Abstract
Curcuminoids are functional food additives, and the effect on gonadal hormone biosynthesis remains unclear. Gonads contain 3β-hydroxysteroid dehydrogenase isoforms, h3β-HSD2 (humans) and r3β-HSD1 (rats), which catalyse pregnenolone into progesterone. The potency and mechanisms of curcuminoids to inhibit 3β-HSD activity were explored. The inhibitory potency was bisdemethoxycurcumin (IC50, 1.68 µM) >demethoxycurcumin (3.27 µM) > curcumin (13.87 µM) > tetrahydrocurcumin (109.0 µM) > dihydrocurcumin and octahydrocurcumin on KGN cell h3β-HSD2, while that was bisdemethoxycurcumin (1.22 µM) >demethoxycurcumin (2.18 µM) > curcumin (4.12 µM) > tetrahydrocurcumin (102.61 µM) > dihydrocurcumin and octahydrocurcumin on testicular r3β-HSD1. All curcuminoids inhibited progesterone secretion by KGN cells under basal and forskolin-stimulated conditions at >10 µM. Docking analysis showed that curcuminoids bind steroid-active site with mixed or competitive mode. In conclusion, curcuminoids inhibit gonadal 3β-HSD activity and de-methoxylation of curcumin increases inhibitory potency and metabolism of curcumin by saturation of carbon chain losses inhibitory potency.
Introduction
In Asia and Africa, Curcuma (turmeric), the rhizome of the perennial herb Curcuma longa L., is widely used as functional food additive to humans and animals. Since the last century, the main bioactive component of turmeric has been identified as curcuminoids. The major component of curcuminoids is curcumin (), a beta-diketone polyphenol that is methane in which two of the hydrogens are substituted by feruloyl groups. Curcuminoids isolated from turmeric usually contain 65% curcumin (CUR) and 35% de-methoxylated curcumin: 25% demethoxycurcumin (DMC) that has one de-methoxylated group in one of benzene rings and 10% bisdemethoxycurcumin (BDMC) that has two de-methoxylated groups in two benzene rings ()Citation1. CUR in plants and animals can be metabolised into dihydrocurcumin (DHC) after one of the double bonds is reduced, or tetrahydrocurcumin (THC) after two double bonds are reduced by gut microbiota, and octahydrocurcumin (OHC) after all double bonds in the bridge moiety are reduced. Curcuminoids have been found to have anti-inflammatory, anti-oxidative, anti-malarial, anti-septic, anti-tumour, analgesic, and anti-virus COVID-19 activityCitation2–6. DMC retains some of the beneficial properties of curcumin, including its antioxidant and anti-inflammatory effectsCitation7. BDMC has been reported to have anticancer effects in several cancer cell lines and animal modelsCitation8.
Figure 1. Chemical structures of curcumin analogues and reaction of 3β-hydroxysteroid dehydrogenase (3β-HSD): Structures of curcumin analogues (A); (B) Gonadal 3β-HSD catalyses pregnenolone (P5) to progesterone (P4).
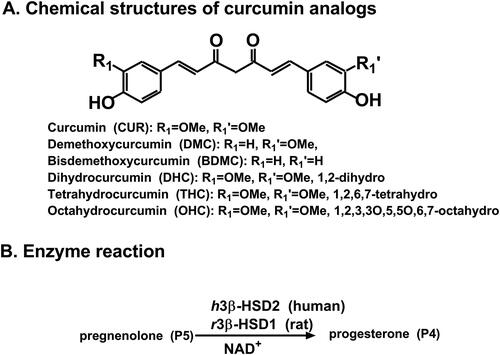
Adrenal and gonadal 3β-hydroxysteroid dehydrogenase/Δ5,4-isomerases (3β-HSDs) are hydroxysteroid dehydrogenase subfamily members that play critical role in the second step catalysis for adrenal glucocorticoid and mineralocorticoid and gonadal sex steroid biosynthesis. They catalyse the conversion from pregnenolone (P5) or dehydroepiandrosterone (DHEA) to progesterone (P4) or androstenedione (), which are precursors of potent glucocorticoids, mineralocorticoids, androgens, and oestrogensCitation9. Two isoforms of 3β-HSD have been cloned in humans, type 1 3β-HSD1 (h3β-HSD1) and type 2 (h3β-HSD2), and h3β-HSD2 is exclusively present in adrenals and gonads for its function. In the rat model, 4 isoforms have been cloned, rat type 1 (r3β-HSD1) is primarily present in gonadal cells (including testicular Leydig cells)Citation9.
Adrenal and gonadal 3β-HSD isoform has been targeted for drug development to treat Cushing’s syndrome. For example, 3β-HSD2 inhibitor trilostane has been used as a drug to treat Cushing’s syndromes in pets and animalsCitation10–12. Human h3β-HSD2 and r3β-HSD1 belong to the short-chain alcohol dehydrogenase superfamilyCitation9 and are nicotinamide adenine dinucleotide (NAD)+-dependent enzymes, with the catalytic motif of “Ser/Thr…Tyr-X-X-X-Lys”Citation9. They catalyses two steps of reactions, first using P5 as substrate to perform 3β-dehydrogenation reaction and then using P5’s intermediate as the substrate and reduced NAD (NADH) to carry out Δ5,4-isomerationCitation9.
Curcuminoids can inhibit several steroid synthases, thereby regulating the steroid hormone levels. In this case, CUR was found to be a potent inhibitor of human 11β-hydroxysteroid dehydrogenase 1 and 2, with half maximal inhibitory concentration (IC50) values about 10–15 µMCitation13, thereby regulating glucocorticoid levels. In the testes, CUR is a potent inhibitor of testosterone synthetase 17β-hydroxysteroid dehydrogenase 3 with an IC50 over 50 µMCitation14. Whether curcumin analogues directly inhibit h3β-HSD2 and rat 3β-HSD1 isoforms, which serve targets to treat Cushing’s syndrome in animals and humans, is still unclear. In the current study, 6 curcumin analogues (CUR, DMC, and BDMC, DHC, THC, and OHC) were investigated for their potency, mode of action, structure-activity relationship (SAR) and species difference.
Materials and methods
Chemicals and animals
Dimethyl sulfoxide (DMSO), P5, P4, phosphorylated buffered saline (PBS, pH 7.2), NAD+, and NADH were purchased from Sigma-Aldrich (St. Louis, MO). CUR (CAS# 458–37-7, Cat# CS1490, purity 100%) was obtained from MCE Standard (Shanghai, China), DMC (CAS# 22608–11-3, Cat# D860706, purity 98%) from Maclin (Shanghai, China), BDMC (CAS# 33171–05-0, Cat# KP4QZWDK, purity 99.27%) from Picassoe (Shanghai, China), DHC (CAS# 76474–56-1, Cat# PA41067, purity 98%), THC (CAS# 36062–04-1, Cat# PN0122, purity 98%), and OHC (CAS# 36062–07-4, Cat# PA72348, purity 98%) from Perfemiker (Shanghai, China). DMEM/F12 medium, penicillin/streptomycin, and foetal bovine serum (FBS) were purchased from Gibco (ThermoFisher Scientific, Shanghai, China). Male Sprague-Dawley rats (age of 90 days) were obtained from Shanghai Laboratory Animal Centre (Shanghai, China) for harvesting testes after CO2 euthanization under approval by the Experimental Animal Ethics Committee of Wenzhou Medical University. Human gonadal granulosa cell KGN cell line was obtained from ATCC (USA) for source of h3β-HSD2, and the h3β-HSD2 expression in KGN cells can be upregulated by 3–5 fold after cAMP (or forskolin) manipulationCitation15,Citation16.
Preparation of rat testicular microsome
Rat testicular microsome as the r3β-HSD1 source was prepared as previously describedCitation17. Briefly, the microsome was prepared after sequential centrifugations of aqueous phase in sucrose (0.25 M)-containing PBS (pH 7.2, 0.01 M) brought by homogenisation of rat testis at 700 ×g for 30 min, 14,500 ×g for 30 min, and 105,000 ×g twice for 60 min. Enhanced BCA protein assay kit (Cat#P0010, Beyotime Biotech Inc, Shanghai, China) was used to measure microsomal protein content.
KGN cell culture
KGN cells were cultured as previously describedCitation15,Citation16. In brief, KGNs were maintained in DMEM/F12 medium containing 10% FBS and 1% antibiotics. After cells were seeded in 75-cm2 culture flasks for growth at 37 °C and 5% CO2 up to 80% confluence, cells were treated with 10 µM FSK for 24 h, and then cells were harvested. Some cells were homogenised to obtain KGN homogenate as the h3β-HSD2 source. Some KGN cells (1 × 106 cells/well) were seeded in the 24-well plate and treated with 0, 10, 20, and 40 µM curcumin analogues in the presence of 10 µM FSK (FSK-stimulated condition) or without FSK (the basal condition) for 24 h. The medium was harvested and P4 content in the medium was determined using HPLC/MS-MS.
3β-HSD activity quantitation
Enzymatic activity for 3β-HSD was determined as describedCitation18. The following experiments were designed: ① Time curve of reaction: 0.2 µM P5, 0.2 mM NAD+, 50 µg KGN cell homogenate or 5 µg rat testicular microsome in a 100 µL PBS (pH 7.2) were incubated for 0, 15, 30, 60, and 120 min; ② Michaelis-Menten constant (Km) and Vmax: 0–12 µM P5, 0.2 mM NAD+ in a 100 µL PBS were incubated for 30 min; ③ Effect of 100 µM curcumin analogues and CUR metabolites on 3β-HSD: 0.2 µM P5, 0.2 mM NAD+, 100 µM chemical, 50 µg KGN cell homogenate or 5 µg rat testicular microsome in a 100 µL PBS were incubated for 30 min; ④ IC50 value: 0.2 µM P5, 0.2 mM NAD+, 0.01–200 µM chemical, 50 µg KGN cell homogenate or 5 µg rat testicular microsome in a 100 µL PBS were incubated for 30 min; ⑤ mode of action vs. P5: 0–20.0 µM P5, 0.2 mM NAD+, 0–20 µM chemical, 50 µg KGN cell homogenate or 5 µg rat testicular microsome in a 100 µL PBS were incubated for 30 min. The assay was done in pH 7.2 PBS. DMSO was used as vehicle to dissolve curcumin analogues. At the end of reaction, we added 10 µL of internal standard (IS, testosterone-d5, T-d5, Shanghai Zzbio, China) and 200 µL of acetonitrile (Merck Supelco, PA) to extract steroids and injected organic layer (10 µL) onto the HPLC-MS/MS system for measurement of P4.
HPLC-MS-MS
HPLC-MS-MS quantification of P4 was performed in an Acquity UPLC instrument (Waters, USA) with Acquity BEH C18 column (2.1 mm × 50 mm, 1.7 µm particle size) and a 0.2 µm stainless steel block filter as previously describedCitation19. We set flow rate of 0.4 µL/min and column temperature of 30 °C. A XEVO TQD triple quadrupole mass spectrometer equipped with an electrospray ionisation source (Waters, USA) was used for mass detection with MRM mode transitions of P4 and IS as m/z 315.16→96.92 and m/z 289.07→96.92, respectively. Masslynx 4.1 software (Waters, USA) was used to collect data, which was exported for analysis of P4 using the standard curve method.
Enzymatic parameter analysis
Enzyme parameter analysis was done by GraphPad (version 8.0, GraphPad Software, San Diego, CA, USA) as previously describedCitation18. ① Time curve of reaction: linear regression; ② Michaelis-Menten constant (Km) and Vmax was calculated by a nonlinear regression using equation: V = Vmax*[P5]/(Km + [P5]), where V is velocity (pmol/mg/min) and [P5] is substrate concentration (µM); ③ Effect of 100 µM curcumin analogues on 3β-HSD: Residual activity related to DMSO control (100%) was calculated; ④ IC50 value was calculated by a nonlinear regression of [inhibitor] vs. response (three parameters); ⑤ mode of action vs. P5 was determined by a nonlinear regression in enzyme kinetics – inhibition (mixed model) for parameters of “α” and Ki by following equations: V = VmaxApp*[P5]/(KmApp + [P5]), where [P5] was substrate concentration, V was the enzyme activity; and VmaxApp and KmApp were derived from VmaxApp = Vmax/(1 + I/(α*Ki)) and KmApp = Km*(1 + I/Ki)/(1 + I/(α*Ki)), where I was inhibitor’s concentration; “α” was determination factor for mode of action. When “α = 1”, it was a non-competitive inhibitor; when “α > 1 or <1", it was a mixed inhibitor; when “α was very small but larger than zero", it was an uncompetitive inhibitor; when “α was very large", it was a competitive inhibitor. ⑥ Lineweaver-Burk plot was drawn using linear regression.
Molecular simulation analysis
The ligand structures of mol2 file were converted from the SDF files from the PubChem (https://pubchem.ncbi.nlm.nih.gov/) by PyMOL software. We searched the PDB dataset for h3β-HSD2 and r3β-HSD1 crystal structures and did not find any. Therefore, the 3D AlphaFold2-generated models were used for molecular simulation analysis as describedCitation20,Citation21. 3D AlphaFold2-generated models included AF-P26439-F-model_v4 (h3β-HSD2) with 94.7% of Average pLDDT credibility and AF-P22071-F1-model_v4 (r3β-HSD1) with 94.66% credibility. The protein structures of h3β-HSD2 and r3β-HSD1 were optimised as previously describedCitation22,Citation23. ProCheck software was used to verify the anticipated 3D structures by creating the Ramachandran plotCitation23. These protein models were docked with each chemical by Autodock 4.0 (Scripps Research Institute, http://autodock.scripps.edu) using a genetic algorithm with local search, and a ranking clustering method for selection and the configuration of the ligand with the lowest binding energy (LBE). P5 and NAD+ and each curcuminoid were docked with these 3D structures. In the Autodock4, we encompassed the entire structure of h3β-HSD2 and set searching grid box to 126 × 126 × 126 with the grid position (x, y, and z) at 0.223, 0.289, and 0.104 for CUR (cluster RMSD = 0, reference RMSD = 4.72), 0.470, −0.446, and 0.186 for DMC (cluster RMSD = 0, reference RMSD = 5.19), −0.006, −0.238, and 0.006 for BDMC (cluster RMSD = 0, reference RMSD = 5.44), and 1.557, −0.589, and −0.675 for THC (cluster RMSD = 1.43, reference RMSD = 5.78), and a spacing of 0.375 Å. We encompassed the entire structure of r3β-HSD1 and set searching grid box to 126 × 126 × 126 with the grid position (x, y, and z) at 1.752, −1.080, and −0.299 for CUR (cluster RMSD = 0, reference RMSD = 6.14), 0.470, −0.446, 0.186 for DMC (cluster RMSD = 0, reference RMSD = 5.34), −0.006, −0.238, and 0.006 for BDMC (cluster RMSD = 0, reference RMSD = 4.81), and 1.752, −1.080, and −0.299 for THC (cluster RMSD = 0, reference RMSD = 4.37), and a spacing of 0.375 Å. Docking structure was visualised by PyMOL software and exported to PDB for 2D superimposed image used by LigPlot Citation24 and for interaction (hydrophobic, hydrogen bond or HB, halogen bond, and π stacking) using Protein-Ligand Interaction Profiler web server as describedCitation25. Molecular docking data of contacting residues, hydrogen bonds, hydrophobic interactions, and overlapping residues were shown in Table S1.
Analysis of the molecular structure of curcumin analogues
A free-searchable database of ZINCCitation26, which includes the chemical properties: molecular weight (MW, d), apolar desolvation energy (AD, kcal/mol), polar desolvation energy (PD, kcal/mol), number of hydrogen bond donors (HBDs), number of hydrogen bond acceptors (HBAs), topological polar surface area (PSA, Å2), and total HB (HBD + HBA). The pKa and partition coefficient (LogP) were acquired from GuideChem (https://www.guidechem.com/product/a-p1.html).
Statistics
Data were shown as mean ± SEM (n = 4–8 measurements). Significant differences between the curcuminoid and control were examined by one-way ANOVA followed by post hoc Dunnett’s multiple comparisons. Bivariate analyses were done using Pearson correlations to measure the strength of the relationship between compounds’ properties and IC50 values. Differences were regarded as statistically significant at *p < 0.05, **p < 0.01, ***p < 0.001.
Results
Effect of curcumin analogues on human 3β-HSD2 activity
With NAD+ cofactor, h3β-HSD2 in KGN cells was determined by conversing P5 to P4. Michaelis-Menten kinetics revealed that apparent Km of h3β-HSD2 was 3.08 ± 0.81 µM and Vmax was 18.88 ± 1.86 pmol/mg/min (). The apparent Km value in this study was in consistency with the reported Km valueCitation27. Six curcumin analogues were screened for their inhibition of h3β-HSD2 activity at a concentration of 100 µM. The results showed that CUR, DMC, and BDMC significantly reduced h3β-HSD2 activity to <25% of the control, indicating that their IC50 values were <100 µM. THC, DHC, and OHC significantly reduced the enzyme activity, leading to the residual activity to ≥50% of the control, indicating their IC50 values were ≥100 µM (). IC50 values were calculated for CUR, DMC, BDMC, and THC, and their IC50 values were 13.87 ± 2.12, 3.27 ± 0.38, 1.68 ± 0.39, and 109.0 ± 19.20 µM ( and ), respectively. Ki values of CUR, DMC, and BDMC were determined, and results showed that Ki values were 15.28, 3.85, and 1.12 µM, respectively (. These results indicate that the inhibitory potency of curcumin analogues on h3β-HSD2 is BDMC > DMC > CUR > THC and that the de-methoxylation of CUR increases the inhibitory potency while CUR metabolites lose inhibitory effect after the carbon-chain double bond is reduced. We further determined mode of action of curcumin analogues using enzyme kinetics-inhibition (mixed model) and Lineweaver-Burk plot, and found that CUR and DMC were mixed inhibitors while BDMC was a competitive inhibitor (.
Figure 2. Michaelis-Menten kinetics, residual activity and dose response of curcumin analogues on human 3β-HSD2: Michaelis-Menton kinetics (A); Residual activity relative to the control (DMSO) at 100 µM curcumin analogues (B): Compared with the control, ** p < 0.01, *** p < 0.001 (n = 4, mean ± SEM); IC50 value of curcumin (CUR), demethoxycurcumin (DMC) and bisdemethoxycurcumin (BDMC), and tetrahydrocurcumin (THC) (C-F: n = 4, mean ± SEM).
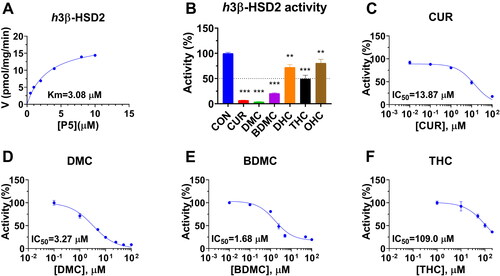
Figure 3. Enzyme kinetics-inhibition and Lineweaver-Burk plot analysis of curcumin analogues on human 3β-HSD2: Enzyme kinetics-inhibition analysis for curcumin (CUR), demethoxycurcumin (DMC) and bisdemethoxycurcumin (BDMC) (A-C); Lineweaver-Burk plot analysis for CUR, DMC, and BDMC (D-F), n = 4.
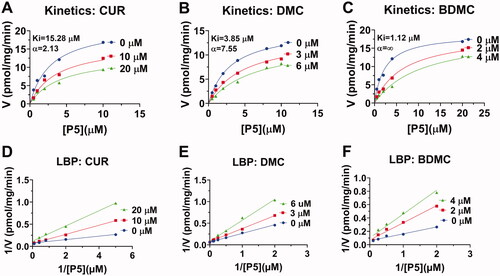
Table 1. Half maximal inhibitory concentration (IC50), inhibition constant (measured, Ki), inhibition Ki (calculated, Cal. Ki), lowest binding energy (LBE), mode action, and binding domain of curcuminoids.
Effect of curcumin analogues on P4 output in human KGN cells
To test whether curcumin analogues can repress h3β-HSD2, thereby reducing P4 secretion, human KGN cells that express h3β-HSD2 and respond to cAMP stimulationCitation15 were used. P4 amount under basal and cAMP-stimulated (10 µM FSK) conditions were measured. DMC and BDMC markedly decreased basal P4 output as low as 10 µM while CUR reduced its production at 20 and 40 µM. All curcumin analogues markedly lowered cAMP-stimulated P4 output at 40 µM (. These results indicate that curcumin analogues can repress h3β-HSD2 activity, thereby resulting in lower P4 output.
Figure 4. Effect of curcumin analogues on progesterone output by human KGN cells under basal and cAMP (forskolin, FSK, 10 µM) conditions: Progesterone output under basal condition for curcumin (CUR), demethoxycurcumin (DMC) and bisdemethoxycurcumin (BDMC) (A, C, E); Progesterone output under FSK-stimulated condition for CUR, DMC, and BDMC (B, D, F): Compared with the control, *p < 0.05, **p < 0.01, ***p < 0.001 (n = 4, mean ± SEM) for each concentration.
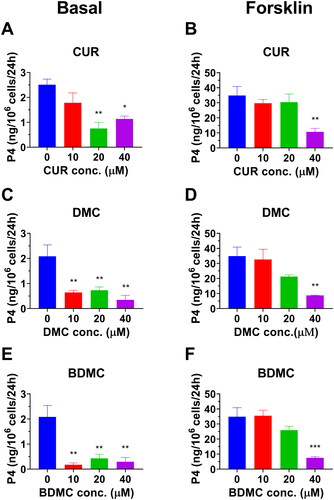
Effect of curcumin analogues on r3β-HSD1 activity
With NAD+ cofactor, r3β-HSD1 in rat testicular microsomes was determined by measuring the conversion of P5 to P4. Michaelis-Menten kinetics analysis revealed that the apparent Km of r3β-HSD1 was 0.32 ± 0.1 µM and Vmax was 12.01 ± 0.59 pmol/mg/min (). The apparent Km value in this study was in consistency with the reported high-affinity Km valueCitation9. Six curcumin analogues were screened for their inhibition of r3β-HSD1 activity at 100 µM. The results showed that CUR, DMC, and BDMC significantly reduced r3β-HSD1 activity to <20% of the control, indicating that their IC50 values were <100 µM. DHC and THC also significantly reduced the enzyme activity, leading to the residual activity to around 50% of the control, indicating their IC50 values were about or over 100 µM (). IC50 values were calculated for CUR, DMC, BDMC, and THC, and their IC50 values were 4.06 ± 1.10, 2.15 ± 0.27, 1.22 ± 0.31, and 102.61 ± 11.65 µM ( and ), respectively. Ki values of CUR, DMC, and BDMC were determined, and the results showed that Ki values were 4.21, 2.18, and 1.56 µM, respectively (. The results indicate that the inhibitory potency of curcumin analogues on r3β-HSD1 is BDMC > DMC > CUR > DHC = THC > OHC and that the de-methoxylation of CUR increases the inhibitory potency while CUR metabolites after the reduction of carbon chain double bond lose the inhibitory potency. We further decided mode of action of these chemicals using enzyme kinetics-inhibition (mixed model) and Lineweaver-Burk plot and found that CUR, DMC, and BDMC were mixed inhibitors (.
Figure 5. Michaelis-Menten kinetics, residual activity and dose response of curcumin analogues on rat 3β-HSD1: Michaelis-Menton kinetics (A); Residual activity relative to the control (DMSO) at 100 µM curcumin analogues (B): Compared with the control, **p < 0.01, ***p < 0.001 (n = 4, mean ± SEM); IC50 value of curcumin (CUR), demethoxycurcumin (DMC) and bisdemethoxycurcumin (BDMC), and tetrahydrocurcumin (THC) (C-F: n = 4, mean ± SEM).
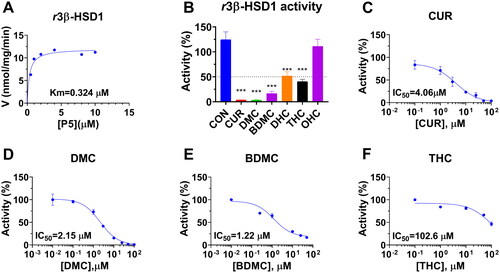
In silico docking of curcumin analogues with h3β-HSD2
No crystal structure of h3β-HSD2 is available. Therefore, a constructed 3D model (AF-P26439-F1-model_v4) was selected for docking. We used ProCheck software to perform a Ramachandran plot analysis, and found that 100% of residues fell inside the favoured (93.4%), the additionally allowed (6.3%) and generously (0.1%) regions, whereas no residues fell within the disallowed region for h3β-HSD2 (Figure S1(A)). Thomas et al. previously reported that h3β-HSD2 has three catalytic residues Ser124 and Tyr154, and Kys158 27. Substrate P5 was docked with the enzyme, and P5 contacts 10 residues, including Ser124 and Tyr154 (), forms a HB with Ser124 and Tyr154, has hydrophobic interactions with residues Val87, Ile125, Glu126, Tyr188, Phe196, Tyr264, and Leu321 (Figure S2(A)), confirming the established modelCitation27. Docking analysis revealed that CUR, DMC, and BDMC bind to steroid binding cavity and contact catalytic residues Ser124 and/or Tyr154. CUR overlaps nine P5 contact residues, forms 1 HB with catalytic residue Ser124 of h3β-HSD2, has hydrophobic interactions with residues Leu13, Ile85, Val87, Tyr188, Ile189, Phe196, Leu321, and π-stacking interaction with Phe196 and Tyr264 (RMSD = Figure S2(B)), and LBE is −6.68 kcal/mol (, and Table S1). CUR also overlaps 7 NAD+ binding residues (Figure S3), supporting its mixed inhibition. DMC overlaps 8 P5 contact residues, forms 2 HBs with residues Ser123 and Tyr264 and has hydrophobic interactions with residues Ile85, Val87, Phe88, Pro186, Tyr188, Ile189, Tyr264, and Leu321 of h3β-HSD2 (Figure S2C), and LBE is −7.51 kcal/mol (, and Table S1). DMC also overlaps 7 NAD+ binding residues (Figure S3), supporting its mixed inhibition. BDMC overlaps 9 P5 contact residues, forms 2 HBs with residues Thr81 and Ile189 of h3β-HSD2, has hydrophobic interaction with residues Ile85, Val87, Tyr188, Ile189, Tyr264, Leu321 and a π-stacking interaction with Phe196 (Figure S2(D)), and LBE was −8.04 kcal/mol (, and Table S1). THC only contacts 6 P5 contact residues (Figure S4(A,B)) and has LBE of −5.26 kcal/mol, indicating that THC has low affinity to h3β-HSD2.
Figure 7. Molecular docking of curcumin (CUR), demethoxycurcumin (DMC) and bisdemethoxycurcumin (BDMC) with human 3β-HSD2: 3D model (cyan, A) and 2 D model (purple, B) of P5 with h3β-HSD2; 3D superimposed image of CUR (C) DMC (E), BDMC (G): curcuminoid (red) occupies P5 (cyan) binding site; 2D superimposed image of CUR (D), DMC (F), BDMC (H): each curcuminoid (purple) overlaps P5 (cyan) contact residues (red circle) with the hydrogen bond (dotted line).
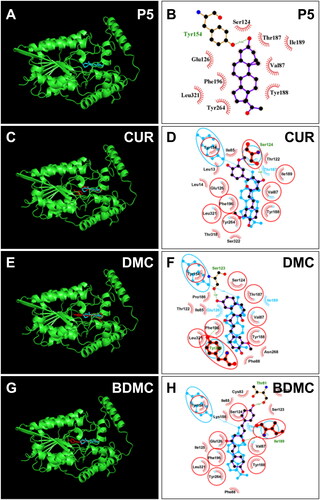
In silico docking of curcumin analogues with r3β-HSD1
No crystal structure of r3β-HSD1 is available. Therefore, a constructed 3D model for r3β-HSD1 (AF-P22071-F1-model_v4) was used. We used ProCheck to perform a Ramachandran plot analysis, and found that 100% of residues fell inside the favoured (94 percent) and the additionally allowed (6 percent) regions (Figure S1(B)). Homolog analysis showed that Thr125, Tyr155, and Lys159 are catalytic residuesCitation9. The docking of P5 with r3β-HSD1 showed that 3β-hydroxyl group of P5 forms HBs with catalytic residues Thr125 (3.01 Å) and Tyr155 (3.23 Å) and C20-hydroxyl group forms a HB with residue Thr215 (3.24 Å) () and P5 contacts nine residues and has hydrophobic interactions with residues Val189, Phe197, Tyr265, Leu319, Leu322 (Figure S2(C). Docking analysis revealed that CUR, DMC, and BDMC bind steroid binding site and contact catalytic residues Thr125 and/or Tyr155. CUR overlaps 5 P5-contact residues (), has hydrophobic interactions with residues Val15, Ala84, Ile86, Tyr189, Ile190, Tyr265, Leu322, and π-Stacking (perpendicular) with Tyr189 (Figure S2(F)), and LBE is −7.33 kcal/mol (). DMC overlaps 6 P5-contact residues, forms 2 HBs with residues Thr82 and Ile190 of r3β-HSD1 (), has hydrophobic interactions with residues of Ile86, Val88, Tyr189, Ile190, Phe197, Leu322 (Figure S2(G)), and LBE is −7.60 kcal/mol (). BDMC overlaps 12 P5-contact residues, forms 4 HBs with residues Tyr189, Ser124, Thr215, and Tyr265 of r3β-HSD1 (), has a hydrophobic interactions with Ile86, Val88, Tyr189, Tyr197, and Leu322 (Figure S2(H)), and LBE was −7.87 kcal/mol (). THC only contacts 3 P5 contact residues with LBE of −5.37 kcal/mol and a predicted Ki of 115.27 µM (Figure S4(B)), indicating that it has a low affinity to bind r3β-HSD1.
Figure 8. Molecular docking of curcumin (CUR), demethoxycurcumin (DMC) and bisdemethoxycurcumin (BDMC) with rat 3β-HSD1: 3D model (cyan, A) and 2 D model (purple, B) of P5 with r3β-HSD1; 3D superimposed image of CUR (C) DMC (E), BDMC (G): curcuminoid (red) occupies P5 (cyan) binding site; 2D superimposed image of CUR (D), DMC (F), BDMC (H): each curcuminoid (purple) overlaps P5 (cyan) contact residues (red circle) with the hydrogen bond (dotted line).
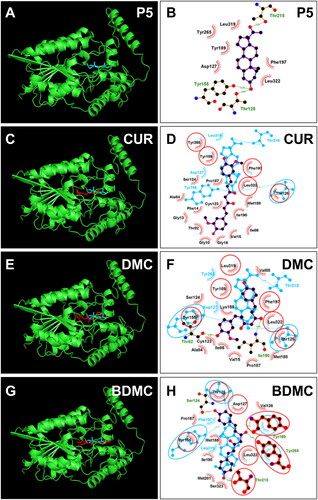
SAR analysis of curcumin analogues with h3β-HSD2 and r3β-HSD1
We analysed which structural determinants of curcumin analogues were associated with observed IC50 values for both enzymes. The following molecular determinants including MW, LogP, AD, PD HBD + HBA, PSA, pKa, and LBE (from docking) were analysed. The most evident positive correlation was found for LogP, pKa, AD, and LBE values (the higher LogP, pKa, AD, and LBE, the higher IC50, and, thus, less inhibitory potency) (. Other features including PD, HB, MW, and PSA were not associated with IC50 values (Figure S5).
Discussion
In the current study, we have proved that curcumin analogues (CUR, DMC and BDMC) were effective inhibitors of h3β-HSD2 and r3β-HSD1. DMC and BDMC inhibited h3β-HSD2 with 3 and 15 times more effectively than CUR, indicating that the increase in de-methoxylation of CUR enhances the inhibitory potency for h3β-HSD2. Interestingly, all CUR metabolites DHC, THC and OHC had weak or almost no inhibitory effect, and the reduction of unsaturated double bonds led to loss of inhibitory effects of these metabolites.
Curcuminoids isolated from traditional Chinese herb turmeric usually have 65% CUR, 25% DMC, and 10% BDMC. De-methoxylation for one methoxyl group as in DMC markedly increased its inhibitory potency by about 4-fold on h3β-HSD2 (). Further de-methoxylation for additional methoxyl group as in BDMC further increased its inhibitory potency about 12 fold. However, the de-methoxylation for effect on r3β-HSD1 was much weaker. CUR itself had higher potency to inhibit r3β-HSD1 than h3β-HSD2 by about 3 fold. De-methoxylation for one methoxyl group as in DMC only slightly enhanced its inhibitory effect by 1 fold, and further de-methoxylation for another methoxyl groups as in BDMC can increase its inhibitory effect by 2-fold ().
LogP (a parameter for lipophilicity is widely used in SAR analysesCitation28. In the present study, the bivariate analyses showed that higher LogP values were correlated with lower inhibitory activity (. Low LogP could reflect a lower number of methoxyl groups (the fewer methoxyl group, the lower LogP value), which possibly confer stronger inhibitory effects, because the de-methoxylation leads to the 4-hydroxyl group to form HBs with the enzyme. It was true that DMC and BDMC also potently inhibited human 3β-HSD1Citation29. In addition, the reduction of double bond as in DHC (LogP 3.772 vs. LogP 3.37 in CUR) and THC (LogP 3.691) confers hydrophobicity to the molecules (high LogP values), resulting in weaker inhibition. For the pKa value, lower pKa values were generally associated with stronger inhibitory potency on these two enzymes. These data indicate that the de-methoxylation of CUR as in DMC (3-position) or BDMC (3, 3′-position) tends to dissociate more easily for 4-hydroxyl group and increases its reactivity. In addition, reduction of double bond could lead to higher pKa, implying less dissociation of 4-hydroxyl group of CUR and less inhibitory potency. Interestingly, AD (apolar desolvation energy, an energy required to remove apolar solvent from the compound, i.e., the reduction in free energy achieved by dissolving the compound) was positively associated with IC50 values. In this case, higher AD possibly led to higher LogP, thereby resulting in weaker inhibition.
CUR in plants and mammals or gut microbes can be metabolised by reductase into DHC, THC, and OHC, respectivelyCitation30. Interestingly, the reduction of CUR to metabolites significantly loses the inhibitory potency on h3β-HSD2. For example, CUR inhibited h3β-HSD2 with an IC50 value of 15.28 µM, while the IC50 value of THC was 109.0 µM and DHC and OHC had even lower potency to inhibit this enzyme. The decrease of inhibitory potency of CUR after reduction suggests that the double bonds in the bridge chain of CUR play key role in maintaining inhibitory effect.
The different inhibitory effects of curcumin analogues depend on their interactions with 3β-HSD enzyme. The hydroxyl group in the benzene ring of CUR cannot form HB with the residue of h3β-HSD2 due to the methoxyl and hydroxyl group interaction. De-methoxylation for one group as in DMC causes the hydroxyl group in the same benzene ring of CUR to form a HB with the critical residue Ser123 (). Ser123 is an important residue, since the mutagenesis of Ser123 causes the reduction of affinity of substrate and inhibitors with the enzymeCitation27. De-methoxylation for two groups as in BDMC causes the hydroxyl group in the benzene ring of CUR to form a HB with the critical residue Thr81 (). The same is true for the interaction of curcumin analogues with r3β-HSD1. The hydroxyl group in the benzene ring of CUR cannot form HB with the residue of r3β-HSD1 due to the methoxyl and hydroxyl group interaction. De-methoxylation for one group as in DMC causes the hydroxyl group in the same benzene ring of CUR to form a HB with the critical residue Thr82 (). De-methoxylation for two groups as in BDMC causes one hydroxyl group in the benzene ring of CUR to form a HB with the critical residue Ser124 and the other hydroxyl group in the benzene ring to form a HB with the Thr215 (). To understand the binding affinity of curcuminoids and the metabolite THC to 3β-HSDs, docking analysis was performed. The results of this analysis showed that the binding energy for BDMC was the lowest, followed by DMC, and then CUR and THC (). This suggests that BDMC has the strongest binding affinity to compared to DMC, CUR, and THC.
In conclusion, docking analysis has demonstrated that the binding energy of BDMC is lower than that of DMC, which in turn is lower than curcumin. This suggests that BDMC has the strongest binding affinity to target proteins compared to DMC and curcumin. These findings have important implications for the development of curcumin-based therapeutics and highlight the usefulness of computational methods in drug discovery.
The different inhibitory effects of curcumin analogues may also depend on their pharmacokinetic and bioavailability. Pharmacokinetic and bioavailability studies have shown that curcumin has low intestinal absorption and rapid clearance from the bodyCitation31. DMC and BDMC have similar absorption properties to CUR, being more water-soluble than CUR. DMC and BDMC are also metabolised in the liver, but with a lower rate than CUR and their half-lives are 2–4 hCitation32,Citation33. CUR, DMC and BDMC are excreted in the urine and faeces in the form of metabolites while DHC, THC and OHC are not metabolised and are excreted unchanged in the urine and faecesCitation1,Citation32,Citation34. That means, DMC and BDMC are the most bioavailable curcuminoids due to their good absorption, distribution, and metabolism.
Curcumin analogues have different inhibitory potencies on r3β-HSD1 and h3β-HSD2. CUR can inhibit r3β-HSD1 with Ki value of 4.2 µM, while it inhibited h3β-HSD2 with Ki value of 12.78 µM (); DMC can inhibit r3β-HSD1 with Ki value of 2.70 µM, while it inhibited h3β-HSD2 with Ki value of 3.85 µM (). However, BDMC inhibited r3β-HSD1 with Ki value of 1.71 µM, while it inhibited h3β-HSD2 with Ki value of 1.28 µM (). 3β-HSD1 contains the catalytic residue Ser125Citation35, which has been reported to play a critical role in inhibitory potency of some 3β-HSD inhibitors and the mutation of Ser125 to Thr125 can decrease the inhibitory potency of 3β-HSD1 inhibitorCitation9.
Interestingly, CUR has been found to exert different inhibitory effects of other hydroxysteroid dehydrogenase such as human 11β-HSD1, and 11β-HSD2 with IC50 values of about 10 µM for human 11β-HSD1 and about 14.5 µM for human 11β-HSD2 13. CUR inhibits human 17β-HSD3 with IC50 values of about 67.3 µMCitation14. Whether other curcumin analogues inhibit 11β-HSD and 17β-HSD3 enzymes remains further investigation.
The inhibition of curcumin analogues on h3β-HSD2 significantly decreased P4 output. Human KGN can secrete P4 under basal and cAMP-stimulated conditionsCitation15. Indeed, CUR, DMC, and BDMC can significantly lower P4 secretion as low as 10 µM, suggesting that they can pass through cell membrane to lower steroid secretion.
Cushing’s syndrome (hypercorticism) is characterised with massive cortisol secretion, which may be because h3β-HSD2 and r3β-HSD1 in adrenal glands were upregulatedCitation36,Citation37. Cushing’s syndrome is accompanied with obesity, hypertension, osteoporosis, and type II diabetesCitation38,Citation39 and women’s PCOS is also a metabolic disease, which is characterised by excessive androgen secretion (hyperandrogenism), accompanied by insulin resistance, and polycystic ovary due to increased expression of 3β-HSD2Citation40. Although trilostane is a moderate 3β-HSD2 inhibitor and was once used to treat Cushing’s syndromeCitation41. Due to different efficacy, it was withdrawn for human use in the United States in the 1990s. However, it was approved for treating Cushing’s syndrome in pets such as dogs and catsCitation11,Citation12. The identified potent h3β-HSD2 and r3β-HSD1 inhibitors of curcumin analogues such as DMC and BDMC may be potential food additives for therapeutic purposes and further preclinical studies are needed.
Conclusion
In conclusion, herein, we demonstrated that some curcumin analogues can potently inhibit h3β-HSD2 (the inhibitory potency: BDMC > DMC > CUR > THC) and r3β-HSD1 (the inhibitory potency: BDMC > DMC > CUR > DHC = THC) activities and have a clear SAR binding steroid active site with mixed or competitive mode of action. Low LogP, pKa, and AD lead to more potent inhibition, and the de-methoxylation of CUR increases its inhibitory potency and the reduction of double carbon bond decreases its inhibitory potency. As inhibitors of h3β-HSD2 and r3β-HSD1, curcumin analogues such as DMC and BDMC may be used to treat Cushing’s syndrome and PCOS after further adequate preclinical studies.
Author contributions
XQ: methodology, validation, formal analysis, and writing–original draft. LY, JL, CP, and QF: Methodology. YZ: UPLC-MS/MS assay of steroids. HL: docking analysis. RG: idea, funding acquisition, and final editing. YW: methodology, and idea and formal analysis.
Supplemental Material
Download PDF (1.2 MB)Acknowledgements
The authos thank Scientific Research Center of Wenzhou Medical University for consultation and instrument availability that supported this work.
Disclosure statement
The authors have no relevant affiliations of financial involvement with any organisation or entity with a financial interest in or financial conflict with the subject matter or materials discussed in the manuscript. This includes employment, consultancies, honoraria, stock ownership or options, expert testimony, grants or patents received or pending, or royalties.
Correction Statement
This article has been republished with minor changes. These changes do not impact the academic content of the article.
Additional information
Funding
References
- Lestari ML, Indrayanto G. Curcumin. Profiles Drug Subst Excip Relat Methodol. 2014;39:113–204.
- Unlu A, Nayir E, Dogukan Kalenderoglu M, Kirca O, Ozdogan M. Curcumin (turmeric) and cancer. J BUON. 2016;21(5):1050–1060.
- Ammon HP, Wahl MA. Pharmacology of curcuma longa. Planta Med. 1991;57(1):1–7.
- Xu S, Chen M, Chen W, Hui J, Ji J, Hu S, Zhou J, Wang Y, Liang G. Chemopreventive effect of chalcone derivative, l2h17, in colon cancer development. BMC Cancer. 2015;15:870.
- Zeng C, Zhong P, Zhao Y, Kanchana K, Zhang Y, Khan ZA, Chakrabarti S, Wu L, Wang J, Liang G. Curcumin protects hearts from ffa-induced injury by activating nrf2 and inactivating nf-kappab both in vitro and in vivo. J Mol Cell Cardiol. 2015;79:1–12.
- Shah V, Bhaliya J, Patel GM. In silico docking and adme study of deketene curcumin derivatives (dkc) as an aromatase inhibitor or antagonist to the estrogen-alpha positive receptor (eralpha(+)): Potent application of breast cancer. Struct Chem. 2022;33(2):571–600.
- Ramsewak RS, DeWitt DL, Nair MG. Cytotoxicity, antioxidant and anti-inflammatory activities of curcumins i-iii from curcuma longa. Phytomedicine. 2000;7(4):303–308.
- Mehner M, Kubelt C, Adamski V, Schmitt C, Synowitz M, Held-Feindt J. Combined treatment of at101 and demethoxycurcumin yields an enhanced anti-proliferative effect in human primary glioblastoma cells. J Cancer Res Clin Oncol. 2020;146(1):117–126.
- Simard J, Ricketts ML, Gingras S, Soucy P, Feltus FA, Melner MH. Molecular biology of the 3beta-hydroxysteroid dehydrogenase/delta5-delta4 isomerase gene family. Endocr Rev. 2005;26(4):525–582.
- Bolisetty S, Lui K, Oei J, Wojtulewicz J. A regional study of underlying congenital diseases in term neonates with necrotizing enterocolitis. Acta Paediatrica. 2007;89(10):1226–1230.
- Mellett Keith AM, Bruyette D, Stanley S. Trilostane therapy for treatment of spontaneous hyperadrenocorticism in cats: 15 cases (2004-2012). J Vet Intern Med. 2013;27(6):1471–1477.
- de Carvalho GLC, Meirelles L, da Silva CC, Neto WS, Furtado PV, Machado L, de Moura Martins FS, da Silva Mello FP, de Faria Valle S, Pöppl ÁG. Assessment of selegiline and trilostane combined therapy efficacy for canine pituitary-dependent hypercortisolism treatment: A pilot randomized clinical trial. Res Vet Sci. 2022;150:107–114.
- Hu GX, Lin H, Lian QQ, Zhou SH, Guo J, Zhou HY, Chu Y, Ge RS. Curcumin as a potent and selective inhibitor of 11beta-hydroxysteroid dehydrogenase 1: Improving lipid profiles in high-fat-diet-treated rats. PLoS One. 2013;8(3):e49976.
- Hu GX, Liang G, Chu Y, Li X, Lian QQ, Lin H, He Y, Huang Y, Hardy DO, Ge RS. Curcumin derivatives inhibit testicular 17beta-hydroxysteroid dehydrogenase 3. Bioorg Med Chem Lett. 2010;20(8):2549–2551.
- Tsutsumi R, Hiroi H, Momoeda M, Hosokawa Y, Nakazawa F, Koizumi M, Yano T, Tsutsumi O, Taketani Y. Inhibitory effects of cholesterol sulfate on progesterone production in human granulosa-like tumor cell line, kgn. Endocr J. 2008;55(3):575–581.
- Cloix L, Reverchon M, Cornuau M, Froment P, Ramé C, Costa C, Froment G, Lecomte P, Chen W, Royère D, et al. Expression and regulation of intelectin1 in human granulosa-lutein cells: Role in igf-1-induced steroidogenesis through nampt. Biol Reprod. 2014;91(2):50. 2:
- Xu RA, Mao B, Li S, Liu J, Li X, Li H, Su Y, Hu G, Lian QQ, Ge RS. Structure-activity relationships of phthalates in inhibition of human placental 3beta-hydroxysteroid dehydrogenase 1 and aromatase. Reprod Toxicol. 2016;61:151–161.
- Wang S, Zhang B, Zhai Y, Tang Y, Lou Y, Zhu Y, Wang Y, Ge RS, Li H. Structure-activity relationship analysis of perfluoroalkyl carbonic acids on human and rat placental 3beta-hydroxysteroid dehydrogenase activity. Toxicology. 2022;480(:153334.
- Zhao X, Ji M, Wen X, Chen D, Huang F, Guan X, Tian J, Xie J, Shao J, Wang J, et al. Effects of midazolam on the development of adult leydig cells from stem cells in vitro. Front Endocrinol (Lausanne)). 2021;12(:765251.
- Jumper J, Evans R, Pritzel A, Green T, Figurnov M, Ronneberger O, Tunyasuvunakool K, Bates R, Zidek A, Potapenko A, et al. Highly accurate protein structure prediction with alphafold. Nature. 2021;596(7873):583–589.
- Varadi M, Anyango S, Deshpande M, Nair S, Natassia C, Yordanova G, Yuan D, Stroe O, Wood G, Laydon A, et al. Alphafold protein structure database: Massively expanding the structural coverage of protein-sequence space with high-accuracy models. Nucleic Acids Res. 2022;50(D1):D439–D444.
- Ongtanasup T, Wanmasae S, Srisang S, Manaspon C, Net-Anong S, Eawsakul K. In silico investigation of ace2 and the main protease of sars-cov-2 with phytochemicals from myristica fragrans (houtt.) for the discovery of a novel covid-19 drug. Saudi J Biol Sci. 2022;29(9):103389.
- Ongtanasup T, Mazumder A, Dwivedi A, Eawsakul K. Homology modeling, molecular docking, molecular dynamic simulation, and drug-likeness of the modified alpha-mangostin against the beta-tubulin protein of acanthamoeba keratitis. Molecules. 2022;27(19):6338.
- Wallace AC, Laskowski RA, Thornton JM. Ligplot: A program to generate schematic diagrams of protein-ligand interactions. Protein Eng. 1995;8(2):127–134.
- Adasme MF, Linnemann KL, Bolz SN, Kaiser F, Salentin S, Haupt VJ, Schroeder M. Plip 2021: Expanding the scope of the protein-ligand interaction profiler to DNA and rna. Nucleic Acids Res. 2021;49(W1):W530–W534.
- Irwin JJ, Shoichet BK. Zinc–a free database of commercially available compounds for virtual screening. J Chem Inf Model. 2005;45(1):177–182.
- Thomas JL, Mason JI, Brandt S, Spencer BR, Jr., Norris W. Structure/function relationships responsible for the kinetic differences between human type 1 and type 2 3beta-hydroxysteroid dehydrogenase and for the catalysis of the type 1 activity. J Biol Chem. 2002;277(45):42795–42801.
- Sprous DG, Palmer RK, Swanson JT, Lawless M. Qsar in the pharmaceutical research setting: Qsar models for broad, large problems. Curr Top Med Chem. 2010;10(6):619–637.
- Sang J, Chu J, Zhao X, Quan H, Ji Z, Wang S, Tang Y, Hu Z, Li H, Li L, et al. Curcuminoids inhibit human and rat placental 3beta-hydroxysteroid dehydrogenases: Structure-activity relationship and in silico docking analysis. J Ethnopharmacol. 2023;305:116051. 2022
- Pandey A, Chaturvedi M, Mishra S, Kumar P, Somvanshi P, Chaturvedi R. Reductive metabolites of curcumin and their therapeutic effects. Heliyon. 2020;6(11):e05469.
- Anand P, Kunnumakkara AB, Newman RA, Aggarwal BB. Bioavailability of curcumin: Problems and promises. Mol Pharm. 2007;4(6):807–818.
- Mirzaei H, Shakeri A, Rashidi B, Jalili A, Banikazemi Z, Sahebkar A. Phytosomal curcumin: A review of pharmacokinetic, experimental and clinical studies. Biomed Pharmacother. 2017;85:102–112.
- Li R, Qiao X, Li Q, He R, Ye M, Xiang C, Lin X, Guo D. Metabolic and pharmacokinetic studies of curcumin, demethoxycurcumin and bisdemethoxycurcumin in mice tumor after intragastric administration of nanoparticle formulations by liquid chromatography coupled with tandem mass spectrometry. J Chromatogr B Analyt Technol Biomed Life Sci. 2011;879(26):2751–2758.
- Jude S, Amalraj A, Kunnumakkara AB, Divya C, Loffler BM, Gopi S. Development of validated methods and quantification of curcuminoids and curcumin metabolites and their pharmacokinetic study of oral administration of complete natural turmeric formulation (cureit) in human plasma via uplc/esi-q-tof-ms spectrometry. Molecules. 2018;23(10):2415.
- Thomas JL, Duax WL, Addlagatta A, Scaccia LA, Frizzell KA, Carloni SB. Serine 124 completes the tyr, lys and ser triad responsible for the catalysis of human type 1 3beta-hydroxysteroid dehydrogenase. J Mol Endocrinol. 2004;33(1):253–261.
- Onoda N, Ishikawa T, Nishio K, Tahara H, Inaba M, Wakasa K, Sumi T, Yamazaki T, Shigematsu K, Hirakawa K. Cushing’s syndrome by left adrenocortical adenoma synchronously associated with primary aldosteronism by right adrenocortical adenoma: Report of a case. Endocr J. 2009;56(3):495–502.
- Rege J, Hoxie J, Liu CJ, Cash MN, Luther JM, Gellert L, Turcu AF, Else T, Giordano TJ, Udager AM, et al. Targeted mutational analysis of cortisol-producing adenomas. J Clin Endocrinol Metab. 2022;107(2):e594–e603.
- Bostan H, Duger H, Akhanli P, Calapkulu M, Turkmenoglu TT, Erdol AK, Duru SA, Sencar ME, Kizilgul M, Ucan B, et al. Cushing’s syndrome due to adrenocorticotropic hormone-secreting metastatic neuroendocrine tumor of unknown primary origin: A case report and literature review. Hormones (Athens). 2022;21(1):147–154.
- Czapla-Iskrzycka A, Świątkowska-Stodulska R, Sworczak K. Comorbidities in mild autonomous cortisol secretion - a clinical review of literature. Exp Clin Endocrinol Diabetes. 2022;130(09):567–576.
- Allen LA, Shrikrishnapalasuriyar N, Rees DA. Long-term health outcomes in young women with polycystic ovary syndrome: A narrative review. Clin Endocrinol (Oxf)). 2022;97(2):187–198.
- Igaz P, Tombol Z, Szabo PM, Liko I, Racz K. Steroid biosynthesis inhibitors in the therapy of hypercortisolism: Theory and practice. Curr Med Chem. 2008;15(26):2734–2747.