Abstract
A series of 21 novel compounds containing a thiosemicarbazone moiety were designed and synthesised based on hit compound 1 from our in-house compound library screening. Most compounds showed potent antifungal activity in vitro against seven common pathogenic fungi. Notably, all compounds showed high potency against Candida glabrata 537 (MIC = ≤0.0156-2 µg/mL). Of note, compounds 5j and 5r displayed excellent antifungal activity against Candida krusei 4946 and Candida auris 922. Additionally, compounds 5j and 5r also showed high potency against 15 C. glabrata isolates with MIC values ranging from 0.0625 µg/mL to 4 µg/mL, with compound 5r being slightly superior to 5j. Moreover, compound 5r has certain effect against biofilm formation of C. glabrata. Furthermore, compound 5r has minimal cytotoxicity against HUVECs with an IC50 value of 15.89 µg/mL and no haemolysis at 64 µg/mL. Taken together, these results suggest that promising lead compound 5r deserves further investigation.
Introduction
Invasive candidiasis can be a very serious and life-threatening fungal infection that is caused by Candida species with Candida albicans, Candida glabrata, Candida krusei, Candida tropicalis, and Candida parapsilosis being the five most frequently clinically isolatedCitation1–4. Although C. albicans is still the most common cause of invasive candidiasis, the incidence of C. glabrata infections is rising and is now the second most common causative pathogen of invasive candidiasisCitation4–8. In 2022, C. glabrata was named the first Candida species in the first ever World Health Organisation (WHO) fungal priority pathogen High Priority Group.
Unlike C. albicans, C. glabrata always grows as unicellular yeast and also has the ability to form filamentous pseudohyphae but has lost the ability to undergo true hyphal growthCitation7,Citation9,Citation10. Genome sequencing identified that C. glabrata shares a common ancestor with Saccharomyces cerevisiae rather than C. albicansCitation7. The ploidy status of C. glabrata varies dramatically under different conditions, including the ability to switch ploidy between haploid, aneuploid, diploid, and hyper-diploid forms. Variations in ploidy, as well as morphological transitions, contribute to C. glabrata’s ability to develop antifungal resistanceCitation10. C. glabrata strains easily and quickly acquire drug resistance to azoles with some strains having intrinsic resistance to azolesCitation11. Moreover, C. glabrata also displays high intrinsic resistance to echinocandins, and polyene-resistant clinical C. glabrata isolates are emergingCitation12,Citation13. Single drug and multi-drug resistance of C. glabrata is becoming increasingly prevalent and difficult to treat. Despite the fact that five classes of antifungal drugs () have been approved for the treatment of IFIs to date (the azoles (6), polyenes (1), flucytosine (1), echinocandins (3) and triterpenoid (1)), the current antifungal arsenal remains inadequateCitation14. Therefore, new antifungal drugs with high potency and novel mechanisms against C. glabrata are urgently needed.
Previously, we carried out a compound library screening with the aim of identifying novel scaffold antifungals with potent in vitro activity against common human pathogenic fungi, including C. albicans, Cryptococcus neoformans and Aspergillus fumigatus. Based on this compound library screening work, we identified several promising antifungal hitsCitation15–17. Among them, hit 1 () displayed moderate antifungal activity against C. albicans SC5314 and C. neoformans H99 with MIC values of 8 µg/mL and 2 µg/mL, respectively. Further investigation revealed that hit 1 has excellent activity against C. glabrata 537 with an MIC value of 0.0313 µg/mL and without obvious cytotoxicity.
Thiosemicarbazone derivatives have been reported to possess a wide range of biological activities including anticancerCitation18, antiviralCitation19, antibacterialCitation20, antioxidantCitation21, anti-Alzheimer’sCitation22, antituberculosisCitation23, antimalarialCitation24 and antifungal activityCitation25. Hydroxy-phenylhydrazone is a typical fragment of pan assay interference compounds (PAINS)Citation26,Citation27. However, our hit compound 1 possesses promising and highly selective anti-C. glabrata activity, giving us confidence to further explore this class of compounds. Herein, we report the design, synthesis, and antifungal activity evaluation of these thiosemicarbazone derivatives.
Results and discussion
Chemistry
The facile synthetic route for the preparation of target compounds 1 and 5a-5t is outlined in Scheme 1. The intermediates 3a-3s were synthesised from compounds 2a-2s and hydrazine hydrate in good yields. Next, compounds 3a-3s were reacted with hydroxy-benzyl aldehyde (4a-4c) to give the target compounds 1 and 5a-5t.
In vitro antifungal activity
All target compounds (hit 1 and 5a-5t) were tested for their minimum inhibitory concentration (MIC) in vitro against seven common human pathogenic fungi including C. albicans SC5314, C. neoformans H99, C. parapsilosis 22019, C. glabrata 537, C. krusei 439, C. tropicalis 2718 and Candida auris 922 (). Fluconazole (FLC), amphotericin B (AmB) and caspofungin (CAS) were used as reference drugs. MICs were determined using the Clinical and Laboratory Standards Institute (CISI, M27-A3 and M60) standard micro broth dilution methodCitation28,Citation29. The MICs were defined as the minimum concentration of drug to inhibit ≥50% growth of fungal cells compared to that of a drug-free control.
Table 1. In vitro antifungal activity of the target compounds.
In this study, we retained the ortho-hydroxy-phenylhydrazone moiety on the right side of the hit compound 1 and varied the substituents on the left benzene ring to examine the antifungal activity. The introduction of fluorine atoms on the left phenyl ring gave compounds 5a (2-F), 5b (3-F), 5c (4-F), 5d (2,4-diF) and 5e (3,4-diF). The 2-F substitution led to a 4-fold and 8-fold decrease in activity against C. neoformans H99 and C. parapsilosis 22019, respectively. The 3-F substitution resulted in a 2-fold increase in activity against C. neoformans H99, C. krusei 439, C. parapsilosis 22019, C. tropicalis 2718 and C. auris 922. The 4-F substitution promoted anti-C. neoformans activity, but resulted in a significant decrease in activity against all other tested fungi. Compounds 5d (2,4-diF) and 5e (3,4-diF) demonstrated that the meta-substitution favoured anti-C. glabrata activity. Compounds 5f (2-Cl), 5g (3-Cl) and 5h (4-Cl) were obtained by the introduction of chlorine atoms. Like compound 5a (2-F), the 2-Cl substitution was also detrimental to the anti-C. glabrata activity which again indicated that the ortho-substitution was not favoured. In contrast, compounds 5g (3-Cl) and 5h (4-Cl) showed improved antifungal activity against C. glabrata 537 compared to hit 1, with compound 5g (3-Cl) exhibiting slightly better antifungal activity against C. neoformans H99, C. parapsilosis 22019, C. tropicalis 2718 and C. auris 922 than that of compound 5h (4-Cl). The introduction of alkanes yielded compounds 5j (4-t-Bu), 5k (3-Me) and 5 l (3,5-diMe), which caused a 4-fold increase in activity against C. albicans SC5314 and a 2–4-fold increase in activity against C. neoformans H99. Compound 5j had a 32-fold and 64-fold increase in activity against C. krusei 439 and C. auris 922, respectively. The introduction of electron-withdrawing groups yielded compounds 5i (4-CN), 5m (4-CF3) and 5n (3-CF3), and the three compounds maintained good activity against C. glabrata 537. In comparison, the 3-CF3 substitution was more beneficial. When a second -CF3 was introduced, compound 5r (3,5-diCF3) was obtained and exhibited the best activity of all compounds with a 32-fold and 256-fold increase in activity against C. krusei 439 and C. auris 922. The benzene ring was replaced with pyridine, cyclohexane, and n-hexane to obtain compounds 5o, 5p, and 5q, respectively. The pyridinyl substitution led to a decrease in activity against the majority of strains, and a cyclohexane substitution resulted in a 16-fold reduction in activity against C. glabrata 537. N-hexyl substitution showed a slight reduction in activity against C. albicans SC5314, but at least a 2-fold increase in activity against C. glabrata 537. Finally, we examined the effect of the position of the hydroxyl group on the activity of compound 5r. Both compounds were significantly less active against C. glabrata 537, with 64-fold and 32-fold reductions, respectively. However, unexpectedly, both compounds retained moderate antifungal activity against other fungi.
We selected the most active compounds, 5j and 5r, for further investigation into their antifungal effect against 15 strains of C. glabrata (). The antifungal activity of both compounds was superior to fluconazole with MIC values of 0.0625–4 µg/mL. In comparison to 5j, compound 5r showed comparable activity against 10 C. glabrata strains (ATCC2001, 203, 204, YC1-34, 338, ATCC28226, 1740, 1843, 1845 and GH200604) and slightly better activity against 5 C. glabrata strains (ATCC1182, 202, Y10-14, Y10-4 and 337). In addition, compounds 5j and 5r displayed potent fungicidal activity against three C. glabrata strains (ATCC2001, ATCC1182, and ATCC28226) with minimum fungicidal concentration (MFC) values of 8 µg/mL. Our results demonstrated that compounds 5j and 5r have moderate to excellent activities against C. glabrata, and much better than FLC.
Table 2. In vitro activity of selected compounds against Candida glabrata.
5j and 5r inhibits the biofilm formation of C. glabrata
Biofilm formation limits drug penetration and is responsible for C. glabrata insensitivity to antifungals, thereby rendering C. glabrata cells more resistant to many of antifungal drugsCitation30,Citation31. Here, we examined the inhibitory effect of 5j and 5r against biofilm formation in C. glabrata using the CCK-8 reduction assay and FLC as a reference drug. Compounds 5j and 5r displayed inhibitory activity against C. glabrata biofilm formation in a dose-dependent manner (). Compound 5r at 0.5 µg/mL significantly inhibited biofilm formation by approximately 15% (p < 0.05); the inhibitory effect on biofilm formation was further improved at higher concentrations. Approximately 50% of biofilm formation was inhibited by 5r at 2 µg/mL and showed no significant difference when compared with that of FLC at the same concentration, indicating that compound 5r has certain inhibitory activity against the biofilm formation of C. glabrata.
Cell viability and hemolysis assay
Since thiosemicarbazone derivatives showed high potency against C. glabrata spp., we employed the CCK-8 assay to further confirm that the antifungal effects of compounds 1, 5j, 5n and 5r were not due to general cytotoxicity. FLC and AmB were used as reference drugs. HUVEC cells were treated with various concentrations (1, 2, 4, 8, and 16 µg/mL) of FLC, AmB, or compounds 1, 5j, 5n, and 5r for 24 h. Compounds 1 and 5r did not cause significant cytotoxicity below 16 µg/mL, but were more toxic than FLC (). The IC50 value of compound 1 was greater than 16 µg/mL, and the IC50 value of 5n and 5r was 8.07 µg/mL and 15.89 µg/mL, respectively. Compound 5j showed mild cytotoxicity with an IC50 value of 3.76 µg/mL.
Figure 4. The in vitro toxicity evaluation of 5j, 5n, 5r, 1, FLC and AmB. The cytotoxic effects of compounds 5j, 5n, 5r and 1, compared to that of FLC on HUVECs viability was assessed by the CCK-8 test. FLC, fluconazole; AmB, amphotericin B.
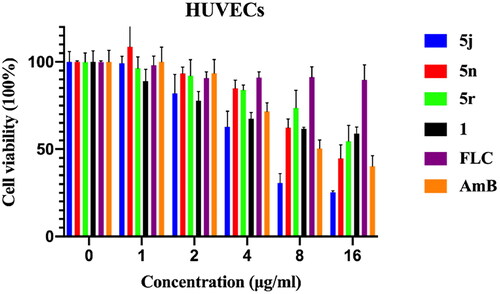
The average haemolysis data for the test compounds against rabbit red blood cells is shown in . AmB caused substantial haemolysis of rabbit erythrocytes at 32 µg/mL and 64 µg/mL with 53.5% and 100% haemolysis, respectively. In contrast, compounds 1, 5j, 5n, 5r, and FLC did not show significant haemolysis at 64 µg/mL.
Figure 5. Haemolytic effect of 5j, 5n, 5r, 1, FLC and AmB against rabbit red blood cells at different indicated concentrations.
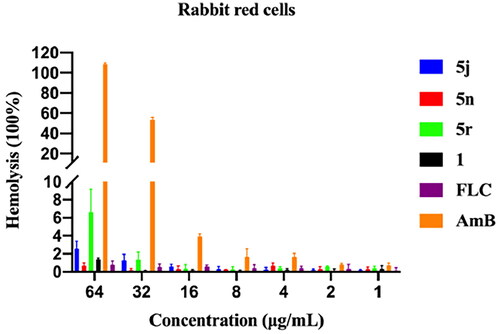
The cytotoxicity and haemolysis assays indicated that the thiosemicarbazone derivatives have good safety profiles.
Theoretical evaluation of ADME/T properties
In silico ADMET prediction of compounds 1, 5j, 5r, and FLC was performed using the free SwissADME online toolCitation32. The Brain Or IntestinaL EstimateD permeation (BOILED-Egg) method is a graphical model that works by calculating the polarity and lipophilicity of small molecules. Compounds 1, 5j, and FLC were located in the white circle, representing that these three compounds were highly absorbed through the gastrointestinal tract (). In contrast, compound 5r was located outside of the white circle, representing low absorption of compound 5r through the gastrointestinal tract. In addition, compounds 1, 5j, and 5r (red spot) are not substrates for P-glycoprotein which perhaps facilitates overcoming efflux pump-mediated resistance mechanisms in pathogenic fungi. Furthermore, as shown in the bioavailability radar (), the physicochemical characteristics, lipophilicity, solubility, pharmacokinetics, and drug-likeness properties of compounds 1, 5j, 5r and FLC were all located in the pink area (optimal range), indicating that compounds 1, 5j, 5r, like FLC, are good lead compounds.
Conclusion
In summary, we designed a series of 21 thiosemicarbazone derivatives, synthesised them, and their in vitro antifungal activities were evaluated. The majority of compounds showed moderate in vitro antifungal activity against seven common pathogenic fungi. Notably, all compounds showed high potency against C. glabrata 537 with MIC values ranging from ≤ 0.0156 µg/mL to 2 µg/mL. The most potent compounds, 5j and 5r, displayed excellent antifungal activity against Candida krusei 4946 (0.0625 µg/mL and 0.0625 µg/mL, respectively) and Candida auris 922 (0.0625 µg/mL and ≤0.0156, respectively). In addition, compounds 5j and 5r also showed high potency against a further 15 C. glabrata isolates with MIC values ranging from 0.0625 µg/mL to 4 µg/mL, with compound 5r being slightly superior to 5j. Moreover, compound 5r had a certain anti-biofilm effect against C. glabrata ATCC28226, which was comparable to that of fluconazole. Furthermore, compound 5r showed low cytotoxicity against HUVECs with an IC50 value of 15.89 µg/mL and no haemolysis at 64 µg/mL. Further structure optimisation and mechanism of action studies of compound 5r are currently under investigation.
Experiment section
Strains and agents
C. albicans SC5314, C. neoformans H99, C. glabrata reference strains (ATCC2001, ATCC1182 and ATCC28226), and C. parapsilosis ATCC22019 were from ATCC collection. C. glabrata clinical strains (537, 202, 203, 204, Y10-14, Y10-4, YC1-34, 337, 338, 1740, 1843, 1845, GH200604), C. krusei 4946, C. tropicalis 2718, and C. auris 922 were from Shanghai Changzheng Hospital (China) and from the fungi collection of our laboratory. Frozen stocks of isolates stored at −80 °C in yeast extract-peptone-dextrose (YPD) medium (1% yeast extract, 2% peptone, 2% dextrose) supplemented with 40% (v/v) glycerol were cultured twice on YPD plates at 30 °C before use. All solvents and agents (including reference drugs: AmB, FLC, and CAS) were purchased from Adamas-beta and used directly or dried prior to use if necessary.
The synthesis of target compounds 1 and 5a-5t
Step 1: Compound 2 (10 mmol) was added to a solution of hydrazinehydrate (85%, 100 mmol) in THF (20 mL) at 0 °C. The resulting mixture was stirred at 0 °C for 1 h. The reaction was monitored by TLC. Once the reaction had completed, the mixture was warmed to room temperature and then poured into water. The precipitated solid was filtered and dried in vacuum to give intermediate 3 a good yield.
Step 2: Compound 3 (1 mmol) was added to a solution of hydroxy-benzyl aldehyde 4 (1 mmol) in 95% Ethanol (10 mL), and the resulting mixture was stirred at 80 °C for 5 h. The reaction was monitored by TLC. Once the reaction had finished, the mixture was warmed to room temperature, water was then added, and the precipitated solid was filtered and dried in a vacuum to give the target compound a good yield.
1: 128 mg, yield: 47%. White solid. 1H NMR (300 MHz, DMSO) δ 11.76 (s, 1H), 10.05 (s, 1H), 9.97 (s, 1H), 8.49 (s, 1H), 8.08 (d, J = 7.6 Hz, 1H), 7.57 (d, J = 8.0 Hz, 2H), 7.36 (t, J = 7.6 Hz, 2H), 7.26 − 7.17 (m, 2H), 6.90 − 6.81 (m, 2H). 13C NMR (75 MHz, DMSO) δ 175.75, 156.63, 140.13, 139.17, 131.36, 128.06, 127.11, 125.70, 125.20, 120.28, 119.26, 116.07. MS (ESI) m/z: 272.1 [M + H]+.
5a: 161 mg, yield: 56%. White solid. 1H NMR (300 MHz, DMSO) δ 11.89 (s, 1H), 9.95 (s, 1H), 9.91 (s, 1H), 8.48 (s, 1H), 8.05 (d, J = 7.8 Hz, 1H), 7.55 (t, J = 7.9 Hz, 1H), 7.36 − 7.19 (m, 4H), 6.90 − 6.80 (m, 2H). 13C NMR (75 MHz, DMSO) δ 176.95, 158.87, 156.65, 155.60, 140.13, 131.41, 130.06, 128.03, 127.93, 127.35, 127.19, 126.88, 123.99, 123.95, 120.25, 119.25, 116.10, 115.77, 115.51. MS (ESI) m/z: 290.1 [M + H]+.
5b: 135 mg, yield: 47%. White solid. 1H NMR (300 MHz, DMSO) δ 11.88 (s, 1H), 10.11 (s, 1H), 9.99 (s, 1H), 8.50 (s, 1H), 8.08 (d, J = 7.3 Hz, 1H), 7.63 (d, J = 11.1 Hz, 1H), 7.46 (d, J = 8.1 Hz, 1H), 7.38 (dd, J = 15.2, 7.5 Hz, 1H), 7.25 (t, J = 7.7 Hz, 1H), 7.05 − 6.99 (m, 1H), 6.90 − 6.82 (m, 2H). 13C NMR (75 MHz, DMSO) δ 175.43, 163.09, 159.89, 156.68, 140.96, 140.82, 140.54, 131.45, 129.48, 129.36, 127.07, 121.04, 121.01, 120.12, 119.21, 116.05, 112.11, 111.78, 111.66, 111.38. MS (ESI) m/z: 290.1 [M + H]+.
5c: 145 mg, yield: 50%. White solid. 1H NMR (300 MHz, DMSO) δ 11.78 (s, 1H), 10.04 (s, 1H), 9.96 (s, 1H), 8.48 (s, 1H), 8.08 (d, J = 7.7 Hz, 1H), 7.57 − 7.52 (m, 2H), 7.26 − 7.16 (m, 3H), 6.90 − 6.81 (m, 2H). 13C NMR (75 MHz, DMSO) δ 176.10, 161.20, 158.00, 156.61, 140.16, 135.54, 135.50, 131.36, 128.07, 127.96, 127.07, 120.25, 119.22, 116.05, 114.81, 114.51. MS (ESI) m/z: 290.1 [M + H]+.
5d: 191 mg, yield: 62%. White solid. 1H NMR (300 MHz, DMSO) δ 11.92 (s, 1H), 9.95 (s, 1H), 9.86 (s, 1H), 8.48 (s, 1H), 8.06 (d, J = 7.4 Hz, 1H), 7.50 (dd, J = 15.3, 8.8 Hz, 1H), 7.37 − 7.29 (m, 1H), 7.23 (t, J = 7.8 Hz, 1H), 7.10 (t, J = 8.4 Hz, 1H), 6.90 − 6.80 (m, 2H). 13C NMR (75 MHz, DMSO) δ 177.34, 156.63, 140.21, 131.66, 131.52, 131.40, 126.87, 124.11, 124.06, 123.95, 123.89, 120.22, 119.21, 116.08, 111.02, 110.73, 104.46, 104.14, 104.11, 103.79. MS (ESI) m/z: 308.1 [M + H]+.
5e: 175 mg, yield: 57%. White solid. 1H NMR (300 MHz, DMSO) δ 11.92 (s, 1H), 10.13 (s, 1H), 10.02 (s, 1H), 8.51 (s, 1H), 8.10 (d, J = 7.5 Hz, 1H), 7.81 − 7.74 (m, 1H), 7.48 − 7.40 (m, 2H), 7.28 − 7.22 (m, 1H), 6.90 − 6.82 (m, 2H). 13C NMR (75 MHz, DMSO) δ 175.80, 156.72, 150.10, 149.92, 148.55, 148.38, 146.87, 146.69, 145.32, 145.16, 140.55, 136.23, 136.19, 136.11, 136.07, 131.51, 127.06, 122.43, 122.39, 122.35, 122.31, 120.16, 119.23, 116.61, 116.38, 116.08, 115.06, 114.79. MS (ESI) m/z: 308.1 [M + H]+.
5f: 166 mg, yield: 54%. White solid. 1H NMR (300 MHz, DMSO) δ 11.95 (s, 1H), 10.04 (s, 1H), 9.99 (s, 1H), 8.49 (s, 1H), 8.02 (d, J = 7.5 Hz, 1H), 7.76 (dd, J = 7.8, 1.4 Hz, 1H), 7.54 (dd, J = 7.8, 1.5 Hz, 1H), 7.37 (td, J = 7.6, 1.5 Hz, 1H), 7.32 − 7.26 (m, 1H), 7.23 − 7.21 (m, 1H), 6.90 − 6.81 (m, 2H). 13C NMR (75 MHz, DMSO) δ 176.22, 156.69, 140.07, 136.53, 131.45, 130.17, 129.37, 129.27, 127.54, 127.10, 126.69, 120.20, 119.32, 116.13. MS (ESI) m/z: 306.1 [M + H]+.
5g: 147 mg, yield: 48%. White solid. 1H NMR (300 MHz, DMSO) δ 11.89 (s, 1H), 10.11 (s, 1H), 10.01 (s, 1H), 8.50 (s, 1H), 8.09 (d, J = 7.6 Hz, 1H), 7.77 (s, 1H), 7.60 (d, J = 8.1 Hz, 1H), 7.38 (t, J = 8.0 Hz, 1H), 7.25 (t, J = 6.9 Hz, 2H), 6.90 − 6.82 (m, 2H). 13C NMR (151 MHz, DMSO) δ 175.56, 156.73, 140.68, 132.18, 131.54, 129.58, 127.12, 124.99, 124.84, 124.01, 120.17, 119.28, 116.11. MS (ESI) m/z: 306.1 [M + H]+.
5h: 192 mg, yield: 63%. White solid. 1H NMR (300 MHz, DMSO) δ 11.86 (s, 1H), 10.09 (s, 1H), 10.00 (s, 1H), 8.49 (s, 1H), 8.09 (d, J = 7.6 Hz, 1H), 7.63 − 7.59 (m, 2H), 7.43 − 7.38 (m, 2H), 7.26 − 7.21 (m, 1H), 6.89 − 6.81 (m, 2H). 13C NMR (75 MHz, DMSO) δ 175.71, 156.66, 140.38, 138.17, 131.44, 129.15, 127.91, 127.33, 127.07, 120.19, 119.23, 116.06. MS (ESI) m/z: 306.1 [M + H]+.
5i: 199 mg, yield: 67%. White solid. 1H NMR (300 MHz, DMSO) δ 12.03 (s, 1H), 10.27 (s, 1H), 10.02 (s, 1H), 8.52 (s, 1H), 8.07 (d, J = 7.6 Hz, 1H), 7.96 (d, J = 8.3 Hz, 2H), 7.81 (d, J = 8.3 Hz, 2H), 7.26 (t, J = 7.7 Hz, 1H), 6.90 − 6.83 (m, 2H). 13C NMR (75 MHz, DMSO) δ 175.08, 156.81, 143.57, 140.92, 132.24, 131.66, 127.06, 124.81, 120.01, 119.24, 119.00, 116.09, 106.54. MS (ESI) m/z: 297.1 [M + H]+.
5j: 212 mg, yield: 65%. White solid. 1H NMR (300 MHz, DMSO) δ 11.75 (s, 1H), 10.00 (s, 2H), 8.52 (s, 1H), 8.10 (d, J = 7.6 Hz, 1H), 7.51 (d, J = 8.2 Hz, 2H), 7.41 (d, J = 8.3 Hz, 2H), 7.27 (t, J = 7.6 Hz, 1H), 6.94 − 6.85 (m, 2H), 1.34 (s, 9H). 13C NMR (75 MHz, DMSO) δ 175.72, 156.60, 147.59, 136.54, 131.31, 127.35, 127.08, 125.33, 124.76, 120.29, 119.25, 116.06, 34.21, 31.24. MS (ESI) m/z: 328.2 [M + H]+.
5k: 187 mg, yield: 66%. White solid. 1H NMR (300 MHz, DMSO) δ 11.73 (s, 1H), 9.97 (s, 2H), 8.48 (s, 1H), 8.08 (d, J = 7.4 Hz, 1H), 7.42 − 7.37 (m, 2H), 7.24 (t, J = 7.7 Hz, 2H), 7.01 (d, J = 7.5 Hz, 1H), 6.89 − 6.81 (m, 2H), 2.32 (s, 3H). 13C NMR (75 MHz, DMSO) δ 175.62, 156.55, 140.04, 138.99, 137.24, 131.25, 127.80, 127.06, 125.97, 125.76, 122.65, 120.23, 119.19, 116.01, 20.93. MS (ESI) m/z: 286.1 [M + H]+.
5l: 211 mg, yield: 70%. White solid. 1H NMR (300 MHz, DMSO) δ 11.70 (s, 1H), 9.97 (s, 1H), 9.91 (s, 1H), 8.48 (s, 1H), 8.07 (d, J = 7.5 Hz, 1H), 7.26 − 7.20 (m, 3H), 6.90 − 6.81 (m, 3H), 2.27 (s, 6H). 13C NMR (75 MHz, DMSO) δ 175.58, 156.62, 138.93, 137.08, 131.33, 127.11, 126.67, 123.21, 120.31, 119.29, 116.08, 20.95. MS (ESI) m/z: 300.1 [M + H]+.
5m: 193 mg, yield: 57%. White solid. 1H NMR (300 MHz, DMSO) δ 11.96 (s, 1H), 10.24 (s, 1H), 10.02 (s, 1H), 8.52 (s, 1H), 8.08 (d, J = 7.3 Hz, 1H), 7.91 (d, J = 8.3 Hz, 2H), 7.72 (d, J = 8.4 Hz, 2H), 7.25 (t, J = 7.2 Hz, 1H), 6.90 − 6.83 (m, 2H). 13C NMR (75 MHz, DMSO) δ 175.50, 156.79, 142.93, 140.75, 131.61, 127.09, 126.19, 125.33, 125.20, 125.16, 125.11, 125.06, 122.59, 120.12, 119.28, 116.71, 116.11. MS (ESI) m/z: 340.1 [M + H]+.
5n: 217 mg, yield: 64%. White solid. 1H NMR (300 MHz, DMSO) δ 11.94 (s, 1H), 10.24 (s, 1H), 10.01 (s, 1H), 8.52 (s, 1H), 8.10 (d, J = 7.5 Hz, 1H), 8.04 (s, 1H), 7.95 (d, J = 7.7 Hz, 1H), 7.62 − 7.52 (m, 2H), 7.25 (t, J = 7.1 Hz, 1H), 6.91 − 6.83 (m, 2H). 13C NMR (75 MHz, DMSO) δ 175.70, 156.76, 140.65, 140.01, 131.58, 129.45, 129.09, 128.98, 128.56, 127.09, 125.97, 122.36, 121.80, 121.50, 120.17, 119.27, 116.11. MS (ESI) m/z: 340.1 [M + H]+.
5o: 119 mg, yield: 44%. White solid. 1H NMR (300 MHz, DMSO) δ 11.94 (s, 1H), 10.16 (s, 1H), 9.99 (s, 1H), 8.68 (s, 1H), 8.51 (s, 1H), 8.38 (d, J = 4.7 Hz, 1H), 8.10 (d, J = 7.7 Hz, 1H), 7.99 (d, J = 8.1 Hz, 1H), 7.40 (dd, J = 8.1, 4.8 Hz, 1H), 7.24 (t, J = 7.7 Hz, 1H), 6.90 − 6.82 (m, 2H). 13C NMR (75 MHz, DMSO) δ 176.29, 156.71, 147.23, 145.96, 140.58, 135.98, 133.41, 131.53, 127.05, 122.88, 120.18, 119.24, 116.09. MS (ESI) m/z: 273.1 [M + H]+.
5p: 132 mg, yield: 48%. White solid. 1H NMR (300 MHz, DMSO) δ 11.34 (s, 1H), 9.92 (s, 1H), 8.37 (s, 1H), 7.92 (dd, J = 16.8, 8.2 Hz, 2H), 7.22 (t, J = 7.7 Hz, 1H), 6.88 − 6.81 (m, 2H), 1.87 (d, J = 11.1 Hz, 2H), 1.72 (d, J = 12.1 Hz, 2H), 1.61 (d, J = 13.0 Hz, 1H), 1.49 − 1.11 (m, 6H). 13C NMR (75 MHz, DMSO) δ 175.61, 156.39, 139.42, 131.01, 126.67, 120.34, 119.20, 116.03, 52.49, 31.82, 25.12, 24.87. MS (ESI) m/z: 278.1 [M + H]+.
5q: 129 mg, yield: 46%. White solid. 1H NMR (300 MHz, DMSO) δ 11.33 (s, 1H), 9.88 (s, 1H), 8.42 (t, J = 5.6 Hz, 1H), 8.36 (s, 1H), 7.92 (d, J = 7.7 Hz, 1H), 7.21 (t, J = 7.7 Hz, 1H), 6.88 − 6.80 (m, 2H), 3.53 (dd, J = 13.6, 6.6 Hz, 2H), 1.59 − 1.55 (m, 2H), 1.28 (s, 6H), 0.87 (t, J = 5.9 Hz, 3H). 13C NMR (75 MHz, DMSO) δ 176.70, 156.34, 139.11, 130.96, 126.66, 120.45, 119.18, 116.03, 43.49, 31.06, 28.85, 26.03, 22.06, 13.91. MS (ESI) m/z: 280.2 [M + H]+.
5r: 287 mg, yield: 70%. White solid. 1H NMR (300 MHz, DMSO) δ 12.19 (s, 1H), 10.48 (s, 1H), 10.08 (s, 1H), 8.59 (s, 1H), 8.52 (s, 2H), 8.16 (d, J = 7.3 Hz, 1H), 7.92 (s, 1H), 7.31 (t, J = 7.8 Hz, 1H), 6.95 − 6.89 (m, 2H). 13C NMR (75 MHz, DMSO) δ 175.47, 156.91, 141.31, 141.21, 131.82, 130.46, 130.02, 129.59, 129.15, 127.05, 125.30, 125.25, 125.14, 121.53, 120.03, 119.30, 117.91, 117.76, 116.15. MS (ESI) m/z: 408.1 [M + H]+.
5s: 245 mg, yield: 60%. White solid. 1H NMR (300 MHz, DMSO) δ 12.15 (s, 1H), 10.48 (s, 1H), 9.61 (s, 1H), 8.48 (s, 2H), 8.13 (s, 1H), 7.89 (s, 1H), 7.31 − 7.22 (m, 3H), 6.86 (d, J = 7.9 Hz, 1H). 13C NMR (75 MHz, DMSO) δ 175.73, 157.61, 144.62, 141.10, 134.92, 130.39, 129.96, 129.66, 129.52, 129.08, 128.67, 125.27, 125.22, 125.06, 121.44, 119.08, 117.84, 117.80, 117.75, 117.61, 113.99. MS (ESI) m/z: 408.1 [M + H]+.
5t: 266 mg, yield: 65%. White solid. 1H NMR (300 MHz, DMSO) δ 12.05 (s, 1H), 10.38 (s, 1H), 9.99 (s, 1H), 8.51 (s, 2H), 8.12 (s, 1H), 7.87 (s, 1H), 7.75 (d, J = 8.0 Hz, 2H), 6.83 (d, J = 8.2 Hz, 2H). 13C NMR (75 MHz, DMSO) δ 175.07, 159.84, 144.74, 141.18, 130.41, 129.97, 129.74, 129.54, 129.10, 125.12, 125.08, 125.03, 124.62, 121.51, 117.64, 115.64. MS (ESI) m/z: 408.1 [M + H]+.
In vitro antifungal activity assay
The MIC values of the target compounds were tested according to the broth microdilution protocol of the Clinical and Laboratory Standards Institute (CLSI, M27-A3 and M60) Citation28,Citation29. Specific experimental operation details can be found in the supplementary material.
Biofilm formation assay
The in vitro biofilm formation assay was performed as described previouslyCitation33. Specific experimental details can be found in the supplementary material.
Cell viability and hemolysis assay
The cytotoxic effect of compounds on HUVECs viability was assessed using the cell counting kit-8 (CCK-8, Target Molecule Corp. USA) assayCitation34. The haemolytic activity of tested compounds was assessed on rabbit erythrocytes as previously reportedCitation35.The experimental details can be found in the supplementary material.
ADME/T prediction
The Swiss ADME software (www.swissadme.ch) of the Swiss Institute of Bioinformatics was accessed through a web server displaying the Swiss ADME submission page and was used to estimate individual ADME properties of compounds. The list contains one input for each molecule, as defined by the Simplified Molecular Input Line Input System (SMILES), and the results for each molecule are displayed in tables and Excel spreadsheets.
Supplemental Material
Download PDF (5.4 MB)Disclosure statement
The authors report no conflicts of interest.
Additional information
Funding
References
- Bitar D, Lortholary O, Le Strat Y, Nicolau J, Coignard B, Tattevin P, Che D, Dromer F. Population-based analysis of invasive fungal infections, France, 2001–2010. Emerg Infect Dis. 2014;20(7):1149–1155.
- Arendrup MC, Patterson TF. Multidrug-resistant candida: epidemiology, molecular mechanisms, and treatment. J Infect Dis. 2017;216(suppl_3):S445–S451.
- Barantsevich N, Barantsevich E. Diagnosis and treatment of invasive candidiasis. Antibiotics . 2022;11(6):718.
- Kumar S, Kumar A, Roudbary M, Mohammadi R, Černáková L, Rodrigues CF. Overview on the infections related to rare candida species. Pathogens. 2022;11(9):963.
- Silva S, Negri M, Henriques M, Oliveira R, Williams DW, Azeredo J. Candida glabrata, candida parapsilosis and candida tropicalis: biology, epidemiology, pathogenicity and antifungal resistance. FEMS Microbiol Rev. 2012;36(2):288–305.
- Frías-De-León MG, Hernández-Castro R, Conde-Cuevas E, García-Coronel IH, Vázquez-Aceituno VA, Soriano-Ursúa MA, Farfán-García ED, Ocharán-Hernández E, Rodríguez-Cerdeira C, Arenas R, et al. Candida glabrata antifungal resistance and virulence factors, a perfect pathogenic combination. Pharmaceutics. 2021;13(10):1529.
- Rodrigues CF, Silva S, Henriques M. Candida glabrata: a review of its features and resistance. Eur J Clin Microbiol Infect Dis. 2014;33(5):673–688.
- Colombo AL, Junior JNA, Guinea J. Emerging multidrug-resistant candida species. Curr Opin Infect Dis. 2017;30(6):528–538.
- Csank C, Haynes K. Candida glabrata displays pseudohyphal growth. FEMS Microbiol Lett. 2000;189(1):115–120.
- Zheng Q, Liu J, Qin J, Wang B, Bing J, Du H, Li M, Yu F, Huang G. Ploidy variation and spontaneous haploid-diploid switching of candida glabrata clinical isolates. mSphere 2022:e0026022.
- Robbins N, Caplan T, Cowen LE. Molecular evolution of antifungal drug resistance. Annu Rev Microbiol. 2017;71:753–775.
- Lee Y, Puumala E, Robbins N, Cowen LE. Antifungal drug resistance: molecular mechanisms in candida albicans and beyond. Chem Rev. 2021;121(6):3390–3411.
- Arendrup MC, Perlin DS. Echinocandin resistance: an emerging clinical problem? Curr Opin Infect Dis. 2014;27(6):484–492.
- Lee A. Ibrexafungerp: first approval. Drugs. 2021;81(12):1445–1450.
- Li L, Wu H, Zhu S, Ji Z, Chi X, Xie F, Hao Y, Lu H, Yang F, Yan L, et al. Discovery of novel 7-hydroxy-5-oxo-4,5-dihydrothieno[3,2-b]pyridine-6-carboxamide derivatives with potent and selective antifungal activity against cryptococcus species. J Med Chem. 2022;65(16):11257–11269.
- Lv Q, Ni T, Li L, Li T, Zhang D, Jiang Y. A new antifungal agent (4-phenyl-1, 3-thiazol-2-yl) hydrazine induces oxidative damage in candida albicans. Front Cell Infect Microbiol. 2020;10:578956.
- Ni T, Xie F, Li L, Hao Y, Chi X, Yan L, Zhang D, Jiang Y, Lv Q. Design, synthesis and structure-activity relationship studies of nicotinamide derivatives as potent antifungal agents by disrupting cell wall. Molecules. 2023;28(3):1135.
- Wang Y, Gu W, Shan Y, Liu F, Xu X, Yang Y, Zhang Q, Zhang Y, Kuang H, Wang Z, et al. Design, synthesis and anticancer activity of novel nopinone-based thiosemicarbazone derivatives. Bioorg Med Chem Lett. 2017;27(11):2360–2363.
- Bal TR, Anand B, Yogeeswari P, Sriram D. Synthesis and evaluation of anti-hiv activity of isatin beta-thiosemicarbazone derivatives. Bioorg Med Chem Lett. 2005;15(20):4451–4455.
- Liu L, Xu YS, Chigan JZ, Zhai L, Ding HH, Wu XR, Chen WY, Yang KW. Dihydroxyphenyl-substituted thiosemicarbazone: a potent scaffold for the development of metallo-beta-lactamases inhibitors and antimicrobial. Bioorg Chem. 2022;127:105928.
- Muğlu H, Çavuş MS, Bakır TK, Yakan H. Synthesis of new bis(thiosemicarbazone) derivatives and dft analysis of antioxidant characteristics in relation to hat and set reactions. J Indian Chem Soc. 2022;99(12):100789.
- Rahim F, Ullah H, Taha M, Hussain R, Sarfraz M, Iqbal R, Iqbal N, Khan S, Ali Shah SA, Albalawi MA, et al. Synthesis of new triazole-based thiosemicarbazone derivatives as anti-alzheimer’s disease candidates: evidence-based in vitro study. Molecules. 2023;28(1):21.
- Singh P, Rawat S, Agrahari AK, Singh M, Chugh S, Gurcha S, Singh A, Abrahams K, Besra GS, Asthana S, et al. Nsc19723, a thiacetazone-like benzaldehyde thiosemicarbazone improves the efficacy of tb drugs in vitro and in vivo. Microbiol Spectr. 2022;10(6):e02592–22.
- de Oliveira RB, de Souza-Fagundes EM, Soares RP, Andrade AA, Krettli AU, Zani CL. Synthesis and antimalarial activity of semicarbazone and thiosemicarbazone derivatives. Eur J Med Chem. 2008;43(9):1983–1988.
- de Araujo Neto LN, de Lima M, de Oliveira JF, de Souza ER, Feitosa Machado SE, de Souza Lima GM, Silva Buonafina MD, Brayner FA, Alves LC, Sandes JM, et al. Thiophene-thiosemicarbazone derivative (l10) exerts antifungal activity mediated by oxidative stress and apoptosis in c. Albicans. Chem Biol Interact. 2020;320:109028.
- Baell JB, Ferrins L, Falk H, Nikolakopoulos G. Pains: relevance to tool compound discovery and fragment-based screening. Aust J Chem. 2013;66(12):1483–1494.
- Baell JB, Holloway GA. New substructure filters for removal of pan assay interference compounds (pains) from screening libraries and for their exclusion in bioassays. J Med Chem. 2010;53(7):2719–2740.
- CLSI. Reference method for broth dilution antifungal suscepti bility testing of yeasts, m27-a3, third ed., Wayne: Clinical and Laboratory Standards Institute pa 2009.
- CLSI. Performance standards for antifungal susceptibility testing of yeast, m60, first ed., Wayne: Clinical and Laboratory Standards Institute, pa. 2017.
- Widiasih Widiyanto T, Chen X, Iwatani S, Chibana H, Kajiwara S. Role of major facilitator superfamily transporter qdr2p in biofilm formation by candida glabrata. Mycoses. 2019;62(12):1154–1163.
- Fidel PL, Jr., Vazquez JA, Sobel JD. Candida glabrata: review of epidemiology, pathogenesis, and clinical disease with comparison to c. Albicans. Clin Microbiol Rev. 1999;12(1):80–96.
- Daina A, Michielin O, Zoete V. Swissadme: a free web tool to evaluate pharmacokinetics, drug-likeness and medicinal chemistry friendliness of small molecules. Sci Rep. 2017;7:42717.
- Li DD, Zhao LX, Mylonakis E, Hu GH, Zou Y, Huang TK, Yan L, Wang Y, Jiang YY. In vitro and in vivo activities of pterostilbene against candida albicans biofilms. Antimicrob Agents Chemother. 2014;58(4):2344–2355.
- Lu R, Ni T, Wu J, Yan L, Lv Q, Li L, Zhang D, Jiang Y. New triazole nt-a9 has potent antifungal efficacy against cryptococcus neoformans in vitro and in vivo. Antimicrob Agents Chemother. 2020;64(2):e01628-01619.
- Li L, Zhang T, Xu J, Wu J, Wang Y, Qiu X, Zhang Y, Hou W, Yan L, An M, et al. The synergism of the small molecule enoblock and fluconazole against fluconazole-resistant candida albicans. Front Microbiol. 2019;10(:2071.