Abstract
We evaluated the effects of dentine biomodification after pre-treatment with two sulphonamide carbonic anhydrase inhibitors (CAIs) of the N-[4-sulphamoylphenethylcarbamoyl]benzenesulphonamide type, investigating matrix metalloproteases activity, resin–dentine micro tensile bond strength, dentine surface wettability, and antimicrobial activities. Ninety-five sound-extracted human molars were selected for the study. Inhibitory effects were evaluated by gelatinase and collagenase activity tests and collagen degradation FT-IR spectroscopic analysis. Pre-treatment with the two CAIs kept the micro tensile values after 12 months of storage (32.23 ± 5.95) and cariogenic challenge (34.13 ± 2.71) similar to the initial, pre-treatment values (33.56 ± 4.34). A decreased Streptococcus mutans biofilm formation on dentine surfaces and antibacterial activity against planktonic bacteria were observed after CAI treatment. Dentine pre-treatment with sulphonamide CAIs maintained adhesion strength stability, allowed better dentine wettability, maintained matrix collagen, and showed anti-S. mutans activity.
Introduction
Despite the constant and visible advances regarding the physicochemical characteristics of resin composites and their adhesion to dental tissues, it is still possible to observe failures in the resin–dentine bonding interface over timeCitation1,Citation2. For the Effectiveness of adhesive procedures, dentine’s structure, composition, and characteristics are decisive since adhesion to the dentine substrate is more complex and challenging than to the enamelCitation3. In general, dentine is a mineralised tissue based on hydroxyapatite and collagen embedded in an extracellular matrix. Approximately 10% of this matrix comprises proteins, including proteoglycans and enzymesCitation4.
Among these enzymes, the matrix metalloproteinases (MMPs) are involved in collagen degradation in the hybrid layer and the progression of dentinal cariesCitation5,Citation6. The collagen degradation occurs due to the incomplete impregnation of resin monomers in demineralised dentine by phosphoric acid conditioning or acidic monomers present in adhesive systems, creating discrepancies between demineralisation depth and monomeric infiltration, leading the collagen fibres unprotected and activating the MMPsCitation2–4. In the progression of dental caries, the acidic environment created by the cariogenic biofilm generates the dissolution of the inorganic content of dental tissues exposing the organic portion constituted by collagen fibres. The action of the MMPs degrades these unprotected fibres activated due to the low pHCitation7–9.
Studies have been developed to identify substances capable of inhibiting the activity of MMPs. Inhibitors such as chlorhexidine and galardinCitation3,Citation4,Citation10–14 can improve the integrity and stability of the dentine–resin bond when used as a dentine pre-treatment. However, the results of the usage of these inhibitors in dentine quality are still controversial. Among these inhibitors, chlorhexidine is the most frequently investigated one, being able to reduce the degradation at the dentine–resin interfaceCitation11,Citation15. Nevertheless, this inhibitor is susceptible to long-term leaching, interrupting the inactivation of the MMPs, promoting the degradation of collagen fibrils exposed at the adhesive interface, and decreasing the resin–dentine bond strengthCitation16.
In addition to the presence of MMPs in dentine, these enzymes are also found in other parts of the human body and other organisms, being involved in a broad spectrum of important biological reactions, including protein metabolism, immune reactions, tissue remodelling, and blood clottingCitation17. Thus, the cellular proteolytic activity must be highly regulated to prevent inappropriate and uncontrolled degradation of proteins. As a result, several studies have been conducted to discover alternative inhibitors of these MMPsCitation18–23.
Sulphonamides and their derivatives are efficient inhibitors of carbonic anhydrases (CAs), metalloenzymes that catalyse the reaction between CO2 and water, generating H+ and HCO3−Citation24–28. Nonetheless, to our knowledge, no reports show these compounds act as inhibitors of enzymes/processes that improve dentine adhesion. Thus, this present study aimed to evaluate the protective effects of sulphonamide CA inhibitors (CAIs) on dentine. The tested hypotheses were: (1) the pre-treatment of the dentine surface with sulphonamide derivatives will not decrease the micro tensile strength after ageing in distilled water for 12 months and after cariogenic challenge, and it will not influence dentine wettability as well; (2) the sulphonamide derivatives will be capable of inhibiting the proteolytic activity of the dentine matrix enzymes with the preservation of dentine collagen; and (3) the sulphonamide derivatives will present antimicrobial and antibiofilm activity against Streptococcus mutans, one of the bacterial species present in the oral cavity, probably due to inhibition of the β-class CA present in this pathogenCitation25.
Materials and methods
Study design and materials
The present study was approved by the Ethics and Research Committee of the Hospital Universitário Antônio Pedro (Universidade Federal Fluminense, RJ, Brasil) under the number 3.383.557.
describes the composition and manufacturers of all commercial materials used in the present study.
Table 1. Composition and manufacture of commercial materials used at the study.
Chemicals
The synthesis of sulphonamide derivatives was previously describedCitation29,Citation30 and consisted in reacting aminosulphonamides with aryl sulphonyl isocyanates, originating in a series of aryl sulphonyl-ureido aromatic/heterocyclic sulphonamides, two of which, namely, N-[4-sulphamoylphenethylcarbamoyl] benzenesulphonamide (SULFA1) and 4-methyl-N-[4-sulphamoylphenethylcarbamoyl] benzenesulphonamide (SULFA2) have been employed in the work (see for structural details). SULFA1 and SULFA2 were solubilised in dimethyl sulphoxide (DMSO) (Sigma-Aldrich®, St. Louis, MO, USA) and then diluted in distilled water until reaching concentrations of 100, 150, and 200 µM. 1,10-phenanthroline (Sigma-Aldrich®, St. Louis, MO, USA) at 100 µM was used as a standard metalloprotease inhibitor.
Gelatinolytic and collagenolytic activity
The concentration of SULFA1 and SULFA2 derivatives used in the present study was previously defined through an experiment that aimed to verify the concentration with the best inhibitory proteolytic activity of the MMPsCitation31.
The gelatinolytic and collagenolytic activity of SULFA1 and SULFA2 were assessed as described by Li et alCitation32. Human molars teeth (n-5) without dental caries extracted due to dental need were cleaned with periodontal curettes, stored for seven days in 0.5% chloramine aqueous solution for disinfection, and kept in distilled water under refrigeration before use. The occlusal enamel was removed in a metallographic cutter (ISOMET 1000, Buehler Ltd, IL, USA). The marginal enamel was removed with a diamond tip (4138, KG Sorensen, Curitiba, PR, BR) assembled on a high-speed turbine under refrigeration. Following this step, teeth were sectioned at the level of the cement-enamel junction, and enamel and pulp tissues were removed. The dentine blocks were frozen in liquid nitrogen to be crushed in a ball mill (Model MM400, Retsch GmbH, Haan, DE) at 30 Hz/7 min. The dentine powders were then demineralised with 37% phosphoric acid for 15 s and rinsed with distilled water. After drying, three different concentrations of the compounds were added: 100 μM, 150 μM, and 200 μM. Eighty milligrams of dentine powders and 20 µl of 100 µg/mL gelatin/collagen (EnzChek-E-12055, Molecular Probes, Eugene, OR, USA) were added to 96-well plates and incubated at 37 °C for 1 h. Fluorescent cleavage products, derived from the proteolytic activity, were measured using a microplate reader (FLUOstar Optima®, BMG Labtech, Ortenberg, GER) with maximum absorption of 495 nm and maximum fluorescence emission of 515 nm. Each well’s relative fluorescence unit (RFU) was corrected by subtracting the background fluorescence value of the non-enzyme control; 2% DMSO (Sigma-Aldrich®, St. Louis, MO, USA) was used as control.
Assessment of dentine adhesion
Specimens preparation
This assay used 50 donated human molars with no cavities and extracted due to dental need. The teeth were cleaned with periodontal curettes, stored for seven days in 0.5% chloramine aqueous solution for disinfection, and kept in distilled water under refrigeration until their use. The teeth were included by the roots with acrylic resin within the PVC rings. After polymerisation of the acrylic resin, the occlusal enamel was removed in a metallographic cutter (ISOMET 1000, Buehler Ltd, IL, USA), exposing the superficial layer of dentine. Then, the marginal enamel was removed with a diamond tip (4138, KG Sorensen, Curitiba, PR, BR) assembled on a high-speed turbine under refrigeration. The dentine smear layer was standardised with 150, 400, and 600-grit SIC papers in a polishing machine (DPU 10, Struers, DEN) for 1 min. After the preparation of the dentine surfaces, the teeth were randomly divided into the following experimental groups (n = 5): SULFA1 − 37% Phosphoric Acid + 200 µM N-(4-sulphamoyl phenethyl carbamoyl) benzenesulphonamide + Adper Single Bond 2; SULFA2 − 37% Phosphoric Acid + 200 µM 4-Methyl-N-(4-sulphamoyl phenethyl carbamoyl) + Adper Single Bond 2; DMSO − 37% Phosphoric Acid + 2% dimethyl sulphoxide + Adper Single Bond 2; PHEN − 37% Phosphoric Acid + 1,10-phenanthroline + Adper Single Bond 2; CTR 37% Phosphoric Acid + Adper Single Bond 2.
The etching of the dentine with 37% phosphoric acid occurred for 15 s, followed by washing with water for 30 s and drying with absorbent paper. Dentine hybridisation with Adper Single Bond 2 adhesive followed the manufacturer’s recommendations. Subsequently, Filtek Z250 composite blocks with 5.0 mm high were constructed incrementally on the dentine surface. Each increment was photoactivated with an irradiance of 1000 mW/cm2 for the 20 s (DEMI, Demetron Inc., Danbury, USA). After the composite block was made, the teeth were stored in distilled water at 37 °C for 24 h. Thus, the teeth were taken to the metallographic cutter (IsoMet 1000, Buëhler, Lake Bluff, IL, USA) and serially sectioned with a double-sided diamond disc under refrigeration in two planes perpendicular to the adhesive interface, obtaining beams with approximately 1.0 mm2 cross-section. After the cut, all beams were evaluated in a stereomicroscope (SZ40, Olympus, Tokyo, JPN), with a 40× magnification, to discard those with structural defects (cracks or blisters in the composite).
The beams obtained from each tooth for each group were randomly divided into three subgroups: (1) 24 h of immersion in distilled water (SULFA1 24H, SULFA2 24H, DMSO 24H, CTR 24H E PHEN 24H); (2) 12 months of immersion in distilled water (SULFA1 12 M, SULFA2 12 M, DMSO 12 M, CTR 12 M E PHEN 12 M) at 37 °C. The distilled water was replaced weekly for fresh amounts; (3) submitted to S. mutans biofilm for 48 h at the cariogenic challenge (CC) (SULFA1 CC, SULFA2 CC, DMSO CC, CTR CC E PHEN CC).
The beams submitted to the cariogenic biofilm were painted with nail varnish (Colorama-CIEIL-SP, BR), leaving 1.0 mm2 free around the bonded interfaces. The specimens were then fixed in 24-well plates (TPP, 24 Zellkultur Festplatte F, GER). The cariogenic challenge was performed according to Carvalho et alCitation33. Afterwards, the beams were exposed to ultraviolet light for disinfectionCitation34 and subjected to bacterial suspension of S. mutans (ATCC 25175), prepared as follows: the S. mutans strain was cultured in Mitis Salivarius agar (Difco, Sparks, USA) at 37 °C under low oxygen tension conditions. Subsequently, single colonies were inoculated into 5 ml of Brain Heart Infusion (BHI) broth (Difco, Sparks, USA) supplemented with 0.5% glucose and 1.0% sucrose and incubated at 37 °C for 24 h. The bacterial suspension was adjusted to 2 × 108 CFU/ml. During the experiment, the growth environment was changed every 24 h. The beams remained for three days at 37 °C under low oxygen conditions.
Microtensile bond strength (µTBS)
The cross-sectional area of the beam was measured with a digital calliper (MPI/E-101, Mitutoyo, Tokyo, JAP). Then, they were fixed to a micro tensile device (ODMT03d, Odeme Biotechnology, Santa Catarina, BRA) using cyanoacrylate glue (Super bonder Gel, 3 M, São Paulo, BRA) and loaded under tension in a universal testing machine (EMIC DL 2000, São José dos Pinhais, São Paulo, BRA) at a crosshead speed of 1.0 mm/min until failure occurred. The µTBS (MPa) was obtained by dividing the load at failure (N) by the cross-sectional area (mm2). An average was calculated with the bond strength values presented by the beams obtained for each tooth so that the tooth was considered the experimental unit for the statistical test.
Failure mode
The fractured surfaces were evaluated under a stereomicroscope at 40x magnification (SZ40, Olympus, Tokyo, Japan). The mode of failure was classified as an adhesive (failures at the adhesive interface), cohesive (failures occurring within dentine or resin composite), or mixed (mixture of adhesive and cohesive failure within the same fractured surface).
Contact angle measurements
To evaluate the contact angle, 25 dentine discs 1.0 mm thick (from 17 human molars) were made following the methodology described by Ricci et alCitation35. The specimens were etched with 37% phosphoric acid for 15 s, rinsed for 30 s with distilled water, and the blot was dried with absorbent paper. The specimens were randomly divided into groups according to dentine surface treatment (n = 5): SULFA1 – 200 µM, SULFA2 – 200 µM; DMSO – 2%; PHEN – 100 µM; CTR – without treatment. The contact angles of the specimens were measured using a contact angle goniometer (Model 200, Ramé-hart Instrument Company, Netcong, USA). On the dentine surface, 2.0 µl of the Adper Single Bond 2 adhesive system (3 M ESPE, St. Paul, MN, USA) was applied with a pre-adjusted 1.0 ml syringe on the dentine surface, followed by the measurement of the contact angle between the dentine surface and the liquid drop. Thirty measurements with an interval of 1 s were performed with the goniometer. Based on the data obtained for the liquid (DA), the Ramé-Hart software calculated the contact angle of the determined groups.
Collagen degradation spectroscopic analysis
After wet grinding the coronal enamel (180-grit SiC paper) of three sound human molars until exposing flat dentine and removal of peripheral enamel, a 1.0 mm thick underlying dentine slice was obtained using a diamond saw in a cutting machine (ISOMET 1000, Lake Bluff, IL, USA) under water cooling. Then, the dentine slices were ground (600-grit Sic paper) until 0.5 mm thick dentine slices were obtained and monitored with a digital calliper (MPI/E −101, Mytutoyo, Tokyo, Japan). Each slice was immersed in 3 ml of 10% phosphoric acid and maintained for 40 h on a magnetic stirrer (Q261A11, Quimes, Rio de Janeiro, RJ, Brasil) until demineralisation and collagen loss. After washing for 10 min in distilled water, each demineralised dentine slice was cut with a scalpel blade to obtain collagen fragments (2 × 2 mm), which were assigned into five groups (SULFA1, SULFA2, DMSO, PHEN, and CTR). After immersion into groups for 1 min, the collagen fragments were gently dried with absorbent paper and stored in a collagenase solution from Clostridium histolyticum (Type I, 125 CDU/mg, Sigma-Aldrich®, St Louis, USA) (100 µg/ml) for 15 days at 37 °C, which was renewed daily.
The collagenase solution was prepared and renewed every 24 h from the solubilisation of 100 µg of collagenase from C. histolyticum (Type I, 125 CDU/mg, Sigma-Aldrich®, St Louis, USA) in 1 ml of buffer solution TESCA (5,72 g of acid N-Tris (hydroxymethyl) methyl-2-aminoethanesulphonic (Sigma-Aldrich®, St Louis, USA) and 20 mg of calcium chloride (Dinâmica, Indaiatuba, SP, BRA) dissolved in 500 ml of distilled water. All components were weighed on an analytical balance (XP 205, METTLER TOLEDO, Greinfensee, CHE) and then mixed manually.
The collagen was analysed according to Kishen et al.Citation36 method, using an ATR crystal of an FT-IR (Alpha-P/Platinum ATR Module, Bruker Optics GmbH, Ettlingen, GER). The spectra were recorded between 1200 and 1800 cm−1 using 80 scans at a 4.0 cm−1 resolution after two storage periods: immediate (after application of the inhibitor for 1 h) and 15 days of storage. The infra-red bands considered for this study were 1648 cm−1 (amide I) and 1563 cm−1 (amide II).
Antibacterial activity of CA inhibitors
For this assay, 20 sound molars were used to make 20 standardised dentine discs (5.0 × 5.0 mm and 1.0 mm thick). Discs were exposed to ultraviolet light for disinfectionCitation34, and the substances tested were previously sterilised by filtration. The discs were randomly divided into SULFA1, SULFA2, DMSO, PHEN, and CTR groups (n = 4). Then, 50 µl of the protease inhibitor solutions were applied to the dentine surface with a sterile micro applicator for 60 s, and excesses were removed with sterile absorbent paper. The pre-treated dentine discs were placed in 24-well plates, with the treatment surface facing up. After the growth of S. mutans in BHI for 48 h at 37 °C under low oxygen tension, the bacterial suspension was adjusted for an optical density of 0.5 (550 nm) according to the McFarland scale. Then, the suspension was diluted (1:100), 10.0 µl was diluted in 2 ml of BHI supplemented with 2% sucrose and added to each well with dentine discs. The 24-well culture plates were incubated at 37 °C under low oxygen tension for 48 h. Over the two days of biofilm formation, the culture environment was replaced every 24 hCitation37.
The cell viability of the S. mutans biofilms on the adhesive discs was analysed by tetrazolium azide (MTT; Sigma-Aldrich®, St. Louis, MO, USA) reduction assay. Briefly, 1.0 ml of sterile MTT (1.0 mg/ml in PBS) was added to each well and incubated at 37 °C under low oxygen tension conditions for 1 h. Afterward, 1.0 ml of DMSO was added to each well, and the plates were incubated for 20 min at room temperature, protected from light, and with gentle agitation. Absorbance was obtained using a microplate reader at 540 nm.
In addition to the viability of the biofilm formed on the dentine blocks, the cell growth of S. mutans was measured. After 48 h of biofilm induction on the dentine blocks, 100 µl of the cell suspension from each well was transferred to 96-well plates, and absorbance at 660 nm was obtained to evaluate the turbidity of the suspensionCitation38.
Statistical analysis
Statistical analysis was performed using Statgraphics Centurion 16.1 (Manugistics, Rockville, MD, USA). Initially, Shapiro-Wilk and Levene tests were applied to verify the normality of the distributions and the homoscedasticity of the variances. Based on the results, data regarding contact angle and antibacterial activity of protease inhibitors were analysed using the one-way analysis of variance (ANOVA). Data related to bond strength and gelatinolytic and collagenolytic activity were analysed using the two-way ANOVA. The Tukey HSD test was performed to contrast the means for both analyses. The failure mode and collagen degradation spectroscopic were evaluated qualitatively by the spectra analysis. All analyses were performed at a significance level of α = 0.05.
In vitro CA inhibition assay
The inhibitory activity of the two sulphonamides against S. mutans CA (SmuCA) was assayed using Khalifah’s stopped flow assayCitation39.
Results
Gelatinolytic and collagenolytic activity
By measuring the proteolytic activity through the fluorescence emitted by the cleaved EnzChek substrate, it was possible to determine that, for both substances (SULFA1 and SULFA2), the concentration of 200 µM was more effective in inhibiting collagen proteolysis since the results were statistically equal to the positive control group PHEN. Moreover, both compounds in all concentrations tested (100 µM, 150 µM, and 200 µM) presented a lower proteolytic activity when compared to the CTR group ().
Table 2. Relative fluorescence intensity emitted by the action of dentine gelatins.
Microtensile bond strength
The values of micro tensile bond strength (µTBS) of the experimental groups after different forms of ageing (24 h and 12 months in distilled water) and cariogenic challenge (CC) are shown in . The adhesion strength of the CTR group after CC (21.87 ± 3.74 MPa) and 12 months in distilled water (19.35 ± 2.19 MPa) were lower when compared to CTR after 24 h (31.91 ± 4.06 MPa). The SULFA1 and SULFA2 groups showed no decrease in bond strength values after 12 months of immersion in water or cariogenic challenge.
Table 3. Average values (± DP) of bond strength after storage in distilled water (24 h and 12 months) and cariogenic challenge (CC) by microtensile bond strength assay (MPa).
It may be observed that after 24 h in distilled water and the cariogenic challenge, results obtained with SULFA1 and SULFA2 were comparable to the PHEN group (pre-treatment with phenanthroline, a standard inhibitor of metalloproteases). Interestingly, only SULFA2 was comparable to the PHEN group after one year of ageing in water. The DMSO group after CC had a lower bond strength value when compared to SULFA1 and SULFA2. None of the groups had premature failures during beams preparation or the µTBS test.
Failure mode
Regarding the analysis of the failure pattern after micro tensile in 24 h, there was a significant predominance of mixed failure in all groups and a significant number of adhesive failures in the PHEN group. After 12 months, although the predominance of failures in the groups continued to be mixed, there was a greater number of cohesive failures in resin in the SULFA1 group. After the cariogenic challenge, there was a predominance of mixed failures for all groups and a greater number of adhesive and cohesive failure in dentine for the PHEN group ().
Contact angle measurements
The contact angle values and representative images of each group are depicted in . This analysis showed that the wettability of the dentine surface pre-treated with the substances SULFA1 and SULFA2 was statistically equal to the CTR group. The SULFA1 group presented a smaller contact angle when compared to the PHEN group (p < 0.05).
Figure 3. (A) Average values (±DP) of the contact angle. Slashes by different capital letters are statistically different (HSD of Tukey, p < 0.05). (B) Representative images of the groups: A-SULFA1; B-SULFA2; C-DMSO; D-PHEN; E-CTR. ↓ drop of adhesive in contact with the dentine, forming the contact angle.
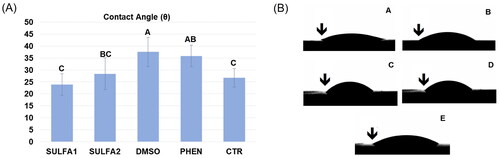
Spectroscopic analysis of collagen degradation
Representative FT-IR spectra of collagen fibres from all experimental groups are shown in . After treatment of the dentine with the substances tested, all spectra, including the control group (without pre-treatment), showed characteristic bands of amide I (1648 cm−1) and amide II (1563 cm−1) (). After 15 days of storage in collagenase solution (B), the amide II band was observed in all groups except for the CTR group.
Antibacterial activity of compounds
Quantitative results for preformed S. mutans biofilms (metabolic activity of biofilm-forming cells) and cell growth (measurement of planktonic cells in the biofilm), in the presence of treated/untreated dentine blocks with SULFA1 and SULFA2, are shown in . The treatment influenced biofilm production on dentine surfaces and cell growth (p < 0.05). The SULFA2 group had lower values of metabolic activity (lower biofilm production) when compared to the CTR (p < 0.05). Both SULFA1 and SULFA2 inhibited the growth of planktonic cells in the suspension used for biofilm induction. The SmuCA inhibitory effects of the two sulphonamides are shown in .
Discussion
The present study aimed to evaluate the effect of dentine pre-treatment with two synthesised sulphonamides CAI derivatives (arylsulphonyl-ureidosulphonamides)Citation29,Citation30 (SULFA1 and SULFA2) on dentine bond strength, dentine surface wettability, collagen preservation of the dentine matrix, and biofilm formation of S. mutans. Sulphonamides constitute an important class of drugs with anti-glaucoma, anti-epileptic, diuretic, and antitumor actionCitation29,Citation30,Citation39. Sulphonamides have also been explored as an alternative treatment against oral diseases. These compounds have shown inhibitory activity on CAs and metalloproteases, both present in S. mutans and Porphyromonas gingivalis, bacteria that cause caries and periodontal diseases, respectively. CAs are essential for developing and replicating these microorganisms, and their inhibition significantly affects bacterial growthCitation24,Citation25. However, to our knowledge, there is no report regarding the inhibitory effect of sulphonamides on the dentinal matrix metalloproteinases. A study of 2000Citation31 explored whether primary sulphonamides acting as effective CA inhibitors also inhibit MMPs. Those simple sulphonamides in fact do not inhibit MMPs, but their N-hydroxy analogs do inhibit both classes of zinc enzymes, the CAs and the MMPs. As arylsulphonyl-ureido benzenesulphonamides (as SULPHA 1 and 2)Citation30 are an underexplored class of highly effective CA inhibitors, we decided to include them in this study, also considering their interesting inhibitory effects on S. mutans CA (see later in the text).
For this study, 2 control groups were used: the positive control group (PHEN) and the negative control group (CTR). Also, it employed an adhesive system already well documented in the literature: the Adper Single Bond 2Citation40,Citation41. The group with the phenanthroline inhibitor was used as a positive control since there is scientific evidence that proves its Effectiveness in inhibiting MMPsCitation42–45, also corroborating the results obtained in this study in the evaluation of degradation of collagen (). 1,10-Phenanthroline is one of the most successful and extensively studied heterocyclic ligands, exhibiting activity against many microorganisms due to its ability to chelate essential metals, interfering with their acquisition and bioavailability for crucial metabolic reactionsCitation42. This inhibitor forms very stable complexes with Zn2+ and, to a lesser extent, with Fe2+, Ca2+, Cu2+, Co2+, and Mn2+Citation43. As expected, the chelation of essential metallic elements impairs the functioning of various biological systems and disrupts microbial cell homeostasis, culminating in various metabolic dysfunctions, including cell deathCitation44,Citation45. Therefore, this chelator cannot be used as a human metalloproteinase inhibitor due to its high cytotoxicity.
According to the dentine bond strength results obtained in this study, SULFA1 maintained its activity after 12 months of storage in water and after the cariogenic challenge, while SULFA2 showed an increase in the bond strength value. Thus, hypothesis 1 may be considered as partially validated since pre-treatment of the dentine surface with sulphonamide derivatives maintained or increased micro tensile bond strength after 12 months and after the cariogenic challenge.
The sulphonamide derivatives tested in the present study (SULFA1 and SULFA2) were solubilised in dimethyl sulphoxide. Therefore, a control group with this solvent was used since Tjäderhane et alCitation46. and Cardenas et alCitation47. observed that using an aqueous solution with DMSO as a dentine pre-treatment was able to preserve bond strength over 12 months. Probably, this effect was observed due to the ability of DMSO to dissociate highly cross-linked collagen into a more dispersed network of apparent fibrils, breaking the auto-associative tendency of water and consequently improving wettability in demineralised dentineCitation46. In the present study, this same effect was observed since the 12-month bond strength value of the DMSO group was maintained compared to the initial value. However, when compared with the experimental groups SULFA1 and SULFA2, the results of bond strength were statistically lower at 24 h, after 12 months, and after exposure to a biofilm of S. mutans (cariogenic challenge), suggesting that the use of DMSO did not enhance the bond strength results of SULFA1 and SULFA2.
Dental caries is a dynamic multifactorial disease that requires a microbial biofilm to act on a suitable substrate. One of the microorganisms widely involved in developing this pathology is S. mutans, a gram-positive bacterium in the oral cavity. When installed on the dental surface and associated with other factors, such as diet and host susceptibility, these bacteria produce acids that promote a pH drop to below 5.5, diffusing through mineralised dental tissues. Consequently, the mineral portion dissolves and exposes the collagen fibres. These unprotected fibres are degraded by the action of MMPs that are activated by low pHCitation5,Citation12,Citation48. Due to this, one of the ways to evaluate the effect of pre-treatment of these substances (SULFA1 and SULFA2) on dentine adhesion strength was the ageing of beams in a biofilm of S. mutans. It was observed an increase in bond strength in the SULFA2 group and maintenance of microtensile bond strength in SULFA1 compared to the other groups after the cariogenic challenge. This can be explained by the inhibitory action of the sulphonamide derivatives tested on MMPs and the antimicrobial effect of SULFA1 and SULFA2 observed in the present study.
The failure pattern described in shows that the SULFA1 group presented a predominance of mixed failures followed by a significant increase in cohesive failure in resin after 12 months in distilled water. This result suggests that the tested substances could maintain the integrity of the dentine/adhesive interface, thus failing in the resin body. SULFA2, DMSO, PHEN, and CTR groups, in 24 h, 12 months, and after cariogenic challenge, presented mainly mixed failure. However, for the PHEN group, it was also possible to observe the predominance of adhesive failure at 24 h and after cariogenic challenge.
Since adhesion requires intimate contact between the adhesive material and the substrate, the dentine surface’s wetting process directly influences the adhesive interface’s quality. The degree of propagation of a liquid over a surface is the measure of wettability, which can be quantified by determining the contact angleCitation15. For the use of conventional adhesive systems, whether simplified or not, acid etching dissolves the smear layer, which is removed by subsequent washing, allowing direct contact of the adhesive with demineralised dentine and, as a result, better surface wettabilityCitation15. Given the above, a treatment after acid etching would negatively affect the contact angle. Therefore, considering the different dentine treatments evaluated, the SULFA1 and SULFA2 groups led to contact angles statistically equal to the CTR group, suggesting that the treatment with these inhibitors could not change the penetration of the adhesive and the formation of the hybrid layer. Thus, the first hypothesis was entirely accepted since sulphonamide derivatives did not influence dentine wettability. These results may be associated with the presence of -NH endings in the SULFA1 and SULFA2 groups, which allow the interaction with the water molecules present in the demineralised dentine through hydrogen bondsCitation49, in addition to interacting with the hydrophilic endings of the adhesive monomers, allowing a better wettability on the substrate pre-treated with the derivatives.
For the spectroscopic analysis of collagen degradation, treatment groups were compared to the PHEN group. After qualitative analysis of the amide I and amide II curves, it was observed that, like phenanthroline, the aryl sulphonyl-ureido sulphonamide derivatives (SULFA1 and SULFA2) at a concentration of 200 µM were also able to reduce collagen degradation () after 15 days in collagenase solution. It may be suggested that the sulphonamide derivatives tested could interact with the active site of MMPs, allowing the inactivation of these enzymes. Therefore, the second hypothesis was accepted concerning the collagen preservation capacity of the dentine matrix by inhibiting the proteolytic activity of collagenases by aryl sulphonyl-ureido sulphonamide derivatives.
According to Dedeoglu et alCitation50,Citation51, sulphonamides have antimicrobial action against S. mutans due to the inhibition of bacterial CAs present in this pathogen. Considering these data, the ability of the sulphonamide derivatives to inhibit cell growth and biofilm formation of S. mutans on dentine blocks was tested. It was observed that both compounds decreased the number of planktonic cells, and SULFA2 decreased the rate of metabolic activity of the cells in the biofilm formed in the dentine blocks. When trying to extrapolate these results to the daily clinic, we can assume that using these sulphonamide derivatives may minimise or hinder the development of recurrent caries at the margins of adhesive restorations. Additionally, we can accept the third hypothesis since the sulphonamide derivatives showed antimicrobial and antibiofilm activity of S. mutans. Indeed, both sulphonamides are potent SmuCA inhibitors, with inhibition constants in the range of 173–215 nM (), which means that they probably interfere with the pathogen’s growth, as already shown for other bacteria and sulphonamide inhibitorsCitation52–68.
In vitro studies have already evaluated the cytotoxicity of these sulphonamide derivatives against different animal cells, showing slight toxicity against the macrophage cells RAW 267.4Citation69 and lower toxicity than amphotericin B against human red blood cellsCitation70. Although we could extrapolate these results to the oral cavity, more specific cellular models should be used in future research.
Conclusions
Based on current data, we can conclude that pre-treatment with the arylsulphonyl-ureido sulphonamide derivatives SULPHA 1 and SULFA2 preserved the adhesive interface even after a cariogenic challenge with S. mutans. It did not interfere negatively with the wettability of the dentine surface, and this result is vital since wettability directly influences the quality of the adhesive interface. They could inhibit MMPs preserving collagen and could still reduce the metabolic activity of S. mutans. Given these results, SULFA1 and SULFA2 could offer improvements in the performance of adhesive systems, allowing a reduction in recurrent risks or the development of recurrent caries at the margins of composite restorations due to their inhibitory action against the bacterial CA present in S. mutans. However, a broader approach to the subject is still needed regarding the use of SULFA1 and SULFA2 in an adhesive protocol or the incorporation of these compounds into experimental adhesive systems, as well as a comparative study with MMP inhibitors already known (e.g. chlorhexidine).
Disclosure statement
No potential conflict of interest was reported by all author(s) except CTS. CT Supuran is Editor-in-Chief of the Journal of Enzyme Inhibition and Medicinal Chemistry. He was not involved in the assessment, peer review, or decision-making process of this paper. The authors have no relevant affiliations or financial involvement with any organisation or entity with a financial interest in or financial conflict with the subject matter or materials discussed in the manuscript. This includes employment, consultancies, honoraria, stock ownership or options, expert testimony, grants or patents received or pending, or royalties.
Additional information
Funding
References
- Spencer P, Ye Q, Park J, Topp EM, Misra A, Marangos O, Wang Y, Bohaty BS, Singh V, Sene F, et al. Adhesive/dentin interface: the weak link in the composite restoration. Ann Biomed Eng. 2010;38(6):1989–2003.
- Huang B, Cvitkovitch DG, Santerre JP, Finer Y. Biodegradation of resin–dentin interfaces is dependent on the restorative material, mode of adhesion, esterase or MMP inhibition. Dent Mater. 2018;34(9):1253–1262.
- Perdigão J, Reis A, Loguercio AD. Dentin adhesion and MMPs: a comprehensive review. J Esthet Restor Dent. 2013;25(4):219–241.
- Maske TT, Kuper NK, Cenci MS, Huysmans MCD. Chlorhexidine, a matrix metalloproteinase inhibitor and the development of secondary caries wall lesions in a microcosm biofilm model. Caries Res. 2019;53(1):107–117.
- Izadora QSM, Ticiano GN, Antonio TS, et al. Inhibition of matrix metalloproteinases: a troubleshooting for dentin adhesion. Restor Dent Endod. 2020;22(45):e31.
- Neves JG, Marcato PD, de Paula E Silva FWG, Mantovani CPT, Prado HS, Aires CP, Massaro TNC, Borsato MC. Synthesis and characterization of an experimental primer containing chitosan nanoparticles - effect on the inactivation of metalloproteinases, antimicrobial activity and adhesive strength. Arch Oral Biol. 2021;127:105148.
- Betancourt DE, Baldion PA, Castellanos JE. Resin-dentin bonding interface: mechanisms of degradation and strategies for stabilization of the hybrid layer. Int J Biomater. 2019;2019:1–11.
- Chaussain C, Boukpessi T, Khaddam M, Tjaderhane L, George A, Menashi S. Dentin matrix degradation by host matrix metalloproteinases: inhibition and clinical perspectives toward regeneration. Front Physiol. 2013;4:308.
- Toledano M, Nieto-Aguilar R, Osorio R, Campos A, Osorio E, Tay FR, Alaminos M. Differential expression of matrix metalloproteinase-2 in human coronal and radicular sound and carious dentine. J Dent. 2010;38(8):635–640.
- Miranda MED, Silva EMD, Oliveira MF, Simmer FS, Santos GBD, Amaral CM. Resin-dentin bond stability of etch-and-rinse adhesive systems with different concentrations of MMP inhibitor GM1489. J Appl Oral Sci. 2020;28:e20190499.
- Carrilho MR, Carvalho RM, de Goes MF, di Hipólito V, Geraldeli S, Tay FR, Pashley DH, Tjäderhane L. Chlorhexidine preserves dentin bond in vitro. J Dent Res. 2007;86(1):90–94.
- Mazzoni A, Tjäderhane L, Checchi V, Di Lenarda R, Salo T, Tay FR, Pashley DH, Breschi L. Role of dentin MMPs in caries progression and bond stability. J Dent Res. 2015;94(2):241–251.
- Tjäderhane L, Nascimento FD, Breschi L, Mazzoni A, Tersariol ILS, Geraldeli S, Tezvergil-Mutluay A, Carrilho M, Carvalho RM, Tay FR, et al. Optimizing dentin bond durability: strategies to prevent hydrolytic degradation of the hybrid layer—a review. Dent Mater. 2013;29(10):999–1011.
- Carrilho MR, Geraldeli S, Tay F, de Goes MF, Carvalho RM, Tjäderhane L, Reis AF, Hebling J, Mazzoni A, Breschi L, et al. In vivo preservation of the hybrid layer by chlorhexidine. J Dent Res. 2007;86(6):529–533.
- da Silva EM, de Sa Rodrigues CU, de Oliveira Matos MP, de Carvalho TR, dos Santos GB, Amaral CM. Experimental etch-and-rinse adhesive systems containing MMP-inhibitors: physicochemical characterization and resin-dentin bonding stability. J Dent. 2015;43:1491–1497.
- Favetti M, Schroeder T, Montagner AF, Correa MB, Pereira-Cenci T, Cenci MS. Effectiveness of pre-treatment with chlorhexidine in restoration retention: a 36-month follow-up randomized clinical trial. J Dent. 2017;60:44–49.
- Mckerrow JH. Parasite proteases. Exp Parasitol. 1989;68(1):111–115.
- Di Fiore A, Supuran CT, Scaloni A, De Simone G. Post-translational modifications in tumor-associated carbonic anhydrases. Amino Acids. 2022;54(4):543–558.
- Mishra CB, Kumari S, Angeli A, Bua S, Mongre RK, Tiwari M, Supuran CT. Discovery of potent carbonic anhydrase inhibitors as effective anticonvulsant agents: drug design, synthesis, and in vitro and in vivo investigations. J Med Chem. 2021;64(6):3100–3114.
- Fares M, Eldehna WM, Bua S, Lanzi C, Lucarini L, Masini E, Peat TS, Abdel-Aziz HA, Nocentini A, Keller PA, et al. Discovery of potent dual-tailed benzenesulfonamide inhibitors of human carbonic anhydrases implicated in glaucoma and in vivo profiling of their intraocular pressure-lowering action. J Med Chem. 2020;63(6):3317–3326.
- Rahman MM, Tikhomirova A, Modak JK, Hutton ML, Supuran CT, Roujeinikova A. Antibacterial activity of ethoxzolamide against Helicobacter pylori strains SS1 and 26695. Gut Pathog. 2020;12:20.
- De Luca V, Petreni A, Nocentini A, Scaloni A, Supuran CT, Capasso C. Effect of sulfonamides and their structurally related derivatives on the activity of i-carbonic anhydrase from Burkholderia territorii. IJMS. 2021;22(2):571.
- Angeli A, Etxebeste-Mitxeltorena M, Sanmartín C, Espuelas S, Moreno E, Azqueta A, Parkkila S, Carta F, Supuran CT. Tellurides bearing sulfonamides as novel inhibitors of leishmanial carbonic anhydrase with potent antileishmanial activity. J Med Chem. 2020;63(8):4306–4314.
- Supuran CT. Novel carbonic anhydrase inhibitors. Future Med Chem. 2021;13(22):1935–1937.
- Supuran CT, Capasso C. An overview of the bacterial carbonic anhydrases. Metabolites. 2017;7(4):56.
- Mishra CB, Tiwari M, Supuran CT. Progress in the development of human carbonic anhydrase inhibitors and their pharmacological applications: where are we today? Med Res Rev. 2020;40(6):2485–2565.
- Alterio V, Di Fiore A, D'Ambrosio K, Supuran CT, De Simone G. Multiple binding modes of inhibitors to carbonic anhydrases: how to design specific drugs targeting 15 different isoforms? Chem Rev. 2012;112(8):4421–4468.
- Supuran CT. Advances in structure-based drug discovery of carbonic anhydrase inhibitors. Expert Opin Drug Discov. 2017;12(1):61–88.
- Bozdag M, Ferraroni M, Carta F, Vullo D, Lucarini L, Orlandini E, Rossello A, Nuti E, Scozzafava A, Masini E, et al. Structural insights on carbonic anhydrase inhibitory action, isoform selectivity, and potency of sulfonamides and coumarins incorporating arylsulfonylureido groups. J Med Chem. 2014;57(21):9152–9167.
- Scozzafava A, Supuran CT. Carbonic anhydrase inhibitors. Arylsulfonylureido- and arylureido-substituted aromatic and heterocyclic sulfonamides: towards selective inhibitors of carbonic anhydrase isozyme I. J Enzyme Inhib. 1999;14(5):343–363.
- Scozzafava A, Supuran CT. Carbonic anhydrase and matrix metalloproteinase inhibitors: sulfonylated amino acid hydroxamates with MMP inhibitory properties act as efficient inhibitors of CA isozymes I, II, and IV, and N-hydroxysulfonamides inhibit both these zinc enzymes. J Med Chem. 2000;43(20):3677–3687.
- Li J, Chen B, Hong N, Wu S, Li Y. Effect of baicalein on matrix metalloproteinases and durability of resin-dentin bonding. Oper Dent. 2018;43(4):426–436.
- Carvalho FG, Carlo HL, Sacramento PA, de Barros S, Santos RL, Puppin-Rontani RM. Biodegradation of caries-affected dentin bonding interface of fluoride and MDPB-containing adhesive system. Int J Adhes Adhes. 2013;47:134–140.
- Bertolini MM, Portela MB, Curvelo JAR, Soares RMA, Lourenço EJV, Telles DM. Resins-based denture soft lining materials modified by chlorhexidine salt incorporation: an in vitro analysis of antifungal activity, drug release and hardness. Dent Mater. 2014;30(8):793–798.
- Ricci HA, Scheffel DLS, de Souza Costa CA, dos Santos FJ, Jafelicci M, Hebling J. Wettability of chlorhexidine treated non-carious and caries-affected dentine. Aust Dent J. 2014;59(1):37–42.
- Kishen A, Shrestha S, Shrestha A, Cheng C, Goh C. Characterizing the collagen stabilizing effect of cross-linked chitosan nanoparticles against collagenase degradation. Dent Mater. 2016;32(8):968–977.
- Dias PG, da Silva EM, Carvalho C. M d, Miranda M. E d S, Portela MB, Amaral CM. Characterization and antibacterial effect of an experimental adhesive containing different concentrations of proanthocyanidin. J Adhes Dent. 2020;22(2):139–147.
- Chylewska A, Dąbrowska AM, Ramotowska S, Maciejewska N, Olszewski M, Bagiński M, Makowski M. Photosensitive and pH-dependent activity of pyrazine-functionalized carbazole derivative as promising antifungal and imaging agent. Sci Rep. 2020;10(1):11767.
- Khalifah RG. The carbon dioxide hydration activity of carbonic anhydrase. I. Stop-flow kinetic studies on the native human isoenzymes B and C. J Biol Chem. 1971;246(8):2561–2573.
- Scherrer SS, Cesar PF, Swain MV. Direct comparison of the bond strength results of the different test methods: a critical literature review. Dent Mater. 2010;26(2):e78–e93.
- Nicoloso GF, Carvalho MP, Soares FZM, Susin AH, Rocha RO. Short-term aging and the dentin bond strength of adhesive systems. Gen Dent. 2019;67(3):71–76.
- . Sousa-Santos O, Neto-Neves EM, Ferraz KC, Ceron CS, Rizzi E, Gerlach RF, Tanus-Santos JE. Antioxidant treatment protects against matrix metalloproteinase activation and cardiomyocyte injury during acute pulmonary thromboembolism. Naunyn Schmiedebergs Arch Pharmacol. 2012;385(7):685–696.
- Tay CX, Quah SY, Lui JN, Yu VSH, Tan KS. Matrix metalloproteinase inhibitor as an antimicrobial agent to eradicate Enterococcus faecalis biofilm. J Endod. 2015;41(6):858–863.
- McCann M, Santos ALS, da Silva BA, Romanos MTV, Pyrrho AS, Devereux M, Kavanagh K, Fichtner I, Kellett A. In vitro and in vivo studies into the biological activities of 1,10- phenanthroline, 1,10-phenanthroline-5,6-dione and its copper(ii) and silver(i) complexes. Toxicol Res. 2012;1(1):47–54.
- Souza AP, Gerlach RF, Line SRP. Inhibition of human gelatinases by metals released from dental amalgam. Biomaterials. 2001;22(14):2025–2030.
- Tjäderhane L, Mehtälä P, Scaffa P, Vidal C, Pääkkönen V, Breschi L, Hebling J, Tay FR, Nascimento FD, Pashley DH, et al. The effect of dimethyl sulfoxide (DMSO) on dentin bonding and nanoleakage of etch-and-rinse adhesives. Dent Mater. 2013;29(10):1055–1062.
- Cardenas AFM, Araujo LCR, Szesz AL, de Jesus Tavarez RR, Siqueira F. S F d, Reis A, Loguercio AD. Influence of application of dimethyl sulfoxide on the bonding properties to eroded dentin. J Adhes Dent. 2021;23(6):589–598.
- Chaussain-Miller C, Fioretti F, Goldberg M, Menashi S. The role of matrix metalloproteinases (MMPs) in human caries. J Dent Res. 2006;85(1):22–32.
- Farge P, Alderete L, Ramos SM. Dentin wetting by three adhesive systems: influence of etching time, temperature and relative humidity. J Dent. 2010;38(9):698–706.
- Dedeoglu N, DeLuca V, Isik S, Yildirim H, Kockar F, Capasso C, Supuran CT. Sulfonamide inhibition study of the β-class carbonic anhydrase from the caries producing pathogen Streptococcus mutans. Bioorg Med Chem Lett. 2015;25(11):2291–2297.
- Dedeoglu N, De Luca V, Isik S, Yildirim H, Kockar F, Capasso C, Supuran CT. Cloning, characterization and anion inhibition study of a β-class carbonic anhydrase from the caries producing pathogen Streptococcus mutans. Bioorg Med Chem. 2015;23(13):2995–3001.
- Supuran CT. Bacterial carbonic anhydrases as drug targets: toward novel antibiotics? Front Pharmacol. 2011;2:34.
- Di Fiore A, De Luca V, Langella E, Nocentini A, Buonanno M, Monti SM, Supuran CT, Capasso C, De Simone G. Biochemical, structural, and computational studies of a γ-carbonic anhydrase from the pathogenic bacterium Burkholderia pseudomallei. Comput Struct Biotechnol J. 2022;20:4185–4194.
- Capasso C, Supuran CT. Anti-infective carbonic anhydrase inhibitors: a patent and literature review. Expert Opin Ther Pat. 2013;23(6):693–704.
- Nishimori I, Vullo D, Minakuchi T, Scozzafava A, Osman SM, AlOthman Z, Capasso C, Supuran CT. Anion inhibition studies of two new β-carbonic anhydrases from the bacterial pathogen Legionella pneumophila. Bioorg Med Chem Lett. 2014;24(4):1127–1132.
- Capasso C, Supuran CT. An overview of the alpha-, beta- and gamma-carbonic anhydrases from Bacteria: can bacterial carbonic anhydrases shed new light on evolution of bacteria? J Enzyme Inhib Med Chem. 2015;30(2):325–332.
- Amedei A, Capasso C, Nannini G, Supuran CT. Microbiota, bacterial carbonic anhydrases, and modulators of their activity: links to human diseases? Mediators Inflamm. 2021;2021:6926082.
- Nocentini A, Supuran CT, Capasso C. An overview on the recently discovered iota-carbonic anhydrases. J Enzyme Inhib Med Chem. 2021;36(1):1988–1995.
- Campestre C, De Luca V, Carradori S, Grande R, Carginale V, Scaloni A, Supuran CT, Capasso C. Carbonic anhydrases: new perspectives on protein functional role and inhibition in Helicobacter pylori. Front Microbiol. 2021;12:629163.
- Del Prete S, Nocentini A, Supuran CT, Capasso C. Bacterial ι-carbonic anhydrase: a new active class of carbonic anhydrase identified in the genome of the Gram-negative bacterium Burkholderia territorii. J Enzyme Inhib Med Chem. 2020;35(1):1060–1068.
- Supuran CT, Capasso C. Biomedical applications of prokaryotic carbonic anhydrases. Expert Opin Ther Pat. 2018;28(10):745–754.
- Flaherty DP, Seleem MN, Supuran CT. Bacterial carbonic anhydrases: underexploited antibacterial therapeutic targets. Future Med Chem. 2021;13(19):1619–1622.
- Hewitt CS, Abutaleb NS, Elhassanny AEM, Nocentini A, Cao X, Amos DP, Youse MS, Holly KJ, Marapaka AK, An W, et al. Structure-activity relationship studies of acetazolamide-based carbonic anhydrase inhibitors with activity against Neisseria gonorrhoeae. ACS Infect Dis. 2021;7(7):1969–1984.
- Abutaleb NS, Elhassanny AEM, Nocentini A, Hewitt CS, Elkashif A, Cooper BR, Supuran CT, Seleem MN, Flaherty DP. Repurposing FDA-approved sulphonamide carbonic anhydrase inhibitors for treatment of Neisseria gonorrhoeae. J Enzyme Inhib Med Chem. 2022;37(1):51–61.
- An W, Holly KJ, Nocentini A, Imhoff RD, Hewitt CS, Abutaleb NS, Cao X, Seleem MN, Supuran CT, Flaherty DP, et al. Structure-activity relationship studies for inhibitors for vancomycin-resistant Enterococcus and human carbonic anhydrases. J Enzyme Inhib Med Chem. 2022;37(1):1838–1844.
- De Luca V, Carginale V, Supuran CT, Capasso C. The gram-negative bacterium Escherichia coli as a model for testing the effect of carbonic anhydrase inhibition on bacterial growth. J Enzyme Inhib Med Chem. 2022;37(1):2092–2098.
- Supuran CT, Capasso C. Antibacterial carbonic anhydrase inhibitors: an update on the recent literature. Expert Opin Ther Pat. 2020;30(12):963–982.
- Giovannuzzi S, Hewitt CS, Nocentini A, Capasso C, Costantino G, Flaherty DP, Supuran CT. Inhibition studies of bacterial α-carbonic anhydrases with phenols. J Enzyme Inhib Med Chem. 2022;37(1):666–671.
- Vermelho AB, da Silva Cardoso V, Ricci Junior E, Dos Santos EP, Supuran CT. Nanoemulsions of sulfonamide carbonic anhydrase inhibitors strongly inhibit the growth of Trypanosoma cruzi. J Enzyme Inhib Med Chem. 2018;33(1):139–146.
- Silva Cardoso V, Vermelho AB, Ricci Junior E, Almeida Rodrigues I, Mazotto AM, Supuran CT. Antileishmanial activity of sulphonamide nanoemulsions targeting the β-carbonic anhydrase from Leishmania species. J Enzyme Inhib Med Chem. 2018;33(1):850–857.