 MHz, CDCl3) δ 202.7, 165.3, 164.9, 163.1 (d, 1J = 245 Hz), 138.6 (d, 3J = 7 Hz), 132.5, 130.4 (d, 3J = 8 Hz), 122.9 (d, 4J = 3 Hz), 115.3 (d, 2J = 21 Hz), 114.4 (d, 2J = 22 Hz), 114.4, 108.2, 102.0, 69.4, 26.3.
1–(4-((4-Fluorobenzyl)oxy)-2-hydroxyphenyl)ethan-1-one (4j)
Yield: 92%, white solid, m.p. = 115–117 °C; 1H NMR (400 MHz, CDCl3) δ 12.73 (s, 1H), 7.64 (d, J = 8.8 Hz, 1H), 7.39 (dd, J = 8.0, 5.6 Hz, 2H), 7.08 (t, J = 8.8 Hz, 2H), 6.52–6.48 (m, 2H), 5.04 (s, 2H), 2.55 (s, 3H); 13C NMR (100 MHz, CDCl3) δ 202.7, 165.3, 165.1, 162.8 (d, 1J = 245 Hz), 132.5, 131.8 (d, 4J = 3 Hz), 129.5 (d, 3J = 8 Hz), 115.8 (d, 2J = 22 Hz), 114.3, 108.2, 102.0, 69.6, 26.3.
1–(4-((3-Chlorobenzyl)oxy)-2-hydroxyphenyl)ethan-1-one (4k)
Yield: 70%, white solid, m.p. = 127–129 °C; 1H NMR (400 MHz, CDCl3) δ 12.72 (s, 1H), 7.64 (d, J = 8.8 Hz, 1H), 7.41 (s, 1H), 7.35–7.26 (m, 3H), 6.51 (dd, J = 8.8, 2.0 Hz, 1H), 6.46 (d, J = 2.0 Hz, 1H), 5.06 (s, 2H), 2.55 (s, 3H); 13C NMR (100 MHz, CDCl3) δ 202.8, 165.3, 164.9, 138.1, 134.8, 132.6, 130.1, 128.6, 127.6, 125.5, 114.4, 108.1, 102.0, 69.4, 26.3.
1–(4-((4-Chlorobenzyl)oxy)-2-hydroxyphenyl)ethan-1-one (4l)
Yield: 79%, white solid, m.p. = 102–104 °C; 1H NMR (400 MHz, CDCl3) δ 12.72 (s, 1H), 7.64 (d, J = 8.8 Hz, 1H), 7.37 (d, J = 9.2 Hz, 2H), 7.34 (d, J = 9.2 Hz, 2H), 6.50 (dd, J = 8.8, 2.0 Hz, 1H), 6.47 (d, J = 2.0 Hz, 1H), 5.05 (s, 2H), 2.56 (s, 3H); 13C NMR (100 MHz, CDCl3) δ 202.8, 165.3, 165.0, 134.5, 134.3, 132.5, 129.0, 129.0, 114.4, 108.2, 102.0, 69.5, 26.4.
General procedure for the preparation of intermediates 5a–l and 6a–e
A solution of compounds 4a–l (10 mmol) in anhydrous DMF (20 ml) was stirred at −10 °C for 35 min. Subsequently, phosphoryl chloride (20 mmol) was added dropwise for 20 min at −10 °C. Then the reaction mixture was allowed to stir at room temperature for another 15 h before being poured into ice water. The produced residue was filtered and washed with ethyl ether to obtain solid powders 5a–l. Afterward, a mixture of compounds 5a–e (3 mmol) in methylene chloride (40 ml) and sulphamic acid (15 mmol) in water (36 ml) was cooled to −0 °C. Sodium chloride (12 mmol) was dissolved in water (26 ml) and added dropwise to the mixture slowly at −0 °C. After 12 h, the reaction solution was extracted with DCM three times. The organic extracts were combined, dried with anhydrous Na2SO4, and evaporated. The products 6a–e were finally purified by recrystallisation with methanol.
4-Oxo-4H-chromene-3-carbaldehyde (5a)
Yield: 72%, yellow solid, m.p. = 149–151 °C; 1H NMR (400 MHz, CDCl3) δ 10.39 (s, 1H), 8.55 (s, 1H), 8.30 (dd, J = 8.0, 1.2 Hz, 1H), 7.78–7.73 (m, 1H), 7.55–7.48 (m, 2H); 13C NMR (100 MHz, CDCl3) δ188.7, 176.1, 160.8, 156.3, 135.0, 126.8, 126.3, 125.5, 120.5, 118.8.
7-Methyl-4-oxo-4H-chromene-3-carbaldehyde (5b)
Yield: 73%, yellow solid, m.p. = 181–183 °C; 1H NMR (400 MHz, CDCl3) δ 10.31 (d, J = 4.4 Hz, 1H), 8.44 (d, J = 2.8 Hz, 1H), 8.12–8.08 (m, 1H), 7.26 (s, 1H), 7.23 (d, J = 6.0 Hz, 1H), 2.45 (s, 3H); 13C NMR (100 MHz, CDCl3) δ 188.8, 175.9, 160.5, 156.4, 146.6, 128.1, 126.0, 123.1, 120.4, 118.5, 22.0.
6-Methyl-4-oxo-4H-chromene-3-carbaldehyde (5c)
Yield: 84%, yellow solid, m.p. = 171–173 °C; 1H NMR (400 MHz, CDCl3) δ 10.36 (s, 1H), 8.51 (s, 1H), 8.05 (s, 1H), 7.54 (dd, J = 8.8, 1.6 Hz, 1H), 7.41 (d, J = 8.4 Hz, 1H), 2.47 (s, 3H); 13C NMR (100 MHz, CDCl3) δ 188.9, 176.2, 160.6, 154.6, 137.0, 136.1, 125.6, 125.1, 120.3, 118.5, 21.1.
7- Methoxy −4-oxo-4H-chromene-3-carbaldehyde (5d)
Yield: 65%, brown solid, m.p. = 148–150 °C; 1H NMR (400 MHz, CDCl3) δ 10.37 (s, 1H), 8.47 (s, 1H), 8.19 (d, J = 8.8 Hz, 1H), 7.04 (dd, J = 8.8, 2.0 Hz, 1H), 6.91 (d, J = 2.0 Hz, 1H), 3.92 (s, 3H); 13C NMR (100 MHz, CDCl3) δ 189.0, 175.4, 165.0, 160.3, 158.1, 127.7, 120.4, 119.0, 115.6, 101.3, 56.2.
6-Methoxy − 4-oxo-4H-chromene-3-carbaldehyde (5e)
Yield: 91%, yellow solid, m.p. = 162–164 °C; 1H NMR (400 MHz, CDCl3) δ 10.38 (s, 1H), 8.51 (s, 1H), 7.62 (d, J = 2.8 Hz, 1H), 7.45 (d, J = 9.2 Hz, 1H), 7.30 (dd, J = 9.2, 3.2 Hz, 1H), 3.91 (s, 3H); 13C NMR (100 MHz, CDCl3) δ 188.8, 176.0, 160.3, 158.1, 151.1, 126.3, 124.6, 120.1, 119.7, 105.6, 56.2.
7-(Benzyloxy)-4-oxo-4H-chromene-3-carbaldehyde (5f)
Yield: 82%, brown solid, m.p. = 126–128 °C; 1H NMR (400 MHz, CDCl3) δ 10.37 (s, 1H), 8.46 (s, 1H), 8.21 (d, J = 9.2 Hz, 1H), 7.43 (d, J = 6.0 Hz, 5H), 7.12 (dd, J = 9.2, 2.0 Hz, 1H), 6.98 (d, J = 1.6 Hz, 1H), 5.18 (s, 2H); 13C NMR (100 MHz, CDCl3) δ 189.0, 175.4, 164.0, 160.3, 158.0, 135.4, 129.0, 128.7, 127.7, 127.6, 120.4, 119.2, 116.2, 102.4, 70.9.
7-((3-Methylbenzyl)oxy)-4-oxo-4H-chromene-3-carbaldehyde (5g)
Yield: 77%, brown solid, m.p. = 144–146 °C; 1H NMR (400 MHz, CDCl3) δ 10.37 (s, 1H), 8.47 (s, 1H), 8.20 (d, J = 8.9 Hz, 1H), 7.30 (td, J = 7.4, 2.5 Hz, 3H), 7.13 (d, J = 2.3 Hz, 1H), 7.11 (d, J = 2.3 Hz, 1H), 6.98 (d, J = 2.3 Hz, 1H), 5.14 (s, 2H), 2.37 (s, 3H); 13C NMR (100 MHz, CDCl3) δ 188.9, 175.3, 164.0, 160.3, 157.9, 138.7, 135.2, 129.4, 128.8, 128.3, 127.6, 124.7, 120.2, 119.0, 116.1, 102.2, 70.9, 21.5.
7-((4-Methylbenzyl)oxy)-4-oxo-4H-chromene-3-carbaldehyde (5h)
Yield: 78%, brown solid, m.p. = 158–160 °C; 1H NMR (400 MHz, CDCl3) δ10.37 (s, 1H), 8.47 (s, 1H), 8.19 (d, J = 8.9 Hz, 1H), 7.33 (d, J = 7.9 Hz, 2H), 7.22 (d, J = 7.9 Hz, 2H), 7.11 (dd, J = 8.9, 2.3 Hz, 1H), 6.98 (d, J = 2.2 Hz, 1H), 5.13 (s, 2H), 2.37 (s, 3H); 13C NMR (150 MHz, CDCl3) δ 188.8, 175.2, 163.9, 160.1, 157.8, 138.5, 132.2, 129.5, 127.6, 127.5, 120.2, 118.9, 116.0, 102.1, 70.7, 21.2.
7-((3-Fluorobenzyl)oxy)-4-oxo-4H-chromene-3-carbaldehyde (5i)
Yield: 95%, brown solid, m.p. = 119–121 °C; 1H NMR (400 MHz, CDCl3) δ 10.36 (s, 1H), 8.46 (s, 1H), 8.21 (d, J = 8.8 Hz, 1H), 7.38 (d, J = 6.0 Hz, 1H), 7.21 (d, J = 7.2 Hz, 1H), 7.15 (d, J = 9.2 Hz, 1H), 7.11 (d, J = 8.8 Hz, 1H), 7.06 (s, 1H), 6.97 (s, 1H), 5.17 (s, 2H); 13C NMR (100 MHz, CDCl3) δ 188.9, 175.3, 163.1 (d, 1J = 246 Hz), 163.7, 160.4, 158.0, 130.6 (d, 3J = 8 Hz), 127.8, 122.9 (d, 4J = 2 Hz), 120.4, 119.4, 116.0, 115.6 (d, 2J = 20 Hz), 115.4 (d, 4J = 2 Hz), 114.4 (d, 2J = 22 Hz), 102.4, 70.0.
7-((4-Fluorobenzyl)oxy)-4-oxo-4H-chromene-3-carbaldehyde (5j)
Yield: 75%, brown solid, m.p. = 118–120 °C; 1H NMR (400 MHz, CDCl3) δ 10.36 (s, 1H), 8.46 (s, 1H), 8.20 (d, J = 8.8 Hz, 1H), 7.43 (d, J = 5.6 Hz, 1H), 7.41 (d, J = 5.6 Hz, 1H), 7.11 (dd, J = 5.2, 2.8 Hz, 2H), 7.09 (d, J = 2.8 Hz, 1H), 6.97 (d, J = 2.0 Hz, 1H), 5.13 (s, 2H); 13C NMR (100 MHz, CDCl3) δ 188.9, 175.3, 162.9 (d, 1J = 246 Hz), 163.8, 160.4, 158.0, 131.2 (d, 4J = 3 Hz), 129.6 (d, 3J = 9 Hz), 127.8, 120.4, 119.3, 116.1, 115.8, 102.4, 70.2.
7-((3-Chlorobenzyl)oxy)-4-oxo-4H-chromene-3-carbaldehyde (5k)
Yield: 94%, brown solid, m.p. = 110–112 °C; 1H NMR (400 MHz, CDCl3) δ 10.37 (s, 1H), 8.47 (s, 1H), 8.22 (d, J = 8.8 Hz, 1H), 7.44 (s, 1H), 7.36–7.33 (m, 3H), 7.12 (dd, J = 8.8, 2.4 Hz, 1H), 6.97 (d, J = 2.4 Hz, 1H), 5.15 (s, 2H); 13C NMR (150 MHz, CDCl3) δ 188.7, 175.2, 163.5, 160.2, 157.8, 137.2, 134.8, 130.1, 128.7, 127.7, 127.4, 125.3, 120.2, 119.2, 115.8, 102.2, 69.8.
7-((4-Chlorobenzyl)oxy)-4-oxo-4H-chromene-3-carbaldehyde (5l)
Yield: 80%, brown solid, m.p. = 111–113 °C; 1H NMR (400 MHz, CDCl3) δ 10.35 (s, 1H), 8.46 (s, 1H), 8.20 (d, J = 8.8 Hz, 1H), 7.38 (s, 4H), 7.10 (dd, J = 8.8, 2.4 Hz, 1H), 6.96 (d, J = 2.0 Hz, 1H), 5.13 (s, 2H); 13C NMR (100 MHz, CDCl3) δ 188.9, 175.3, 163.7, 160.4, 157.9, 133.8, 132.5, 129.1, 129.0, 127.8, 120.3, 119.2, 116.0, 102.3, 70.1.
4-Oxo-4H-chromene-3-carboxylic acid (6a)
Yield: 89%, yellow solid, m.p. = 201–202 °C; 1H NMR (400 MHz, DMSO-d6) δ 13.21 (s, 1H), 9.10 (s, 1H), 8.15 (dd, J = 8.0, 1.2 Hz, 1H), 7.91 (t, J = 8.4 Hz, 1H), 7.77 (d, J = 8.4 Hz, 1H), 7.60 (t, J = 7.6 Hz, 1H); 13C NMR (100 MHz, DMSO-d6) δ 176.1, 163.8, 163.7, 155.7, 135.5, 126.8, 125.4, 123.3, 118.8, 114.3.
7-Methyl-4-oxo-4H-chromene-3-carboxylic acid (6b)
Yield: 76%, yellow solid, m.p. = 206–208 °C; 1H NMR (400 MHz, DMSO-d6) δ 13.29 (s, 1H), 9.08 (s, 1H), 8.03 (d, J = 7.2 Hz, 1H), 7.60 (s, 1H), 7.43 (d, J = 6.8 Hz, 1H), 2.50 (s, 3H); 13C NMR (100 MHz, DMSO-d6) δ 176.3, 163.8, 163.7, 155.9, 147.1, 128.2, 125.1, 120.8, 118.3, 113.8, 21.3.
6-Methyl-4-oxo-4H-chromene-3-carboxylic acid (6c)
Yield: 85%, yellow solid, m.p. = 248–250 °C; 1H NMR (400 MHz, DMSO-d6) δ 13.28 (s, 1H), 9.11 (s, 1H), 7.96 (s, 1H), 7.75 (dd, J = 8.4, 1.2 Hz,1H), 7.70 (d, J = 8.8 Hz, 1H), 2.46 (s, 3H); 13C NMR (100 MHz, DMSO-d6) δ 176.3, 163.9, 163.7, 154.1, 136.8, 136.7, 124.6, 123.0, 118.6, 114.0, 20.5.
7-Methoxy-4-oxo-4H-chromene-3-carboxylic acid (6d)
Yield: 48%, yellow solid, m.p. = 176–178 °C; 1H NMR (400 MHz, DMSO-d6) δ13.24 (s, 1H), 9.08 (s, 1H), 8.06 (d, J = 8.8 Hz, 1H), 7.29 (s, 1H), 7.18 (d, J = 8.0 Hz, 1H), 3.93 (s, 3H); 13C NMR (100 MHz, DMSO-d6) δ 176.2, 165.0, 163.8, 163.7, 157.9, 126.8, 116.5, 116.2, 113.4, 101.3, 56.4.
6-Methoxy-4-oxo-4H-chromene-3-carboxylic acid (6e)
Yield: 78%, yellow solid, m.p. = 172–174 °C; 1H NMR (400 MHz, DMSO-d6) δ 13.30 (s, 1H), 9.12 (s, 1H), 7.78 (d, J = 8.8 Hz, 1H), 7.54 (d, J = 2.8 Hz, 1H), 7.51 (s, 1H), 3.92 (s, 3H); 13C NMR (100 MHz, DMSO-d6) δ 176.1, 163.9, 163.4, 157.4, 150.5, 124.6, 124.0, 120.4, 113.2, 105.1, 55.9.
General procedure for the preparation of compounds 7a–e
The intermediates 6a–e (1 mmol) were dissolved in DMF (4 ml), then POCl3 (1.3 mmol) was added to the mixture, and stirred for 40 min at room temperature for the generation of corresponding acyl chloride. Subsequently, intermediate 3 (1 mmol) was added. After 10 h, the reaction mixture was diluted with CH2Cl2, washed with water two times, and with saturated NaHCO3 solution three times. The combined organic phases were dried over Na2SO4 and concentrated in vacuo. The target product was purified by column chromatography DCM/MeOH, and the solvents were removed under reduced pressure to give 7a–e.
N-(2–(3-((4-methoxybenzyl)oxy)-2-methyl-4-oxopyridin-1(4H)-yl)ethyl)-4-oxo-4H-chromene-3-carboxamide (7a)
Yield: 57%, yellow oil, 1H NMR (400 MHz, CDCl3) δ 9.52 (t, J = 5.2 Hz, 1H), 8.93 (s, 1H), 8.26 (d, J = 8.0 Hz, 1H), 7.78 (t, J = 8.4 Hz, 1H), 7.57 (d, J = 8.4 Hz, 1H), 7.52 (t, J = 7.6 Hz, 1H), 7.32 (d, J = 8.4 Hz, 2H), 7.19 (d, J = 7.6 Hz, 1H), 6.84 (d, J = 8.4 Hz, 2H), 6.38 (d, J = 7.2 Hz, 1H), 5.15 (s, 2H), 4.00 (t, J = 6.4 Hz, 2H), 3.78 (s, 3H), 3.61 (q, J = 6.4 Hz, 2H), 2.14 (s, 3H); 13C NMR (100 MHz, CDCl3) δ 177.2, 173.7, 163.9, 162.6, 159.6, 156.3, 146.3, 140.9, 138.5, 135.1, 131.0, 130.0, 126.7, 126.4, 124.2, 118.6, 117.5, 115.3, 113.7, 72.8, 55.4, 52.3, 39.6, 12.6; ESI-HRMS: m/z calcd for C26H24N2O6 [M + H]+: 461.1707; found: 461.1674.
N-(2–(3-((4-methoxybenzyl)oxy)-2-methyl-4-oxopyridin-1(4H)-yl)ethyl)-7-methyl-4-oxo-4H-chromene-3-carboxamide (7b)
Yield: 36%, yellow oil, 1H NMR (400 MHz, CDCl3) δ 9.57 (t, J = 5.8 Hz, 1H), 8.89 (s, 1H), 8.13 (d, J = 8.2 Hz, 1H), 7.37 − 7.30 (m, 4H), 7.22 (d, J = 7.5 Hz, 1H), 6.87 − 6.83 (m, 2H), 6.42 (d, J = 7.5 Hz, 1H), 5.15 (s, 2H), 4.01 (t, J = 6.7 Hz, 2H), 3.78 (s, 3H), 3.61 (q, J = 6.5 Hz, 2H), 2.53 (s, 3H), 2.15 (s, 3H); 13C NMR (100 MHz, CDCl3) δ 177.0, 173.6, 164.0, 162.3, 159.5, 156.3, 146.8, 146.1, 141.0, 138.5, 130.8, 129.8, 128.1, 126.0, 121.8, 118.2, 117.4, 114.9, 113.6, 72.7, 55.2, 52.2, 39.4, 21.9, 12.5.
N-(2–(3-((4-methoxybenzyl)oxy)-2-methyl-4-oxopyridin-1(4H)-yl)ethyl)-6-methyl-4-oxo-4H-chromene-3-carboxamide (7c)
Yield: 38%, yellow oil, 1H NMR (400 MHz, CDCl3) δ 9.55 (t, J = 5.8 Hz, 1H), 8.89 (s, 1H), 8.01 (s, 1H), 7.57 (dd, J = 8.6, 2.1 Hz, 1H), 7.45 (d, J = 8.6 Hz, 1H), 7.31 (d, J = 8.6 Hz, 2H), 7.20 (d, J = 7.5 Hz, 1H), 6.83 (d, J = 8.6 Hz, 2H), 6.37 (d, J = 7.5 Hz, 1H), 5.12 (s, 2H), 3.99 (t, J = 6.6 Hz, 2H), 3.77 (s, 3H), 3.60 (q, J = 6.4 Hz, 2H), 2.48 (s, 3H), 2.13 (s, 3H); 13C NMR (100 MHz, CDCl3) δ 177.1, 173.6, 164.0, 162.3, 159.5, 154.5, 146.1, 140.9, 138.5, 136.9, 136.2, 130.8, 129.8, 125.5, 123.7, 118.2, 117.4, 114.9, 113.6, 72.7, 55.2, 52.2, 39.4, 21.1, 12.5.
7-Methoxy-N-(2–(3-((4-methoxybenzyl)oxy)-2-methyl-4-oxopyridin-1(4H)-yl) ethyl)-4-oxo-4H-chromene-3-carboxamide (7d)
Yield: 32%, yellow oil, 1H NMR (400 MHz, CDCl3) δ 9.62 (t, J = 5.8 Hz, 1H), 8.85 (s, 1H), 8.15 (d, J = 9.0 Hz, 1H), 7.33 (d, J = 8.6 Hz, 2H), 7.21 (d, J = 7.5 Hz, 1H), 7.07 (dd, J = 9.0, 2.4 Hz, 1H), 6.93 (d, J = 2.3 Hz, 1H), 6.88 − 6.83 (m, 2H), 6.41 (d, J = 7.5 Hz, 1H), 5.15 (s, 2H), 4.00 (t, J = 6.7 Hz, 2H), 3.94 (s, 3H), 3.79 (s, 3H), 3.61 (q, J = 6.5 Hz, 2H), 2.15 (s, 3H); 13C NMR (100 MHz, CDCl3) δ 176.4, 173.4, 165.1, 164.0, 162.0, 159.5, 158.0, 141.2, 138.6, 130.9, 130.9, 129.2, 128.0, 117.3, 117.3, 116.0, 114.9, 113.6, 100.5, 73.2, 56.1, 55.3, 52.2, 39.4, 12.5; ESI-HRMS: m/z calcd for C27H26N2O7 [M + H]+: 491.1813; found: 491.1826.
6-Methoxy-N-(2–(3-((4-methoxybenzyl)oxy)-2-methyl-4-oxopyridin-1(4H)-yl) ethyl)-4-oxo-4H-chromene-3-carboxamide (7e)
Yield: 30%, yellow oil, 1H NMR (400 MHz, CDCl3) δ 9.57 (t, J = 5.7 Hz, 1H), 8.92 (s, 1H), 7.59 (d, J = 3.0 Hz, 1H), 7.51 (d, J = 9.2 Hz, 1H), 7.37 − 7.31 (m, 3H), 7.21 (d, J = 7.5 Hz, 1H), 6.87 − 6.82 (m, 2H), 6.41 (d, J = 7.5 Hz, 1H), 5.15 (s, 2H), 4.01 (t, J = 6.5 Hz, 2H), 3.92 (s, 3H), 3.79 (s, 3H), 3.62 (q, J = 6.3 Hz, 2H), 2.15 (s, 3H); 13C NMR (100 MHz, CDCl3) δ 176.8, 173.6, 164.0, 162.1, 159.5, 157.9, 151.0, 146.1, 141.0, 138.5, 130.9, 129.8, 124.9, 124.8, 119.9, 117.4, 114.3, 113.6, 105.3, 72.7, 56.0, 55.3, 52.2, 39.4, 12.6; ESI-HRMS: m/z calcd for C27H26N2O7 [M + H]+: 491.1813; found: 491.1821.
General procedure for the preparation of compounds 8a–e, 15a–g, and 17a–l
The chromone derivatives 7a–e, 14a–g, and 16a–l (0.3 mmol) were dissolved in anhydrous DCM (10 ml) and protected by nitrogen. After the solution was cooled to −48 °C, BCl3 (1 M in DCM, 1.5 eq) dissolved in anhydrous DCM (10 ml) was added dropwise slowly. After the addition was completed, the mixture was stirred for 12 h at room temperature. The excess boron trichloride was quenched with methanol (10 ml) and left to stir for another 1 h. After the removal of solvents in a high vacuum, the residues were purified by recrystallisation from methanol/ether to afford the final compounds 8a–e, 15a–g, and 17a–l as solids.
N-(2–(3-hydroxy-2-methyl-4-oxopyridin-1(4H)-yl)ethyl)-4-oxo-4H-chromene-3-carboxamide (8a)
Yield: 93%, yellow solid, m.p. = 268–270 °C; 1H NMR (400 MHz, DMSO-d6) δ 9.30 (t, J = 6.0 Hz, 1H), 8.90 (s, 1H), 8.16 (d, J = 8.0 Hz, 1H), 8.09 (d, J = 6.8 Hz, 1H), 7.91 (t, J = 7.6 Hz, 1H), 7.76 (d, J = 8.4 Hz, 1H), 7.60 (t, J = 7.6 Hz, 1H), 7.30 (d, J = 6.8 Hz, 1H), 4.5 3 (t, J = 5.6 Hz, 2H), 4.01 (q, J = 6.0 Hz, 2H), 2.59 (s, 3H); 13C NMR (100 MHz, DMSO-d6) δ 176.0, 162.9, 162.7, 158.8, 155.6, 142.8, 141.8, 138.6, 135.3, 126.6, 125.4, 123.5, 118.69, 115.1, 110.5, 55.2, 38.3, 12.6; ESI-HRMS: m/z calcd for C18H16N2O5 [M + H]+: 341.1132; found: 341.1125. HPLC purity: 97.9%.
N-(2–(3-hydroxy-2-methyl-4-oxopyridin-1(4H)-yl)ethyl)-7-methyl-4-oxo-4H-chromene-3-carboxamide (8b)
Yield: 94.5%, yellow solid, m.p. = 270–272 °C; 1H NMR (400 MHz, DMSO-d6) δ 9.33 (t, J = 6.1 Hz, 1H), 8.95 (s, 1H), 8.07 (dd, J = 10.1, 7.6 Hz, 2H), 7.60 (s, 1H), 7.44 (d, J = 8.2 Hz, 1H), 7.24 (d, J = 6.8 Hz, 1H), 4.53 (t, J = 5.8 Hz, 2H), 3.78 (q, J = 5.9 Hz, 2H), 2.59 (s, 3H), 2.50 (s, 3H); 13C NMR (100 MHz, DMSO-d6) δ 175.8, 162.8, 162.7, 158.8, 155.7, 146.5, 142.8, 141.7, 138.5, 128.0, 125.2, 121.3, 118.2, 114.9, 110.5, 55.2, 38.2, 21.3, 12.6; ESI-HRMS: m/z calcd for C19H18N2O5 [M + H]+: 355.1288; found: 355.1276. HPLC purity: 98.9%.
N-(2–(3-hydroxy-2-methyl-4-oxopyridin-1(4H)-yl)ethyl)-6-methyl-4-oxo-4H-chromene-3-carboxamide (8c)
Yield: 98.5%, yellow solid, m.p. = 265–267 °C; 1H NMR (400 MHz, DMSO-d6) δ 9.32 (t, J = 6.1 Hz, 1H), 8.97 (s, 1H), 8.09 (d, J = 7.0 Hz, 1H), 7.95 (s, 1H), 7.74 (dd, J = 8.6, 1.9 Hz, 1H), 7.68 (d, J = 8.6 Hz, 1H), 7.29 − 7.23 (m, 1H), 4.54 (t, J = 5.8 Hz, 2H), 3.78 (q, J = 5.5, 5.1 Hz, 2H), 2.60 (s, 3H), 2.47 (s, 3H); 13C NMR (100 MHz, DMSO-d6) δ 176.4, 163.3, 163.2, 159.3, 154.4, 143.3, 142.3, 139.0, 136.9, 136.8, 125.0, 123.7, 119.0, 115.4, 111.0, 55.6, 38.7, 21.0, 13.1. ESI-HRMS: m/z calcd for C19H18N2O5 [M + H]+: 355.1288; found: 355.1273. HPLC purity: 99.1%.
N-(2–(3-hydroxy-2-methyl-4-oxopyridin-1(4H)-yl)ethyl)-7-methoxy-4-oxo-4H-chromene-3-carboxamide (8d)
Yield: 94.6%, yellow solid, m.p. = 248–250 °C; 1H NMR (400 MHz, DMSO-d6) δ 9.36 (t, J = 6.1 Hz, 1H), 8.91 (s, 1H), 8.06 (dd, J = 7.9, 3.3 Hz, 2H), 7.27 (d, J = 2.3 Hz, 1H), 7.21 (d, J = 6.9 Hz, 1H), 7.17 (dd, J = 8.9, 2.3 Hz, 1H), 4.52 (t, J = 5.7 Hz, 2H), 3.92 (s, 3H), 3.76 (q, J = 6.8, 6.3 Hz, 2H), 2.58 (s, 3H); 13C NMR (100 MHz, DMSO-d6) δ 175.7, 165.0, 163.3, 162.9, 159.3, 158.0, 143.3, 142.2, 139.0, 127.3, 117.6, 116.4, 115.3, 111.0, 101.5, 56.9, 55.6, 38.7, 13.1; ESI-HRMS: m/z calcd for C19H18N2O6 [M + H]+: 371.1238; found: 371.1227. HPLC purity: 99.7%.
N-(2–(3-hydroxy-2-methyl-4-oxopyridin-1(4H)-yl)ethyl)-6-methoxy-4-oxo-4H-chromene-3-carboxamide (8e)
Yield: 98.3%, yellow solid, m.p. = 260–262 °C; 1H NMR (400 MHz, DMSO-d6) δ 9.32 (t, J = 6.1 Hz, 1H), 8.97 (s, 1H), 8.08 (d, J = 7.0 Hz, 1H), 7.77 − 7.73 (m, 1H), 7.51 (dd, J = 7.3, 2.9 Hz, 2H), 7.24 (d, J = 7.0 Hz, 1H), 4.53 (t, J = 5.8 Hz, 2H), 3.89 (s, 3H), 3.78 (q, J = 6.0 Hz, 2H), 2.59 (s, 3H); 13C NMR (100 MHz, DMSO-d6) δ 176.1, 163.4, 163.0, 159.2, 157.8, 150.8, 143.3, 142.3, 139.0, 124.8, 124.6, 120.8, 114.8, 111.0, 105.8, 56.4, 55.7, 38.7, 13.1; ESI-HRMS: m/z calcd for C19H18N2NaO6 [M + Na]+: 393.1057; found: 393.1057. HPLC purity: 98.4%.
7-(Benzyloxy)-N-(2–(3-hydroxy-2-methyl-4-oxopyridin-1(4H)-yl)ethyl)-4-oxo-4H-chromene-3-carboxamide (15a)
Yield: 54.7%, yellow solid, m.p. = 225–227 °C; 1H NMR (400 MHz, DMSO-d6) δ 9.37 (t, J = 6.1 Hz, 1H), 8.92 (s, 1H), 8.07 (t, J = 7.8 Hz, 2H), 7.50 (d, J = 7.0 Hz, 2H), 7.46 − 7.36 (m, 4H), 7.26 (dd, J = 8.9, 2.3 Hz, 1H), 7.17 (d, J = 6.9 Hz, 1H), 5.31 (s, 2H), 4.52 (t, J = 5.7 Hz, 2H), 3.77 (q, J = 7.4, 6.5 Hz, 2H), 2.59 (s, 3H); 13C NMR (100 MHz, DMSO-d6) δ 175.7, 164.0, 163.3, 163.0, 159.1, 157.9, 143.3, 142.3, 139.0, 136.3, 129.1, 128.7, 128.5, 127.4, 117.7, 116.9, 115.3, 110.9, 102.4, 70.8, 55.7, 38.7, 13.1; ESI-HRMS: m/z calcd for C25H22N2O6 [M + H]+: 447.1551; found: 447.1539. HPLC purity: 99.7%.
N-(2–(3-hydroxy-2-methyl-4-oxopyridin-1(4H)-yl)ethyl)-7-((3-methylbenzyl) oxy)-4-oxo-4H-chromene-3-carboxamide (15b)
Yield: 28%, yellow solid, m.p. = 238–240 °C; 1H NMR (400 MHz, DMSO-d6) δ 1H NMR (400 MHz, DMSO-d6) δ 9.37 (t, J = 6.1 Hz, 1H), 8.92 (s, 1H), 8.07 (dd, J = 7.9, 4.0 Hz, 2H), 7.36 − 7.34 (m, 1H), 7.33 − 7.21 (m, 5H), 7.17 (d, J = 6.9 Hz, 1H), 5.24 (s, 2H), 4.52 (t, J = 5.6 Hz, 2H), 3.76 (q, J = 5.6 Hz, 2H), 2.58 (s, 3H), 2.32 (s, 3H). 13C NMR (100 MHz, DMSO-d6) δ 175.3, 163.6, 162.9, 162.6, 158.9, 157.5, 142.9, 141.7, 138.6, 137.9, 135.8, 129.0, 128.6, 128.6, 127.0, 125.2, 117.3, 116.5, 114.9, 110.5, 102.0, 70.4, 55.2, 38.3, 21.1, 12.7. ESI-HRMS: m/z calcd for C26H24N2O6 [M + H]+: 461.1707; found: 461.1708. HPLC purity: 98.3%.
N-(2–(3-hydroxy-2-methyl-4-oxopyridin-1(4H)-yl)ethyl)-7-((4-methylbenzyl)oxy)-4-oxo-4H-chromene-3-carboxamide (15c)
Yield: 23%, yellow solid, m.p. = 234–236 °C; 1H NMR (400 MHz, DMSO-d6) δ 9.37 (t, J = 6.1 Hz, 1H), 8.92 (s, 1H), 8.05 (dd, J = 7.8, 5.3 Hz, 2H), 7.40 − 7.33 (m, 3H), 7.22 (d, J = 8.0 Hz, 3H), 7.15 (d, J = 6.9 Hz, 1H), 5.24 (s, 2H), 4.50 (t, J = 5.5 Hz, 2H), 3.77 − 3.73 (m, 2H), 2.57 (s, 3H), 2.31 (s, 3H). 13C NMR (100 MHz, DMSO-d6) δ 175.3, 163.6, 162.9, 162.6, 159.1, 157.5, 143.0, 141.4, 138.6, 137.7, 132.9, 129.2, 128.2, 127.0, 117.2, 116.5, 114.9, 110.5, 102.0, 70.3, 55.2, 38.3, 20.9, 12.6. ESI-HRMS: m/z calcd for C26H24N2O6 [M + H]+: 461.1707; found: 461.1724. HPLC purity: 98.2%.
7-((3-Fluorobenzyl)oxy)-N-(2–(3-hydroxy-2-methyl-4-oxopyridin-1(4H)-yl)ethyl)-4-oxo-4H-chromene-3-carboxamide (15d)
Yield: 38%, yellow solid, m.p. = 237–239 °C; 1H NMR (400 MHz, DMSO-d6) δ 9.37 (t, J = 6.1 Hz, 1H), 8.93 (s, 1H), 8.08 (d, J = 8.7 Hz, 2H), 7.51 − 7.44 (m, 1H), 7.36 (dd, J = 11.7, 4.9 Hz, 3H), 7.29 − 7.18 (m, 3H), 5.33 (s, 2H), 4.53 (t, J = 5.7 Hz, 2H), 3.77 (q, J = 6.0 Hz, 2H), 2.59 (s, 3H). 13C NMR (100 MHz, DMSO-d6) δ 175.3, 163.3, 162.9, 162.6, 162.2 (d, 1J = 242 Hz), 158.7, 157.4, 142.9, 141.9, 138.8 (d, 3J = 8 Hz), 138.6, 130.7 (d, 3J = 8 Hz), 127.0, 123.9 (d, 4J = 2 Hz), 117.4, 116.4, 115.1 (d, 2J = 21 Hz), 114.9, 114.6 (d, 2J = 22 Hz), 110.5, 102.1, 69.4, 55.3, 38.3, 12.7. ESI-HRMS: m/z calcd for C25H21FN2O6 [M + H]+: 465.1456; found: 465.1475. HPLC purity: 98%.
7-((4-Fluorobenzyl)oxy)-N-(2–(3-hydroxy-2-methyl-4-oxopyridin-1(4H)-yl) ethyl)-4-oxo-4H-chromene-3-carboxamide (15e)
Yield: 35%, yellow solid, m.p. = 246–248 °C; 1H NMR (400 MHz, DMSO-d6) δ 9.36 (t, J = 6.2 Hz, 1H), 8.91 (s, 1H), 8.07 (dd, J = 8.0, 3.9 Hz, 2H), 7.57 − 7.54 (m, 2H), 7.36 (d, J = 2.3 Hz, 1H), 7.27 − 7.23 (m, 3H), 7.19 (d, J = 6.9 Hz, 1H), 5.28 (s, 2H), 4.52 (t, J = 5.9 Hz, 2H), 3.76 (q, J = 6.0 Hz, 2H), 2.58 (s, 3H); 13C NMR (100 MHz, DMSO-d6) δ 175.7, 163.9, 163.7, 163.3, 163.0, 161.2, 159.1, 157.9, 143.3, 142.4, 139.1, 132.6 (d, 4J = 3 Hz), 130.9 (d, 3J = 9 Hz), 127.4, 116.9, 116.5 (d, 1J = 244 Hz), 115.9 (d, 2J = 22 Hz), 110.9, 102.4, 70.0, 55.7, 38.7, 13.1. ESI-HRMS: m/z calcd for C25H22FN2O6 [M + H]+: 465.1456; found: 465.1470. HPLC purity: 99.7%.
7-((3-Chlorobenzyl)oxy)-N-(2–(3-hydroxy-2-methyl-4-oxopyridin-1(4H)-yl)ethyl)-4-oxo-4H-chromene-3-carboxamide (15f)
Yield: 55%, yellow solid, m.p. = 230–232 °C; 1H NMR (400 MHz, DMSO-d6) δ 9.36 (t, J = 6.1 Hz, 1H), 8.91 (s, 1H), 8.07 (d, J = 8.7 Hz, 2H), 7.57 (s, 1H), 7.45 (t, J = 4.4 Hz, 3H), 7.36 (d, J = 2.1 Hz, 1H), 7.28 − 7.21 (m, 2H), 5.31 (s, 2H), 4.52 (t, J = 5.5 Hz, 2H), 3.76 (q, J = 6.8, 6.1 Hz, 2H), 2.58 (s, 3H). 13C NMR (100 MHz, DMSO-d6) δ 175.7, 163.7, 163.3, 163.1, 159.2, 157.8, 143.3, 142.2, 139.0, 138.9, 133.7, 131.0, 128.6, 128.1, 127.5, 127.0, 117.9, 116.8, 115.3, 111.0, 102.5, 69.7, 55.6, 38.7, 13.1; ESI-HRMS: m/z calcd for C25H21ClN2O6 [M + H]+: 481.1161; found: 481.1167. HPLC purity: 99.7%.
7-((4-Chlorobenzyl)oxy)-N-(2–(3-hydroxy-2-methyl-4-oxopyridin-1(4H)-yl)ethyl)-4-oxo-4H-chromene-3-carboxamide (15g)
Yield: 23%, yellow solid, m.p. = 241–243 °C; 1H NMR (400 MHz, DMSO-d6) δ 9.36 (t, J = 6.1 Hz, 1H), 8.91 (s, 1H), 8.07 (dd, J = 7.9, 3.9 Hz, 2H), 7.54 − 7.46 (m, 4H), 7.36 (d, J = 2.2 Hz, 1H), 7.26 − 7.18 (m, 2H), 5.30 (s, 2H), 4.52 (t, J = 5.7 Hz, 2H), 3.76 (q, J = 5.7 Hz, 2H), 2.58 (s, 3H). 13C NMR (100 MHz, DMSO-d6) δ 175.7, 163.8, 163.3, 163.0, 159.3, 157.9, 143.3, 142.1, 139.1, 135.4, 133.3, 130.3, 129.1, 127.5, 117.8, 116.9, 115.3, 110.9, 102.5, 69.9, 55.6, 38.7, 13.1; ESI-HRMS: m/z calcd for C25H21ClN2O6 [M + H]+: 481.1161; found: 481.1170. HPLC purity: 98.1%.
3-Hydroxy-2-methyl-1–(2-(((4-oxo-4H-chromen-3-yl)methyl)amino)ethyl)pyridin-4(1H)-one (17a)
Yield: 92%, yellow solid, m.p. = 248–250 °C; 1H NMR (400 MHz, DMSO-d6) δ 9.81 (s, 2H), 8.72 (s, 1H), 8.28 (d, J = 7.0 Hz, 1H), 8.12 (dd, J = 8.0, 1.5 Hz, 1H), 7.88 (ddd, J = 8.7, 7.2, 1.7 Hz, 1H), 7.73 (d, J = 8.4 Hz, 1H), 7.59 − 7.55 (m, 1H), 7.31 (d, J = 6.9 Hz, 1H), 4.74 (t, J = 6.8 Hz, 2H), 4.05 (s, 2H), 3.45 (s, 2H), 2.56 (s, 3H). 13C NMR (100 MHz, DMSO-d6) δ 175.9, 159.3, 158.7, 155.9, 143.1, 142.1, 138.6, 134.9, 126.1, 125.1, 123.0, 118.7, 115.1, 111.0, 51.5, 45.0, 41.4, 13.0. ESI-HRMS: m/z calcd for C18H18N2O4 [M + H]+: 327.1339; found: 327.1332. HPLC purity: 99.7%.
3-Hydroxy-2-methyl-1–(2-(((7-methyl-4-oxo-4H-chromen-3-yl)methyl)amino)ethyl)pyridin-4(1H)-one (17b)
Yield: 92.9%, yellow solid, m.p. = 251–253 °C; 1H NMR (400 MHz, DMSO-d6) δ 9.80 (s, 2H), 8.69 − 8.64 (m, 1H), 8.32 − 8.24 (m, 1H), 7.99 (d, J = 8.1 Hz, 1H), 7.55 (s, 1H), 7.36 (dd, J = 24.6, 8.2 Hz, 2H), 4.78 − 4.68 (m, 2H), 4.03 (s, 2H), 3.44 (s, 2H), 2.56 (s, 3H), 2.48 (s, 3H). 13C NMR (100 MHz, DMSO-d6) δ 176.2, 159.7, 158.9, 156.5, 146.5, 143.5, 142.4, 139.1, 127.9, 125.3, 121.3, 118.6, 115.4, 111.4, 52.0, 45.5, 42.0, 21.7, 13.4. ESI-HRMS: m/z calcd for C19H20N2O4 [M + H]+: 341.1496; found: 341.1490. HPLC purity: 99.1%.
3-Hydroxy-2-methyl-1–(2-(((6-methyl-4-oxo-4H-chromen-3-yl)methyl)amino) ethyl)pyridin-4(1H)-one (17c)
Yield: 77.8%, yellow solid, m.p. = 259–261 °C; 1H NMR (400 MHz, DMSO-d6) δ 9.87 (s, 2H), 8.69 (s, 1H), 8.29 (d, J = 7.0 Hz, 1H), 7.89 (s, 1H), 7.69 (dd, J = 8.6, 2.0 Hz, 1H), 7.62 (d, J = 8.6 Hz, 1H), 7.32 (d, J = 7.0 Hz, 1H), 4.74 (t, J = 6.7 Hz, 2H), 4.04 (s, 2H), 3.44 (t, J = 5.9 Hz, 2H), 2.56 (s, 3H), 2.45 (s, 3H); 13C NMR (100 MHz, DMSO-d6) δ 176.4, 159.8, 159.0, 154.7, 143.6, 142.3, 139.1, 136.4, 136.3, 124.7, 123.2, 118.9, 115.3, 111.4, 51.9, 45.5, 42.0, 20.9, 13.4. ESI-HRMS: m/z calcd for C19H20N2O4 [M + H]+: 341.1496; found: 341.1490. HPLC purity: 99.2%.
3-Hydroxy-1–(2-(((7-methoxy-4-oxo-4H-chromen-3-yl)methyl)amino)ethyl)-2-methylpyridin-4(1H)-one (17d)
Yield: 89.6%, yellow solid, m.p. = 252–254 °C; 1H NMR (400 MHz, DMSO-d6) δ 9.74 (s, 2H), 8.63 (s, 1H), 8.28 (d, J = 7.0 Hz, 1H), 8.01 (d, J = 8.9 Hz, 1H), 7.31 (d, J = 7.0 Hz, 1H), 7.22 (d, J = 2.3 Hz, 1H), 7.13 (dd, J = 8.9, 2.3 Hz, 1H), 4.73 (t, J = 6.7 Hz, 2H), 4.02 (s, 2H), 3.91 (s, 3H), 3.44 (s, 2H), 2.56 (s, 3H); 13C NMR (100 MHz, DMSO-d6) δ 175.6, 164.7, 159.8, 158.6, 158.3, 143.6, 142.4, 139.1, 127.0, 117.2, 115.8, 115.3, 111.4, 101.4, 56.8, 52.0, 45.5, 42.0, 13.4. ESI-HRMS: m/z calcd for C19H20N2O5 [M + H]+: 357.1445; found: 357.1460. HPLC purity: 97.3%.
3-Hydroxy-1–(2-(((6-methoxy-4-oxo-4H-chromen-3-yl)methyl)amino)ethyl)-2-methylpyridin-4(1H)-one (17e)
Yield: 90.6%, yellow solid, m.p. = 267–269 °C; 1H NMR (400 MHz, DMSO-d6) δ 9.89 (s, 2H), 8.71 (s, 1H), 8.31 (d, J = 7.0 Hz, 1H), 7.70 (d, J = 9.1 Hz, 1H), 7.53 − 7.41 (m, 2H), 7.36 (d, J = 7.0 Hz, 1H), 4.75 (t, J = 6.6 Hz, 2H), 4.05 (s, 2H), 3.87 (s, 3H), 3.45 (s, 2H), 2.56 (s, 3H). 13C NMR (100 MHz, DMSO-d6) δ 175.7, 159.3, 158.5, 157.0, 150.7, 143.1, 142.0, 138.7, 123.9, 123.8, 120.4, 114.2, 111.0, 104.8, 55.9, 51.6, 45.1, 41.5, 13.0. ESI-HRMS: m/z calcd for C19H20N2O5 [M + H]+: 357.1445; found: 357.1448. HPLC purity: 99.3%.
1–(2-(((7-(Benzyloxy)-4-oxo-4H-chromen-3-yl)methyl)amino)ethyl)-3-hydroxy-2-methylpyridin-4(1H)-one (17f)
Yield: 54.4%, yellow solid, m.p. = 169–171 °C; 1H NMR (400 MHz, DMSO-d6) δ 9.73 (s, 2H), 8.62 (s, 1H), 8.27 (d, J = 7.0 Hz, 1H), 8.02 (d, J = 8.9 Hz, 1H), 7.49 (d, J = 7.0 Hz, 2H), 7.44 − 7.27 (m, 5H), 7.21 (dd, J = 8.9, 2.3 Hz, 1H), 5.29 (s, 2H), 4.72 (t, J = 6.6 Hz, 2H), 4.02 (s, 2H), 3.43 (s, 2H), 2.55 (s, 3H); 13C NMR (100 MHz, DMSO-d6) δ 175.6, 163.7, 159.8, 158.6, 158.2, 143.6, 142.4, 139.1, 136.4, 129.0, 128.7, 128.4, 127.0, 117.4, 116.3, 115.3, 111.4, 102.4, 70.7, 52.0, 45.5, 42.0, 13.4. ESI-HRMS: m/z calcd for C25H24N2O5 [M + H]+: 433.1758; found: 433.1769. HPLC purity: 98.3%.
3-Hydroxy-2-methyl-1–(2-(((7-((3-methylbenzyl)oxy)-4-oxo-4H-chromen-3-yl)methyl)amino)ethyl)pyridin-4(1H)-one (17g)
Yield: 35.9%, yellow solid, m.p. = 211–213 °C; 1H NMR (400 MHz, DMSO-d6) δ 9.72 (s, 2H), 8.62 (s, 1H), 8.25 (d, J = 7.0 Hz, 1H), 8.02 (d, J = 8.9 Hz, 1H), 7.32 − 7.25 (m, 5H), 7.22 − 7.16 (m, 2H), 5.24 (s, 2H), 4.71 (t, J = 6.5 Hz, 2H), 4.02 (s, 2H), 3.43 (s, 2H), 2.55 (s, 3H), 2.33 (s, 3H); 13C NMR (100 MHz, DMSO-d6) δ 175.6, 163.7, 160.1, 158.6, 158.2, 143.6, 142.0, 139.1, 138.2, 136.3, 129.3, 129.0, 128.9, 127.0, 125.5, 117.3, 116.3, 115.3, 111.5, 102.4, 70.7, 51.9, 45.5, 42.0, 21.5, 13.4. ESI-HRMS: m/z calcd for C26H26N2O5 [M + H]+: 447.1914; found: 447.1934. HPLC purity: 99.7%.
3-Hydroxy-2-methyl-1–(2-(((7-((4-methylbenzyl)oxy)-4-oxo-4H-chromen-3-yl)methyl)amino)ethyl)pyridin-4(1H)-one (17h)
Yield: 90.1%, yellow solid, m.p. = 134–136 °C; 1H NMR (400 MHz, DMSO-d6) δ 9.64 (s, 2H), 8.60 (s, 1H), 8.22 (d, J = 5.6 Hz, 1H), 8.01 (d, J = 8.9 Hz, 1H), 7.37 (d, J = 8.0 Hz, 2H), 7.30 (d, J = 2.3 Hz, 1H), 7.24 − 7.17 (m, 4H), 5.23 (s, 2H), 4.69 (t, J = 5.7 Hz, 2H), 4.02 (s, 2H), 3.43 (s, 2H), 2.54 (s, 3H), 2.31 (s, 3H); 13C NMR (100 MHz, DMSO-d6) δ 175.6, 163.7, 160.0, 158.6, 158.2, 143.6, 142.1, 139.1, 138.0, 133.4, 129.6, 128.6, 127.0, 117.3, 116.3, 115.3, 111.5, 102.4, 70.6, 51.9, 45.5, 41.9, 21.3, 13.4. ESI-HRMS: m/z calcd for C26H26N2O5 [M + H]+: 447.1914; found: 447.1908. HPLC purity: 98%.
1–(2-(((7-((3-Fluorobenzyl)oxy)-4-oxo-4H-chromen-3-yl)methyl)amino)ethyl)-3-hydroxy-2-methylpyridin-4(1H)-one (17i)
Yield: 62.8%, yellow solid, m.p. = 215–217 °C; 1H NMR (400 MHz, DMSO-d6) δ 9.78 (s, 2H), 8.63 (s, 1H), 8.27 (d, J = 7.0 Hz, 1H), 8.03 (d, J = 8.9 Hz, 1H), 7.47 (q, J = 7.6 Hz, 1H), 7.35 − 7.28 (m, 4H), 7.20 (ddd, J = 14.5, 8.2, 2.0 Hz, 2H), 5.32 (s, 2H), 4.73 (t, J = 6.7 Hz, 2H), 4.02 (s, 2H), 3.43 (s, 2H), 2.55 (s, 3H); 13C NMR (100 MHz, DMSO-d6) δ 175.2, 163.0, 162.2 (d, 1J = 243 Hz), 159.5, 158.2, 157.7, 143.2, 141.8, 138.9 (d, 3J = 7 Hz), 138.7, 130.7 (d, 3J = 9 Hz), 126.7, 123.9 (d, 4J = 3 Hz), 117.1, 115.9, 115.0 (d, 2J = 21 Hz), 114.9, 114.6 (d, 2J = 22 Hz), 111.0, 102.1, 69.3, 51.5, 45.1, 41.6, 13.0. ESI-HRMS: m/z calcd for C25H23FN2O5 [M + H]+: 451.1664; found: 451.1676. HPLC purity: 97.9%.
1–(2-(((7-((4-Fluorobenzyl)oxy)-4-oxo-4H-chromen-3-yl)methyl)amino)ethyl)-3-hydroxy-2-methylpyridin-4(1H)-one (17j)
Yield: 43.5%, yellow solid, m.p. = 218–220 °C; 1H NMR (400 MHz, DMSO-d6) δ 9.74 (s, 2H), 8.62 (s, 1H), 8.26 (d, J = 7.0 Hz, 1H), 8.02 (d, J = 8.9 Hz, 1H), 7.55 (dd, J = 8.4, 5.6 Hz, 2H), 7.32 (d, J = 2.2 Hz, 1H), 7.24 (ddd, J = 21.6, 7.9, 6.0 Hz, 4H), 5.27 (s, 2H), 4.72 (t, J = 6.6 Hz, 2H), 4.02 (s, 2H), 3.44 (s, 2H), 2.55 (s, 3H); 13C NMR (100 MHz, DMSO-d6) δ175.6, 163.5, 162.4 (d, 1J = 242 Hz), 159.6, 158.6, 158.1, 143.5, 142.6, 139.1, 132.7 (d, 4J = 3 Hz), 130.8 (d, 3J = 8 Hz), 127.1, 117.4, 116.3, 115.9 (d, 2J = 21 Hz), 115.3, 111.4, 102.4, 69.9, 52.0, 45.5, 42.0, 13.4. ESI-HRMS: m/z calcd for C25H23FN2O5 [M + H]+: 451.1664; found: 451.1683. HPLC purity: 98%.
1–(2-(((7-((3-Chlorobenzyl)oxy)-4-oxo-4H-chromen-3-yl)methyl)amino)ethyl)-3-hydroxy-2-methylpyridin-4(1H)-one (17k)
Yield: 63.9%, yellow solid, m.p. = 226–228 °C; 1H NMR (400 MHz, DMSO-d6) δ 9.63 (s, 2H), 8.61 (s, 1H), 8.22 (d, J = 7.0 Hz, 1H), 8.03 (d, J = 8.9 Hz, 1H), 7.57 (s, 1H), 7.47 − 7.42 (m, 3H), 7.32 (d, J = 2.3 Hz, 1H), 7.24 − 7.20 (m, 2H), 5.31 (s, 2H), 4.69 (t, J = 6.8 Hz, 2H), 4.02 (s, 2H), 3.43 (s, 2H), 2.54 (s, 3H); 13C NMR (100 MHz, DMSO-d6) δ 175.6, 163.4, 160.2, 158.6, 158.1, 143.7, 141.9, 139.1, 139.0, 133.7, 131.0, 128.6, 128.1, 127.1, 126.9, 117.5, 116.3, 115.3, 111.5, 102.5, 69.6, 51.9, 45.6, 42.0, 13.3. ESI-HRMS: m/z calcd for C25H23ClN2O5 [M + H]+: 467.1368; found: 467.1388. HPLC purity: 99.7%.
1–(2-(((7-((4-Chlorobenzyl)oxy)-4-oxo-4H-chromen-3-yl)methyl)amino)ethyl)-3-hydroxy-2-methylpyridin-4(1H)-one (17l)
Yield: 65%, yellow solid, m.p. = 189–191 °C; 1H NMR (400 MHz, DMSO-d6) δ 9.68 (s, 2H), 8.61 (s, 1H), 8.25 (d, J = 7.0 Hz, 1H), 8.02 (d, J = 8.9 Hz, 1H), 7.52 (d, J = 8.5 Hz, 2H), 7.48 (d, J = 8.5 Hz, 2H), 7.31 (d, J = 2.3 Hz, 1H), 7.25 (d, J = 6.8 Hz, 1H), 7.21 (dd, J = 8.9, 2.4 Hz, 1H), 5.29 (s, 2H), 4.71 (t, J = 6.7 Hz, 2H), 4.02 (s, 2H), 3.43 (s, 2H), 2.55 (s, 3H); 13C NMR (100 MHz, DMSO-d6) δ 175.6, 163.5, 160.0, 158.6, 158.1, 143.6, 142.1, 139.1, 135.5, 133.3, 130.3, 129.1, 127.1, 117.5, 116.3, 115.3, 111.5, 102.5, 69.8, 51.9, 45.5, 42.0, 13.4. ESI-HRMS: m/z calcd for C25H23ClN2O5 [M + H]+: 467.1368; found: 467.1363. HPLC purity: 99.7%.
General procedure for the preparation of compounds 10 and 11
The synthesis procedure of compound 10 was the same as that of compounds 5a–l. A mixture of compound 10 (10 mmol), and triethylamine (20 mmol) in anhydrous DCM (80 ml) was cooled to 0 °C for a few minutes. Then acetyl chloride (10 mmol) dissolved in anhydrous DCM was added dropwise. After the addition was finished, the reaction mixture was stirred for 3 h at room temperature. The reaction solution was washed by water three times, evaporated under vacuum, and purified by column chromatography hexane/ethyl acetate. The target product 11 was obtained by removing the solvent in vacuo.
7-Hydroxy-4-oxo-4H-chromene-3-carbaldehyde (10)
Yield: 51%, Rufous solid, m.p. = 265–267 °C; 1H NMR (400 MHz, DMSO-d6) δ 11.07 (s, 1H), 10.10 (s, 1H), 8.76 (s, 1H), 7.96 (d, J = 8.8 Hz, 1H), 6.98 (d, J = 8.8 Hz, 1H), 6.93 (s, 1H); 13C NMR (100 MHz, DMSO-d6) δ 188.7, 174.1, 163.5, 162.7, 157.4, 127.1, 119.7, 117.0, 116.0, 103.1.
3-Formyl-4-oxo-4H-chromen-7-yl acetate (11)
Yield: 54.3%, yellow solid, m.p. = 168–170 °C; 1H NMR (400 MHz, CDCl3) δ 10.40 (s, 1H), 8.56 (s, 1H), 8.34 (d, J = 8.7 Hz, 1H), 7.40 (d, J = 1.9 Hz, 1H), 7.27 (d, J = 2.0 Hz, 1H), 2.40 (s, 3H). 13C NMR (100 MHz, CDCl3) δ 189.1, 175.4, 165.0, 160.4, 158.1, 127.6, 120.3, 118.9, 115.7, 101.2, 56.2.
General procedure for the preparation of compounds 12 and 13
The synthesis procedure of compound 12 was the same as that of compounds 6a–e. A solution of intermediate 12 (1.0 mmol) in anhydrous CH2Cl2 (10 ml) was treated with DMF (2 drops). After stirring for a few minutes at room temperature, oxalyl chloride (2.0 mmol) was added dropwise to the above system. For the generation of corresponding acyl chloride, the mixture was allowed to stir for further 3 h. Subsequently, the reaction solvent was evaporated, dissolved by anhydrous DCM renewedly, and added dropwise to a solution of the intermediate 3 (2 mmol) and triethylamine (4 mmol) in anhydrous CH2Cl2 at 0 °C. After stirring for another 3 h at room temperature, the crude product was afforded by washed with water three times, dried, and concentrated in vacuo. The target compound was purified by column chromatography DCM/MeOH; the solvents were concentrated to give 13.
7-Acetoxy-4-oxo-4H-chromene-3-carboxylic acid (12)
Yield: 44.3%, yellow solid, m.p. = 202–204 °C; 1H NMR (400 MHz, DMSO-d6) δ 13.23 (s, 1H), 9.09 (s, 1H), 8.19 (d, J = 8.7 Hz, 1H), 7.69 (d, J = 2.0 Hz, 1H), 7.41 (dd, J = 8.7, 2.1 Hz, 1H), 2.34 (s, 3H); 13C NMR (100 MHz, DMSO-d6) δ 176.6, 165.5, 164.4, 164.2, 158.4, 127.3, 117.0, 116.8, 114.0, 101.7, 57.0.
3-((2–(3-((4-Methoxybenzyl)oxy)-2-methyl-4-oxopyridin-1(4H)-yl)ethyl)carbamoyl)-4-oxo-4H-chromen-7-yl acetate (13)
Yield: 23.6%, yellow oil, 1H NMR (400 MHz, CDCl3) δ 9.48 (t, J = 5.8 Hz, 1H), 8.91 (s, 1H), 8.28 (d, J = 8.8 Hz, 1H), 7.41 (d, J = 2.0 Hz, 1H), 7.32 (d, J = 8.6 Hz, 2H), 7.28 (d, J = 2.1 Hz, 1H), 7.23 (d, J = 7.5 Hz, 1H), 6.85 (d, J = 8.6 Hz, 2H), 6.44 (d, J = 7.5 Hz, 1H), 5.15 (s, 2H), 4.02 (t, J = 6.6 Hz, 2H), 3.78 (s, 3H), 3.61 (q, J = 6.4 Hz, 2H), 2.37 (s, 3H), 2.14 (s, 3H). 13C NMR (100 MHz, CDCl3) δ 176.4, 173.4, 165.0, 164.0, 162.0, 159.5, 158.0, 146.0, 141.3, 138.6, 130.9, 129.7, 127.6, 117.7, 117.3, 116.0, 114.9, 113.6, 100.5, 72.8, 56.1, 55.3, 52.3, 39.4, 12.6.
General procedure for the preparation of compounds 14a–g
Potassium carbonate (1.4 mmol) was added to the solution of intermediate 13 (1 mmol) in component solvent (acetone: water = 1: 1, 10 ml). After refluxing for 30 min, the ester group of intermediate 13 was hydrolysed. Then the mixture was evaporated and neutralised by adding 1 N HCl dropwise till no more solids precipitated. Filtered, washed with water to yield a faint yellow solid. Subsequently, appropriate benzyl bromide (1.2 eq) and K2CO3 (1.3 eq) were added to a solution of above intermediate (1 mmol) in DMF (4 ml). After stirring at 80 °C for another 12 h, the mixture was poured into water and extracted with dichloromethane three times, and washed with water. The collected organic layers were removed under vacuum to obtain yellow oil, which was purified by chromatography (DCM: MeOH = 30:1) to obtain the final compounds.
7-(Benzyloxy)-N-(2–(3-((4-methoxybenzyl)oxy)-2-methyl-4-oxopyridin-1(4H)-yl)ethyl)-4-oxo-4H-chromene-3-carboxamide (14a)
Yield: 25%, yellow oil, 1H NMR (400 MHz, CDCl3) δ 9.61 (t, J = 5.8 Hz, 1H), 8.83 (s, 1H), 8.15 (d, J = 9.0 Hz, 1H), 7.43 (t, J = 6.3 Hz, 5H), 7.32 (d, J = 8.5 Hz, 2H), 7.20 (d, J = 7.5 Hz, 1H), 7.13 (dd, J = 9.0, 2.2 Hz, 1H), 7.00 (d, J = 2.1 Hz, 1H), 6.84 (d, J = 8.5 Hz, 2H), 6.40 (d, J = 7.5 Hz, 1H), 5.18 (s, 2H), 5.14 (s, 2H), 3.99 (t, J = 6.6 Hz, 2H), 3.78 (s, 3H), 3.60 (q, J = 6.4 Hz, 2H), 2.14 (s, 3H); 13C NMR (100 MHz, CDCl3) δ 176.3, 173.4, 164.1, 164.0, 162.0, 159.5, 157.9, 146.0, 141.2, 138.5, 135.2, 130.9, 129.7, 128.9, 128.6, 127.7, 127.6, 117.9, 117.3, 116.5, 114.9, 113.6, 101.7, 72.8, 70.9, 55.3, 52.3, 39.4, 12.6; ESI-HRMS: m/z calcd for C33H30N2O7 [M + H]+: 567.2126; found: 567.2111.
N-(2–(3-((4-methoxybenzyl)oxy)-2-methyl-4-oxopyridin-1(4H)-yl)ethyl)-7-((3-methylbenzyl)oxy)-4-oxo-4H-chromene-3-carboxamide (14b)
Yield: 15%, yellow oil, 1H NMR (400 MHz, DMSO-d6) δ 9.34 (t, J = 6.0 Hz, 1H), 8.93 (s, 1H), 8.05 (d, J = 8.9 Hz, 1H), 7.51 (d, J = 7.5 Hz, 1H), 7.32 (d, J = 2.3 Hz, 1H), 7.28 (q, J = 7.3, 4.9 Hz, 5H), 7.21 (dd, J = 9.0, 2.3 Hz, 1H), 7.16 (d, J = 6.7 Hz, 1H), 6.87 (d, J = 8.6 Hz, 2H), 6.11 (d, J = 7.5 Hz, 1H), 5.22 (s, 2H), 4.92 (s, 2H), 4.05 (t, J = 6.3 Hz, 2H), 3.72 (s, 3H), 3.58 (q, J = 6.2 Hz, 2H), 2.32 (s, 3H), 2.19 (s, 3H). 13C NMR (100 MHz, DMSO-d6) δ 175.8, 172.5, 164.0, 163.1, 163.0, 159.4, 157.9, 145.7, 141.3, 140.0, 138.3, 136.2, 130.6, 130.2, 129.3, 129.0, 128.9, 127.4, 125.6, 117.7, 116.8, 116.5, 115.4, 114.0, 102.4, 72.0, 70.8, 55.5, 52.1, 39.2, 21.4, 12.5.
N-(2–(3-((4-methoxybenzyl)oxy)-2-methyl-4-oxopyridin-1(4H)-yl)ethyl)-7-((4-methylbenzyl)oxy)-4-oxo-4H-chromene-3-carboxamide (14c)
Yield: 16%, yellow oil, 1H NMR (400 MHz, DMSO-d6) δ 9.34 (t, J = 6.0 Hz, 1H), 8.93 (s, 1H), 8.05 (d, J = 8.9 Hz, 1H), 7.54 (d, J = 7.5 Hz, 1H), 7.37 (d, J = 7.9 Hz, 2H), 7.33 − 7.28 (m, 3H), 7.23 − 7.18 (m, 3H), 6.87 (d, J = 8.6 Hz, 2H), 6.15 (d, J = 7.5 Hz, 1H), 5.22 (s, 2H), 4.92 (s, 2H), 4.07 (t, J = 6.3 Hz, 2H), 3.72 (s, 3H), 3.58 (q, J = 6.1 Hz, 2H), 2.30 (s, 3H), 2.21 (s, 3H); 13C NMR (100 MHz, DMSO-d6) δ 175.8, 172.1, 164.0, 163.1, 163.0, 159.4, 157.9, 145.6, 141.8, 140.1, 138.0, 133.3, 130.6, 130.1, 129.6, 128.6, 127.4, 117.7, 116.9, 116.3, 115.4, 114.0, 102.4, 72.1, 70.7, 55.5, 52.3, 39.2, 21.3, 12.5.
7-((3-Fluorobenzyl)oxy)-N-(2–(3-((4-methoxybenzyl)oxy)-2-methyl-4-oxopyridin-1(4H)-yl)ethyl)-4-oxo-4H-chromene-3-carboxamide (14d)
Yield: 19%, yellow oil, 1H NMR (400 MHz, CDCl3) δ 9.61 (t, J = 5.9 Hz, 1H), 8.83 (s, 1H), 8.17 (d, J = 9.0 Hz, 1H), 7.42 − 7.28 (m, 4H), 7.21 (d, J = 7.7 Hz, 1H), 7.18 − 7.12 (m, 2H), 7.05 (dd, J = 8.5, 2.2 Hz, 1H), 6.99 (d, J = 2.3 Hz, 1H), 6.85 (d, J = 8.6 Hz, 2H), 6.58 (s, 1H), 5.18 (s, 2H), 5.16 (s, 2H), 4.05 (t, J = 6.4 Hz, 2H), 3.79 (s, 3H), 3.63 (q, J = 6.3 Hz, 2H), 2.18 (s, 3H); 13C NMR (100 MHz, CDCl3) δ 176.4, 173.4, 164.0, 163.8, 163.1 (d, 1J = 245 Hz), 162.1, 159.6, 158.0, 146.1, 141.2, 138.6, 137.9 (d, 3J = 7 Hz), 131.0, 130.6 (d, 3J = 9 Hz), 129.8, 127.9, 122.9 (d, 4J = 3 Hz), 118.2, 117.4, 116.4, 115.6 (d, 2J = 21 Hz), 115.1, 114.4 (d, 2J = 22 Hz), 113.7, 101.8, 72.8, 70.0, 55.4, 52.3, 39.5, 12.7.
7-((4-Fluorobenzyl)oxy)-N-(2–(3-((4-methoxybenzyl)oxy)-2-methyl-4-oxopyridin-1(4H)-yl)ethyl)-4-oxo-4H-chromene-3-carboxamide (14e)
Yield: 15%, yellow oil, 1H NMR (400 MHz, CDCl3) δ 9.59 (t, J = 5.9 Hz, 1H), 8.83 (s, 1H), 8.15 (d, J = 9.0 Hz, 1H), 7.41 (dd, J = 8.5, 5.3 Hz, 2H), 7.31 (d, J = 8.5 Hz, 2H), 7.22 (d, J = 7.5 Hz, 1H), 7.10 (td, J = 8.9, 2.2 Hz, 3H), 6.98 (d, J = 2.3 Hz, 1H), 6.84 (d, J = 8.6 Hz, 2H), 6.41 (d, J = 7.5 Hz, 1H), 5.13 (s, 4H), 4.00 (t, J = 6.6 Hz, 2H), 3.77 (s, 3H), 3.60 (q, J = 6.4 Hz, 2H), 2.14 (s, 3H); 13C NMR (100 MHz, CDCl3) δ 176.4, 173.4, 164.0, 163.9, 162.9 (d, 1J = 246 Hz), 162.1, 159.6, 158.0, 146.1, 141.2, 138.6, 131.1 (d, 4J = 3 Hz), 130.9, 129.8, 129.6 (d, 3J = 8 Hz), 127.8, 118.1, 117.4, 116.5, 115.9 (d, 2J = 21 Hz), 115.1, 113.7, 101.7, 72.8, 70.2, 55.4, 52.3, 39.5, 12.7.
7-((3-Chlorobenzyl)oxy)-N-(2–(3-((4-methoxybenzyl)oxy)-2-methyl-4-oxopyridin-1(4H)-yl)ethyl)-4-oxo-4H-chromene-3-carboxamide (14f)
Yield: 18%, yellow oil, 1H NMR (400 MHz, CDCl3) δ 9.62 (t, J = 5.8 Hz, 1H), 8.85 (s, 1H), 8.18 (d, J = 9.0 Hz, 1H), 7.45 (s, 1H), 7.37 − 7.31 (m, 5H), 7.21 (d, J = 7.5 Hz, 1H), 7.14 (dd, J = 9.0, 2.3 Hz, 1H), 6.99 (d, J = 2.3 Hz, 1H), 6.85 (d, J = 8.6 Hz, 2H), 6.41 (d, J = 7.4 Hz, 1H), 5.16 (s, 2H), 5.14 (s, 2H), 4.00 (t, J = 6.6 Hz, 2H), 3.79 (s, 3H), 3.60 (q, J = 6.4 Hz, 2H), 2.13 (s, 3H); 13C NMR (100 MHz, CDCl3) δ 176.3, 173.3, 164.0, 163.7, 162.1, 159.5, 157.9, 145.9, 141.5, 138.7, 137.3, 134.8, 130.8, 130.2, 129.6, 128.7, 127.8, 127.5, 125.4, 118.1, 117.1, 116.3, 115.0, 113.6, 101.7, 72.8, 69.9, 55.3, 52.4, 39.4, 12.6.
7-((4-Chlorobenzyl)oxy)-N-(2–(3-((4-methoxybenzyl)oxy)-2-methyl-4-oxopyridin-1(4H)-yl)ethyl)-4-oxo-4H-chromene-3-carboxamide (14g)
Yield: 16%, yellow oil, 1H NMR (600 MHz, DMSO-d6) δ 9.33 (t, J = 6.1 Hz, 1H), 8.95 (s, 1H), 8.08 (d, J = 8.9 Hz, 1H), 7.54 − 7.47 (m, 5H), 7.36 (d, J = 2.3 Hz, 1H), 7.30 (d, J = 8.5 Hz, 2H), 7.24 (dd, J = 8.9, 2.3 Hz, 1H), 6.88 (d, J = 8.5 Hz, 2H), 6.12 (d, J = 7.5 Hz, 1H), 5.30 (s, 2H), 4.92 (s, 2H), 4.06 (t, J = 6.4 Hz, 2H), 3.73 (s, 3H), 3.59 (q, J = 6.3 Hz, 2H), 2.20 (s, 3H). 13C NMR (150 MHz, DMSO-d6) δ 175.4, 172.0, 163.4, 162.6, 162.6, 159.0, 157.4, 145.2, 140.9, 139.5, 135.0, 132.9, 130.1, 129.8, 129.8, 128.6, 127.0, 117.4, 116.4, 116.0, 115.0, 113.5, 102.0, 71.6, 69.4, 55.0, 51.6, 40.1, 12.0.
General procedure for the preparation of compounds 16a–l
To a solution of compounds 5a–l (1 mmol) in anhydrous DCM (10 ml), Na(AcO)3BH (1.4 mmol) and intermediate 3 (1 mmol) were added under argon atmosphere at room temperature. After stirring for 6 h, the mixture was diluted with CH2Cl2 and washed with saturated NaHCO3 solution. The collected organic phases were dried over anhydrous sodium sulphate and removed in vacuo. The residue was purified by flash chromatography DCM/MeOH to afford intermediates 16a–l.
3-((4-Methoxybenzyl)oxy)-2-methyl-1–(2-(((4-oxo-4H-chromen-3-yl)methyl) amino)ethyl)pyridin-4(1H)-one (16a)
Yield: 30%, yellow oil, 1H NMR (400 MHz, CDCl3) δ 8.15 (dd, J = 8.0, 1.4 Hz, 1H), 7.82 (s, 1H), 7.67 − 7.61 (m, 1H), 7.43 − 7.35 (m, 2H), 7.28 (s, 2H), 7.25 (s, 1H), 6.78 (d, J = 8.5 Hz, 2H), 6.35 (d, J = 7.5 Hz, 1H), 5.09 (s, 2H), 3.83 (t, J = 6.3 Hz, 2H), 3.72 (s, 3H), 3.54 (s, 2H), 2.79 (t, J = 6.3 Hz, 2H), 2.05 (s, 3H). 13C NMR (100 MHz, CDCl3) δ 178.0, 173.4, 159.4, 156.5, 153.5, 145.8, 141.0, 138.9, 133.9, 130.8, 129.7, 125.6, 125.3, 123.7, 121.6, 118.3, 116.9, 113.6, 72.5, 55.2, 53.6, 48.6, 45.5, 12.6.
3-((4-Methoxybenzyl)oxy)-2-methyl-1–(2-(((7-methyl-4-oxo-4H-chromen-3-yl)methyl)amino)ethyl)pyridin-4(1H)-one (16b)
Yield: 27%, yellow oil, 1H NMR (400 MHz, CDCl3) δ 8.05 (dd, J = 8.4, 3.3 Hz, 1H), 7.79 (d, J = 9.1 Hz, 1H), 7.28 (d, J = 8.5 Hz, 2H), 7.22 (dt, J = 8.1, 4.9 Hz, 3H), 6.80 (d, J = 8.6 Hz, 2H), 6.37 (s, 1H), 5.11 (s, 2H), 3.84 (t, J = 6.3 Hz, 2H), 3.75 (s, 3H), 3.54 (s, 2H), 2.81 (t, J = 6.3 Hz, 2H), 2.46 (s, 3H), 2.06 (s, 3H); 13C NMR (100 MHz, CDCl3) δ 178.0, 173.5, 159.4, 156.7, 153.3, 145.8, 145.4, 141.1, 139.0, 130.8, 129.7, 126.9, 125.3, 121.5, 121.3, 117.9, 116.9, 113.6, 72.6, 55.2, 53.7, 48.6, 45.6, 21.9, 12.6.
3-((4-Methoxybenzyl)oxy)-2-methyl-1–(2-(((6-methyl-4-oxo-4H-chromen-3-yl)methyl)amino)ethyl)pyridin-4(1H)-one (16c)
Yield: 28%, yellow oil, 1H NMR (400 MHz, CDCl3) δ 7.94 (s, 1H), 7.82 (s, 1H), 7.46 (d, J = 7.9 Hz, 1H), 7.30 (d, J = 26.9 Hz, 4H), 6.79 (d, J = 6.6 Hz, 2H), 6.36 (d, J = 5.5 Hz, 1H), 5.11 (s, 2H), 3.85 (s, 2H), 3.74 (s, 3H), 3.55 (s, 2H), 2.81 (s, 2H), 2.43 (s, 3H), 2.06 (s, 3H); 13C NMR (100 MHz, CDCl3) δ 178.1, 173.5, 159.4, 154.8, 153.6, 145.8, 141.1, 139.0, 135.4, 135.3, 130.9, 129.6, 124.9, 123.4, 121.0, 118.0, 117.0, 113.5, 72.6, 55.2, 53.5, 48.5, 45.5, 21.0, 12.7.
1–(2-(((7-Methoxy-4-oxo-4H-chromen-3-yl)methyl)amino)ethyl)-3-((4-methoxybenzyl)oxy)-2-methylpyridin-4(1H)-one (16d)
Yield: 24%, yellow oil, 1H NMR (400 MHz, CDCl3) δ 8.07 (d, J = 8.9 Hz, 1H), 7.74 (d, J = 6.5 Hz, 1H), 7.29 (d, J = 8.5 Hz, 3H), 6.96 (dd, J = 8.9, 2.3 Hz, 1H), 6.80 (d, J = 8.7 Hz, 3H), 6.38 (d, J = 7.4 Hz, 1H), 5.12 (s, 2H), 3.88 (s, 3H), 3.86 − 3.82 (m, 2H), 3.75 (s, 3H), 3.53 (s, 2H), 2.80 (t, J = 6.3 Hz, 2H), 2.06 (s, 3H); 13C NMR (100 MHz, CDCl3) δ 177.3, 173.4, 164.2, 159.4, 158.3, 153.1, 145.8, 141.2, 139.1, 130.7, 129.6, 127.0, 121.3, 117.6, 116.9, 114.9, 113.6, 100.2, 72.6, 55.9, 55.2, 53.6, 48.5, 45.4, 12.6.
1–(2-(((6-Methoxy-4-oxo-4H-chromen-3-yl)methyl)amino)ethyl)-3-((4-methoxybenzyl)oxy)-2-methylpyridin-4(1H)-one (16e)
Yield: 25%, yellow oil, 1H NMR (400 MHz, CDCl3) δ 7.83 (s, 1H), 7.53 (d, J = 3.1 Hz, 1H), 7.40 (d, J = 9.2 Hz, 1H), 7.32 − 7.27 (m, 4H), 6.85 − 6.79 (m, 2H), 6.40 (d, J = 7.5 Hz, 1H), 5.14 (s, 2H), 3.89 (s, 3H), 3.86 (d, J = 6.4 Hz, 2H), 3.77 (s, 3H), 3.58 (s, 2H), 2.84 (t, J = 6.4 Hz, 2H), 2.08 (s, 3H); 13C NMR (100 MHz, CDCl3) δ 177.8, 173.5, 159.5, 157.0, 153.4, 151.4, 145.8, 141.1, 139.0, 130.8, 129.7, 124.3, 124.1, 120.6, 119.7, 116.9, 113.6, 104.6, 72.6, 55.9, 55.2, 53.6, 48.5, 45.5, 12.6.
1–(2-(((7-(Benzyloxy)-4-oxo-4H-chromen-3-yl)methyl)amino)ethyl)-3-((4-methoxybenzyl)oxy)-2-methylpyridin-4(1H)-one (16f)
Yield: 19%, yellow oil, 1H NMR (400 MHz, CDCl3) δ 8.09 (d, J = 8.9 Hz, 1H), 7.78 (s, 1H), 7.41 (q, J = 7.8 Hz, 5H), 7.30 (d, J = 8.6 Hz, 3H), 7.05 (dd, J = 8.9, 2.3 Hz, 1H), 6.90 (d, J = 2.3 Hz, 1H), 6.82 (d, J = 8.6 Hz, 2H), 6.40 (d, J = 7.4 Hz, 1H), 5.14 (s, 2H), 5.13 (s, 2H), 3.89 (t, J = 6.2 Hz, 2H), 3.76 (s, 3H), 3.57 (s, 2H), 2.85 (t, J = 6.2 Hz, 2H), 2.09 (s, 3H); 13C NMR (100 MHz, CDCl3) δ 177.3, 173.5, 163.3, 159.5, 158.2, 153.1, 145.9, 140.9, 138.9, 135.6, 130.8, 129.8, 128.8, 128.5, 127.6, 127.1, 121.2, 117.8, 117.0, 115.4, 113.6, 101.3, 72.6, 70.6, 55.2, 53.6, 48.5, 45.5, 12.7.
3-((4-Methoxybenzyl)oxy)-2-methyl-1–(2-(((7-((3-methylbenzyl)oxy)-4-oxo-4H-chromen-3-yl)methyl)amino)ethyl)pyridin-4(1H)-one (16g)
Yield: 15%, yellow oil, 1H NMR (400 MHz, CDCl3) δ 8.08 (d, J = 8.9 Hz, 1H), 7.73 (s, 1H), 7.28 (t, J = 7.3 Hz, 3H), 7.23 (dd, J = 11.8, 6.2 Hz, 3H), 7.16 (d, J = 7.3 Hz, 1H), 7.03 (dd, J = 8.9, 2.2 Hz, 1H), 6.88 (d, J = 2.1 Hz, 1H), 6.80 (d, J = 8.4 Hz, 2H), 6.38 (d, J = 7.5 Hz, 1H), 5.13 (s, 2H), 5.09 (s, 2H), 3.83 (t, J = 6.3 Hz, 2H), 3.75 (s, 3H), 3.52 (s, 2H), 2.80 (t, J = 6.3 Hz, 2H), 2.37 (s, 3H), 2.06 (s, 3H); 13C NMR (100 MHz, CDCl3) δ 177.3, 173.5, 163.3, 159.4, 158.2, 153.0, 145.8, 141.0, 139.0, 138.6, 135.5, 130.8, 129.7, 129.2, 128.7, 128.3, 127.0, 124.7, 121.3, 117.8, 117.0, 115.4, 113.6, 101.3, 72.6, 70.7, 55.2, 53.7, 48.6, 45.5, 21.5, 12.7.
3-((4-Methoxybenzyl)oxy)-2-methyl-1–(2-(((7-((4-methylbenzyl)oxy)-4-oxo-4H-chromen-3-yl)methyl)amino)ethyl)pyridin-4(1H)-one (16h)
Yield: 16%, yellow oil, 1H NMR (400 MHz, CDCl3) δ 8.08 (d, J = 8.9 Hz, 1H), 7.80 (s, 1H), 7.31 (t, J = 8.7 Hz, 5H), 7.21 (d, J = 7.8 Hz, 2H), 7.03 (dd, J = 8.9, 2.1 Hz, 1H), 6.89 (d, J = 2.0 Hz, 1H), 6.82 (d, J = 8.5 Hz, 2H), 6.40 (d, J = 7.4 Hz, 1H), 5.11 (d, J = 9.9 Hz, 4H), 3.90 (t, J = 6.0 Hz, 2H), 3.76 (s, 3H), 3.58 (s, 2H), 2.85 (t, J = 6.1 Hz, 2H), 2.36 (s, 3H), 2.08 (s, 3H); 13C NMR (100 MHz, CDCl3) δ 177.3, 173.4, 163.4, 159.5, 158.3, 145.8, 141.3, 139.0, 138.4, 132.4, 130.9, 129.6, 129.5, 127.8, 127.0, 117.7, 117.0, 115.5, 113.6, 101.3, 72.7, 70.6, 55.3, 53.4, 48.3, 45.3, 21.3, 12.7.
1–(2-(((7-((3-Fluorobenzyl)oxy)-4-oxo-4H-chromen-3-yl)methyl)amino)ethyl)-3-((4-methoxybenzyl)oxy)-2-methylpyridin-4(1H)-one (16i)
Yield: 16%, yellow oil, 1H NMR (400 MHz, CDCl3) δ 8.09 (d, J = 8.9 Hz, 1H), 7.74 (s, 1H), 7.39 − 7.27 (m, 4H), 7.19 (d, J = 7.7 Hz, 1H), 7.14 (d, J = 9.4 Hz, 1H), 7.05 − 7.00 (m, 2H), 6.88 − 6.85 (m, 1H), 6.80 (d, J = 8.3 Hz, 2H), 6.38 (d, J = 7.4 Hz, 1H), 5.12 (s, 4H), 3.85 (t, J = 6.1 Hz, 2H), 3.75 (s, 3H), 3.54 (s, 2H), 2.82 (t, J = 6.1 Hz, 2H), 2.07 (s, 3H). 13C NMR (100 MHz, CDCl3) δ 177.3, 173.4, 163.0 (d, 1J = 245 Hz), 162.9, 159.4, 158.2, 153.2, 145.9, 141.0, 139.0, 138.1 (d, 3J = 8 Hz), 130.8, 130.4 (d, 3J = 242 Hz), 129.7, 127.2, 122.8 (d, 4J = 2 Hz), 121.3, 118.0, 117.0, 115.4, 115.2, 114.3 (d, 2J = 22 Hz), 113.6, 101.4, 72.6, 69.7, 55.2, 53.6, 48.5, 45.4, 12.7.
1–(2-(((7-((4-Fluorobenzyl)oxy)-4-oxo-4H-chromen-3-yl)methyl)amino)ethyl)-3-((4-methoxybenzyl)oxy)-2-methylpyridin-4(1H)-one (16j)
Yield: 17%, yellow oil, 1H NMR (400 MHz, CDCl3) δ 8.10 (d, J = 8.9 Hz, 1H), 7.75 (s, 1H), 7.41 (dd, J = 8.5, 5.4 Hz, 2H), 7.34 − 7.27 (m, 3H), 7.12 − 7.07 (m, 2H), 7.04 (dd, J = 8.9, 2.4 Hz, 1H), 6.89 (d, J = 2.3 Hz, 1H), 6.82 (d, J = 8.6 Hz, 2H), 6.39 (d, J = 7.5 Hz, 1H), 5.15 (s, 2H), 5.10 (s, 2H), 3.86 (t, J = 6.3 Hz, 2H), 3.77 (s, 3H), 3.55 (s, 2H), 2.84 (t, J = 6.3 Hz, 2H), 2.09 (s, 3H). 13C NMR (100 MHz, CDCl3) δ 177.4, 173.5, 163.1, 162.8 (d, 1J = 245 Hz) 159.5, 158.3, 153.2, 145.9, 141.2, 139.1, 131.4 (d, 4J = 3 Hz), 130.9, 129.8, 129.6 (d, 3J = 9 Hz), 129.6 (d, 3J = 8 Hz), 127.2, 121.4, 118.0, 117.0, 115.8 (d, 2J = 21 Hz), 115.4, 113.7, 101.4, 72.7, 70.0, 55.3, 53.7, 48.6, 45.5, 12.7.
1–(2-(((7-((3-Chlorobenzyl)oxy)-4-oxo-4H-chromen-3-yl)methyl)amino)ethyl)-3-((4-methoxybenzyl)oxy)-2-methylpyridin-4(1H)-one (16k)
Yield: 15%, yellow oil, 1H NMR (400 MHz, CDCl3) δ 8.10 − 8.06 (m, 1H), 7.73 (s, 1H), 7.41 (s, 1H), 7.32 − 7.27 (m, 5H), 7.24 (s, 1H), 7.02 (dd, J = 8.9, 2.1 Hz, 1H), 6.85 (s, 1H), 6.79 (d, J = 8.6 Hz, 2H), 6.38 − 6.33 (m, 1H), 5.11 (s, 2H), 5.09 (s, 2H), 3.83 (t, J = 6.1 Hz, 2H), 3.75 − 3.72 (m, 3H), 3.52 (s, 2H), 2.80 (t, J = 6.0 Hz, 2H), 2.06 (s, 3H); 13C NMR (100 MHz, CDCl3) δ 177.2, 173.4, 162.8, 159.4, 158.2, 153.1, 145.9, 140.9, 139.0, 137.6, 134.7, 130.8, 130.1, 129.7, 128.6, 127.5, 127.2, 125.4, 121.4, 118.0, 116.9, 115.2, 113.6, 101.4, 72.5, 69.6, 55.2, 53.6, 48.6, 45.4, 12.6.
1–(2-(((7-((4-Chlorobenzyl)oxy)-4-oxo-4H-chromen-3-yl)methyl)amino)ethyl)-3-((4-methoxybenzyl)oxy)-2-methylpyridin-4(1H)-one (16l)
Yield: 16%, yellow oil, 1H NMR (400 MHz, CDCl3) δ 8.09 (d, J = 8.9 Hz, 1H), 7.73 (s, 1H), 7.37 (s, 4H), 7.31 − 7.27 (m, 3H), 7.03 (dd, J = 8.9, 2.2 Hz, 1H), 6.87 (d, J = 2.3 Hz, 1H), 6.81 (d, J = 8.5 Hz, 2H), 6.38 (d, J = 7.5 Hz, 1H), 5.12 (s, 2H), 5.10 (s, 2H), 3.84 (t, J = 6.3 Hz, 2H), 3.75 (s, 3H), 3.53 (s, 2H), 2.81 (t, J = 6.3 Hz, 2H), 2.07 (s, 3H); 13C NMR (100 MHz, CDCl3) δ 177.3, 173.5, 163.0, 159.5, 158.2, 153.0, 145.9, 141.0, 139.0, 134.3, 134.1, 130.8, 129.7, 129.0, 128.9, 127.2, 121.4, 118.0, 116.9, 115.2, 113.6, 101.3, 72.6, 69.8, 55.2, 53.7, 48.6, 45.5, 12.7.
Determination of pKa and Fe3+ affinity by spectrophotometry method
The experimental procedures of the pKa and Fe3+ affinity assay were performed according to our reported methodsCitation20. For a detailed experiment protocol, please refer to the supporting information.
Monoamine oxidase enzyme assay and kinetic studies
The reagents and enzymes (Human MAO-B and Human MAO-A) applied in this experiment were purchased from Sigma-Aldrich. According to the manuals, all components (substrate solution and enzyme solution) are diluted and placed aside for use. The detailed process has been mentioned in our early workCitation34.
To further examine the interaction mode of compound 17d, the type of enzyme inhibition was determined by Michealis–Menten kinetic experiments. The catalytic rates of MAO‐B enzyme were measured at six different concentrations of substrate p‐tyramine (0.05, 0.1, 0.25, 0.5, 1.0, and 1.25 mM) in the absence and in the three different concentrations (33.3, 100, and 300 nM) of compound 17d. The corresponding dose‐response curves and the nonlinear/linear regression analysis were performed using GraphPad Prism version 6 software (Graphpad Software Inc., La Jolla, CA).
PAMPA-BBB assay
PAMPA was used to predict the BBB permeability of the screened compound. The detailed process has been mentioned in our early workCitation32,Citation33.
Cytotoxicity test
Neural pheochromocytoma-derived PC-12 cells at the log phase of their growth cycle (8 × 104 cells/ml) were added to each well (100 μl/well) and incubated for 24 h at 37 °C in a humidified atmosphere of 5% CO2. Then three replicates at various concentrations of the samples (3.13, 6.25, 12.5, 25, and 50 μM) were added to each well (100 μl/well). After incubating for 24 h, 20 μl of MTT solution (5 mg/ml/well) was added to each culture medium, which was then incubated for another 4 h. Then DMSO was added to each well (150 μl/well). After 10 min at room temperature, the OD values of each well were measured on a Microplate Reader (BioTek ELx800, Winooski, VT) at the wavelength of 490 nm.
Molecular modelling studies
According to our previous methodsCitation20, docking simulation was detailed in the supporting information, using crystal structures of MAO-B in complex with safinamide (PDB code 2V5Z)Citation35 as the template. For a detailed experiment protocol, please refer to the supporting information.
Morris water maze test
Adult female ICR mice (weight 20–25 g) were obtained from the Zhejiang Academy of Medical Sciences (Hangzhou, China). Scopolamine hydrobromide and Pargyline were purchased from Aladdin Reagents. Memantine was purchased from Energy Chemical. All experiments were approved by the Laboratory Animals Ethical Committee of the Zhejiang University of Technology (No. 20211210103). In this test, mice were prepared as clarified and clear injections, consisting of 20% (2-Hydroxypropyl)-β-cyclodextrin, 10% DMSO, and PBS. The mice were randomly quartered into five groups: (i) control group (PBS of 20% (2-Hydroxypropyl)-β-cyclodextrin), (ii) scopolamine hydrobromide model group (15 mg/kg), (iii) pargyline group (15 mg/kg) + 15 mg/kg scopolamine hydrobromide, (iv) memantine group (15 mg/kg) + 15 mg/kg scopolamine hydrobromide, and (v) compound 17d group (15 mg/kg) + 15 mg/kg scopolamine hydrobromide. Mice were administered at a frequency of once a day over 15 d with a fixed dosing schedule. The body weight of mice was measured during the experiment.
After eliminating environmental stress, the behavioural test was performed over the next five days. The mice were trained to find the platform (10 cm diameter) in the circular light-avoidance pool (120 cm diameter and 60 cm height) filled with water (40 cm, depth), which was described in detail in our previous studyCitation34. All mice received at least one training session daily in four quadrants for four consecutive days before the probe trial of finding the platform within 120 s. Recordings were made with SMART version 3.0 (Panlab, Spain) and processed by Graphpad Prism version 6 software (Graphpad Software Inc., La Jolla, CA, USA).
Pharmacokinetic study
Rats were purchased from the Zhejiang Academy of Medical Sciences (Hangzhou, China). All rats were kept strictly following the requirements of experimental animal operation instructions, kept at a temperature (22 ± 2 °C), day/night cycle every 12 h in a constant environment. 12 h before the experiment, the rats were fasted but free to drink. For each rat, retro-orbital blood samples (0.2 ml) were obtained into EDTA soaked polythene tubes before drug administration and at time points of 0.083, 0.25, 0.50, 0.75, 1.0, 2.0, 4.0, 6.0, 8.0, 10, 12, and 24 h post-dosing. The collected blood samples were immediately centrifuged at 3500 rpm for 10 min at room temperature to separate plasma, and stored in a −80 °C refrigerator before use. For brain tissue distribution investigation, rats were sacrificed at Cmax time point after i.g. administration (15 mg/kg) of 17d. The tissue was weighed and homogenised under ice bath conditions and was collected for further processing.
Biological samples were performed with ACQUITY UPLC® H Class system, which was coupled to an Xevo TQ-S micro triple quadrupole mass spectrometer (Waters, Milford, MA, USA) with an ESI source and MassLynxTM Workstation software version 4.2 (Waters, Milford, MA, USA). We used a BEH Shield RP C18 column (100 × 2.1 mm, 1.7 µm, Waters, Milford, MA, USA) for chromatographic separation, and the column temperature is kept at 30 °C. The mobile phase was composed of 30%, 0.2% formic acid aqueous solution (A) and 70%, 0.3% formic acid methanol solution (B) and is delivered at a flow rate of 0.2 ml/min. The temperature of the autosampler is set to 30 °C. The sample injection volume is 5 µl. Pharmacokinetic parameters of the analytes were calculated using the pharmacokinetic software DAS version 2.0 (Bontz Inc., Beijing, China). All data were expressed as mean ± SD.
Disclosure statement
No potential conflict of interest was reported by the author(s).
Additional information
Funding
References
- Alzheimer's Association. Alzheimer’s disease facts and figures. Alzheimer’s Dement. 2021;17:327–406.
- Srivastava S, Ahmad R, Khare SK. Alzheimer’s disease and its treatment by different approaches: a review. Eur J Med Chem. 2021;216:113320.
- Abeysinghe A, Deshapriya R, Udawatte C. Alzheimer’s disease; a review of the pathophysiological basis and therapeutic interventions. Life Sci. 2020;256:117996.
- Kepp KP. Bioinorganic chemistry of Alzheimer’s disease. Chem Rev. 2012;112(10):5193–5239.
- Telling ND, Everett J, Collingwood JF, Dobson J, van der Laan G, Gallagher JJ, Wang J, Hitchcock AP. Iron biochemistry is correlated with amyloid plaque morphology in an established mouse model of Alzheimer’s disease. Cell Chem Biol. 2017;24(10):1205–1215 e3.
- Savelieff MG, Nam G, Kang J, Lee HJ, Lee M, Lim MH. Development of multifunctional molecules as potential therapeutic candidates for Alzheimer’s disease, Parkinson’s disease, and amyotrophic lateral sclerosis in the last decade. Chem Rev. 2019;119(2):1221–1322.
- Bai R, Guo J, Ye XY, Xie Y, Xie T. Oxidative stress: the core pathogenesis and mechanism of Alzheimer’s disease. Ageing Res Rev. 2022;77:101619.
- Lane DJR, Ayton S, Bush AI. Iron and Alzheimer’s disease: an update on emerging mechanisms. J Alzheimers Dis. 2018;64(s1):S379–S395.
- Mesiti F, Chavarria D, Gaspar A, Alcaro S, Borges F. The chemistry toolbox of multitarget-directed ligands for Alzheimer’s disease. Eur J Med Chem. 2019;181:111572.
- Jiang X, Zhou T, Bai R, Xie Y. Hydroxypyridinone-based iron chelators with broad-ranging biological activities. J Med Chem. 2020;63(23):14470–14501.
- Cong L, Dong X, Wang Y, Deng Y, Li B, Dai R. On the role of synthesized hydroxylated chalcones as dual functional amyloid-beta aggregation and ferroptosis inhibitors for potential treatment of Alzheimer’s disease. Eur J Med Chem. 2019;166:11–21.
- Tripathi AC, Upadhyay S, Paliwal S, Saraf SK. Privileged scaffolds as MAO inhibitors: retrospect and prospects. Eur J Med Chem. 2018;145:445–497.
- Manzoor S, Hoda N. A comprehensive review of monoamine oxidase inhibitors as anti-Alzheimer’s disease agents: a review. Eur J Med Chem. 2020;206:112787.
- Zhou J, Jiang X, He S, Jiang H, Feng F, Liu W, Qu W, Sun H. Rational design of multitarget-directed ligands: strategies and emerging paradigms. J Med Chem. 2019;62(20):8881–8914.
- Wang T, Liu XH, Guan J, Ge S, Wu MB, Lin JP, Yang LR. Advancement of multi-target drug discoveries and promising applications in the field of Alzheimer’s disease. Eur J Med Chem. 2019;169:200–223.
- Carradori S, Silvestri R. New frontiers in selective human MAO-B inhibitors. J Med Chem. 2015;58(17):6717–6732.
- Liu W, Lang M, Youdim MBH, Amit T, Sun Y, Zhang Z, Wang Y, Weinreb O. Design, synthesis and evaluation of novel dual monoamine-cholinesterase inhibitors as potential treatment for Alzheimer’s disease. Neuropharmacology. 2016;109:376–385.
- Mechlovich D, Amit T, Mandel SA, Bar-Am O, Bloch K, Vardi P, Youdim MBH. The novel multifunctional, iron-chelating drugs M30 and HLA20 protect pancreatic beta-cell lines from oxidative stress damage. J Pharmacol Exp Ther. 2010;333(3):874–882.
- Esteban G, Allan J, Samadi A, Mattevi A, Unzeta M, Marco-Contelles J, Binda C, Ramsay RR. Kinetic and structural analysis of the irreversible inhibition of human monoamine oxidases by ASS234, a multi-target compound designed for use in Alzheimer’s disease. Biochim Biophys Acta. 2014;1844(6):1104–1110.
- Zhang C, Yang K, Yu S, Su J, Yuan S, Han J, Chen Y, Gu J, Zhou T, Bai R, et al. Design, synthesis and biological evaluation of hydroxypyridinone-coumarin hybrids as multimodal monoamine oxidase B inhibitors and iron chelates against Alzheimer’s disease. Eur J Med Chem. 2019; 180:367–382.
- Pachón-Angona I, Refouvelet B, Andrýs R, Martin H, Luzet V, Iriepa I, Moraleda I, Diez-Iriepa D, Oset-Gasque M-J, Marco-Contelles J, et al. Donepezil + chromone + melatonin hybrids as promising agents for Alzheimer’s disease therapy. J Enzyme Inhib Med Chem. 2019;34(1):479–489.
- Jiang X, Guo J, Zhang C, Gu J, Zhou T, Bai R, Xie Y. Discovery of benzamide-hydroxypyridinone hybrids as potent multi-targeting agents for the treatment of Alzheimer’s disease. J Enzyme Inhib Med Chem. 2021;36(1):2045–2054.
- Reis J, Cagide F, Chavarria D, Silva T, Fernandes C, Gaspar A, Uriarte E, Remião F, Alcaro S, Ortuso F, et al. Discovery of new chemical entities for old targets: insights on the lead optimization of chromone-based monoamine oxidase B (MAO-B) inhibitors. J Med Chem. 2016;59(12):5879–5893.
- Fonseca A, Reis J, Silva T, Matos MJ, Bagetta D, Ortuso F, Alcaro S, Uriarte E, Borges F. Coumarin versus chromone monoamine oxidase B inhibitors: quo vadis? J Med Chem. 2017;60(16):7206–7212.
- Reis J, Gaspar A, Milhazes N, Borges F. Chromone as a privileged scaffold in drug discovery: recent advances. J Med Chem. 2017;60(19):7941–7957.
- Mi Z, Gan B, Yu S, Guo J, Zhang C, Jiang X, Zhou T, Su J, Bai R, Xie Y. Dual-target anti-Alzheimer’s disease agents with both iron ion chelating and monoamine oxidase-B inhibitory activity. J Enzyme Inhib Med Chem. 2019;34(1):1489–1497.
- Rullo M, Catto M, Carrieri A, de Candia M, Altomare CD, Pisani L. Chasing ChEs-MAO B multi-targeting 4-aminomethyl-7-benzyloxy-2-chromen-2-ones. Molecules. 2019;24(24):4507.
- Zeng H, Wu X. Alzheimer’s disease drug development based on computer-aided drug design. Eur J Med Chem. 2016; 121:851–863.
- Gaspar A, Silva T, Yanez M, Vina D, Orallo F, Ortuso F, Uriarte E, Alcaro S, Borges F. Chromone, a privileged scaffold for the development of monoamine oxidase inhibitors. J Med Chem. 2011;54(14):5165–5173.]
- Masaldan S, Bush AI, Devos D, Rolland AS, Moreau C. Striking while the iron is hot: iron metabolism and ferroptosis in neurodegeneration. Free Radic Biol Med. 2019;133:221–233.
- Xie Y-Y, Lu Z, Kong X-L, Zhou T, Bansal S, Hider R. Systematic comparison of the mono-, dimethyl- and trimethyl 3-hydroxy-4(1H)-pyridones – attempted optimization of the orally active iron chelator, deferiprone. Eur J Med Chem. 2016;115:132–140.
- Guo J, Zhang Y, Zhang C, Yao C, Zhang J, Jiang X, Zhong Z, Ge J, Zhou T, Bai R, et al. N-propargylamine-hydroxypyridinone hybrids as multitarget agents for the treatment of Alzheimer’s disease. Bioorg Chem. 2021;113:105013.
- Di L, Kerns EH, Fan K, McConnell OJ, Carter GT. High throughput artificial membrane permeability assay for blood–brain barrier. Eur J Med Chem. 2003;38(3):223–232.
- Guo J, Mi Z, Jiang X, Zhang C, Guo Z, Li L, Gu J, Zhou T, Bai R, Xie Y. Design, synthesis and biological evaluation of potential anti-AD hybrids with monoamine oxidase B inhibitory and iron-chelating effects. Bioorg Chem. 2021;108:104564.
- Binda C, Wang J, Pisani L, Caccia C, Carotti A, Salvati P, Edmondson DE, Mattevi A. Structures of human monoamine oxidase B complexes with selective noncovalent inhibitors: safinamide and coumarin analogs. J Med Chem. 2007;50(23):5848–5852.